The spectrum of osseous and articular pathology encountered about the elbow is complex and varied, reflecting its anatomic complexity, biomechanical susceptibility, and developmental maturation. Previous chapters have emphasized soft tissue contrast and multiplanar imaging capability, rather than spatial resolution, as primary strengths of MRI. It is for this reason that the primary indications for MRI in the setting of bone and cartilage injury center on radiographically occult and subtle injuries. Specific anatomic considerations, commonly encountered osseous and articular pathology as well as MRI diagnostic criteria are addressed in the following discussion.
Osseous and Articular Anatomy
The proximal aspect of the elbow is formed by the humerus, which flares distally forming two central condyles that comprise the articular surfaces of the humerus and two peripheral epicondyles that serve as attachment sites for soft tissue structures (Fig. 12.1). The articular surface of the distal humerus is divided into two components, the trochlea and the capitellum, which articulate with the ulna and radius, respectively. The articular surfaces of the trochlea and capitellum are smooth and lined with articular cartilage. Both the capitellum and trochlea are oriented with 30 degrees of anterior angulation relative to the long axis of the humerus (1).
The trochlea is a pulley-like central depression of the distal humerus. The capitellum or lateral humeral condyle is nearly spherical in configuration and allows for rotation of the radial head. The capitellum approximates a sphere superiorly but narrows inferiorly with a distinct contour change in which the anteriorly placed capitellum intersects the more posterior lateral epicondyle. The imaging appearance of the abrupt contour change at the posterolateral margin of the capitellum has been termed the “pseudodefect of the capitellum” (Fig. 12.2). The pseudodefect of the capitellum is most conspicuous on coronal images through the posterior aspect of the capitellum. In the sagittal imaging plane, the defect appears exaggerated on more lateral images (2–4). True osteochondral lesions result in flattening and deformity of the normally smooth convex anterior surface of the capitellum and are usually associated with subjacent marrow edema in the acute setting. However, increased marrow signal adjacent to a pseudodefect of the capitellum may be seen on MR arthrogram studies, which may relate to filling of the defect with contrast material (5).
Whereas the capitellum and trochlea have smooth surfaces lined by articular cartilage, the epicondyles are extra-articular structures with a rough, irregular contour. The prominent medial epicondyle is accentuated by the concave medial supracondylar ridge and serves as the origin of the anterior and posterior bands of the ulnar collateral ligament complex and, more superficially, the common flexor tendon. The less conspicuous lateral epicondyle is located just distal to the lateral supracondylar ridge and serves as the attachment site for portions of the lateral collateral ligament complex as well as the more superficial common extensor tendon.
Several bony fossae of the distal humerus are common sites for accumulation of intra-articular bodies in the elbow. The radial fossa is a shallow depression just proximal to the capitellum. Centrally, a deep excavation in the bone exists forming the coronoid fossa. This fossa is separated by a thin, fenestrated piece of bone from the posterior olecranon fossa. The olecranon fossa forms a solitary midline depression posteriorly for articulation with the olecranon.
The proximal ulna is formed by the confluence of the olecranon and coronoid processes and has several important anatomic landmarks (Fig. 12.3A–B ). The posterior olecranon process and anterior coronoid process possess articular surfaces that join to form the concave trochlear (greater sigmoid) notch. It is named for its articulation with the adjacent trochlea of the humerus. The radial (lesser sigmoid) notch is a small impression along the lateral margin of the coronoid process that articulates with the radial head. Articular hyaline cartilage covers most of the trochlear notch and the entirety of the radial notch.
Viewed en face, the ulnar articular surface has a figure-of-eight configuration with slight constriction midway between the coronoid and olecranon processes traversed by a slight bony elevation called the trochlear ridge (Fig. 12.4). The trochlear ridge lacks articular cartilage and is approximately 2 to 3 mm wide and 3 to 5 mm high (6). Because the height corresponds to that of adjacent articular cartilage, the articular surface remains smooth and congruent. In most cases, the trochlear ridge completely traverses the trochlear notch but occasionally can be incomplete (traversing only the medial or lateral aspect of the notch. Sagittal MR images may reveal a central elevation of the trochlear articular surface corresponding to the trochlear ridge. The ridge has been reported in 81% of MR studies of normal volunteers (3). This anatomic structure may simulate either a central osteophyte or an olecranon stress fracture.
Normal cortical irregularity may be seen at the peripheral margins of the trochlear ridge, termed the trochlear groove (Fig. 12.5). The contour change encountered at the peripheral margins of the trochlear ridge corresponds to the waist of the figure-of-eight–shaped articular surface of the ulna. This normal variant
can be distinguished from a true osteochondral lesion by recognition of its characteristic location.
can be distinguished from a true osteochondral lesion by recognition of its characteristic location.
The posterior surface of the olecranon process serves as the insertion of the triceps. Its marrow space is predominantly fatty with sparse trabeculation, predisposing this part of the ulna to fracture (7). The coronoid process acts as a buttress to prevent posterior translation of the ulnohumeral articulation. Indeed, fractures involving greater than 50% of the coronoid often result in elbow instability necessitating internal fixation (8). Although no discrete anatomic structure inserts on the tip of the coronoid process, the joint capsule inserts approximately 6 mm distal to this bony landmark (9). The brachialis tendon inserts approximately 11 mm distal to the coronoid tip at the ulnar tuberosity.
In general, changes in osseous contour often correspond to the attachment of soft tissue structures. In the ulna, two important soft tissue attachment sites are noted. The sublime tubercle is a small bony eminence (1) along the most medial aspect of the coronoid process where the anterior bundle of the ulnar collateral ligament inserts. This small tubercle may be avulsed in throwing athletes (10). The supinator crest (crista supinatoris) is a thin linear ridge along the lateral aspect of
the ulna representing an attachment site for the lateral ulnar collateral ligament.
the ulna representing an attachment site for the lateral ulnar collateral ligament.
The proximal radius includes the discoid-shaped radial head and the tapered radial neck (Fig. 12.6). The radial head articular surface has a central depression that accommodates the capitellum. The outer margin of the radial head articulates with the radial notch of the ulna. Hyaline cartilage of the articular surface of the radial head is contiguous with its outer margin, except for the anterolateral portion of the articular periphery, which is devoid of cartilage (7). The anterolateral portion of the articular periphery also lacks a subchondral bone plate, predisposing to fracture (1).
In the radius, an important soft tissue attachment site is the radial tuberosity located medially well below the joint line. The posterior aspect of the radial tuberosity serves as the insertion for the biceps brachii tendon, whereas the anterior portion of the tuberosity is covered by the bicipitoradial bursa.
In a minority of individuals, a bony excrescence termed the supracondylar process extends anteromedially from the distal humerus approximately 7 cm proximal to the medial epicondyle (Fig. 12.7). The supracondylar process is considered an atavistic structure typically found in amphibians, reptiles, and some mammals. This normal variant may be seen in 0.3% to 2.7% of humans and has been characterized on MRI (11). The ligament of Struthers is a fibrous band extending from the tip of the supracondylar process to the medial epicondyle, creating a fibro-osseous tunnel through which passes the median nerve and brachial artery. Compression of the median nerve may occur, causing pain and numbness of the hand with weakness of the forearm muscles.
Fractures
MRI is rarely used to assess the acute, radiographically obvious fracture. Its primary use is in the setting of radiographically occult or equivocal fractures. Posttraumatic fractures about the elbow, however, are commonly encountered. Familiarity with accepted classification systems and imaging findings that alter treatment and prognosis in the acute setting is optimal.
Distal Humeral Fracture
Fractures of the distal end of the humerus can be classified as follows: (a) supracondylar fractures; (b) transcondylar fractures; (c) intercondylar fractures; (d) fractures of the condyles (lateral and medial); (e) fractures of the articular surfaces (capitellum and trochlea); and (f) fractures of the epicondyles. This system of classification focuses on the specific anatomic site of the fracture and is considered the more traditional classification system. Muller and Schild (12) proposed a slight variation on this theme in which the distal humeral fracture was first classified as intra- or extra-articular, then classified according to anatomic site, epicondylar, supracondylar, transcondylar, bicondylar, or intercondylar (Fig. 12.8).
Distal humeral fractures can also be analyzed according to a columnar approach to the distal humerus. It has been observed that the distal humerus, when viewed from its posterior aspect, extends distally in two columns, one medial and the other lateral (13). Both columns terminate distally in the region of the trochlea. Fractures involving the distal humerus are classified
according to columnar involvement as well as by the presence of intra-articular extension of the fracture line.
according to columnar involvement as well as by the presence of intra-articular extension of the fracture line.
Regardless of the classification system used to describe distal humeral fractures, the crucial information to be rendered during imaging interpretation of such an injury should include the presence and location of intra-articular extension, the location of all fracture lines- and the degree of displacement of fracture fragments. Fractures involving only the articular surface of the distal humerus usually result in loss of motion, but not loss of stability. Fractures of the entire condyle, both articular and extra-articular portions, can lead to both restriction of motion and loss of stability. Although MRI can be used to evaluate both osseous and soft tissue components of injuries to the distal humerus, it is seldom used for the sole purpose of fracture evaluation.
Supracondylar Fractures
Supracondylar fracture of the distal humerus is the most common and problematic elbow fracture that occurs in children. This fracture represents between 55% and 80% of all children’s elbow fractures. It occurs from a fall, usually from a height greater than 2 feet (14). In 95% to 98% of such cases, the injury occurs in the outstretched arm with the elbow extended. In only 2% to 5% of patients does this injury occur in the flexed elbow.
The extension-type supracondylar fracture of the distal humerus has been classified into three types. Type IA nondisplaced fractures can be challenging to diagnose radiographically because the fracture line may be difficult to detect (Fig. 12.9). The diagnosis is based on clinical examination, which reveals tenderness, both laterally and medially over the distal humeral condyles. Although radiographs may show no cortical disruption, a posterior fat pad is often present. This injury is treated conservatively with long-arm cast placement with the elbow positioned in 90 degrees of flexion. Type IB minimally displaced/medial compression injury must be carefully evaluated by radiography to avoid underestimating the degree of deformity (Fig. 12.10). Underestimation of deformity can lead to subsequent cubitus varus alignment of the elbow. Type IIA hyperextension/posterior cortex intact injury is best diagnosed on the lateral radiograph. Fractures with extension of the humeral/capitellar relationship of more than 20 degrees will require reduction. Type IIB, displaced and angulated with osseous contact
at the fracture line, demonstrates both rotational and hyperextension deformity radiographically, but with some cortical contact remaining, usually on the posterior aspect of the humerus (Fig. 12.11). Both Types IIA and IIB will require closed reduction in the operating room. Type III completely displaced fracture generally results from an extension-type injury as previously noted. With this mechanism of injury, the distal fragment is displaced posteriorly. In the paucity of cases in which the mechanism of injury is of the flexion type, there is anterior displacement of the distal fragment (14–17).
at the fracture line, demonstrates both rotational and hyperextension deformity radiographically, but with some cortical contact remaining, usually on the posterior aspect of the humerus (Fig. 12.11). Both Types IIA and IIB will require closed reduction in the operating room. Type III completely displaced fracture generally results from an extension-type injury as previously noted. With this mechanism of injury, the distal fragment is displaced posteriorly. In the paucity of cases in which the mechanism of injury is of the flexion type, there is anterior displacement of the distal fragment (14–17).
![]() Figure 12.11 Displaced supracondylar fracture. A: Frontal and B: lateral radiographs show a completely displaced supracondylar fracture of the distal humerus. |
The complications of the supracondylar injury may be more likely to require assessment by MRI than the osseous injury itself. The incidence of nerve damage from the fracture is reported to be approximately 10% (14, 16, 18). The most commonly injured nerve in a displaced supracondylar fracture is the anterior interosseous branch of the median nerve (Fig. 12.12). After the median nerve, the nerves most likely injured include, in decreasing frequency, the radial and ulnar nerves. Ulnar neuropathies have also been reported as an iatrogenic complication from medial pin placement. In such cases, the neuropathy has been reported to resolve in 1 to 6 months after pin removal (16, 18, 19).
In approximately 10% of cases of displaced supracondylar fracture, the radial pulse will be absent at presentation. In greater than 90% of these cases, the pulse will return with closed reduction and pinning of the fracture under general anesthesia (20). In one study reviewing 13 patients with a pulseless, pink hand after closed reduction and percutaneous pinning of the supracondylar fracture, all patients were found to have vascular injuries involving the brachial artery. In this setting, segmental pressure monitoring, color flow Doppler scanning, and MR angiography were recommended for evaluation of brachial artery patency and collateral circulation around the elbow (21).
Compartment syndrome represents an all but obsolete complication of the supracondylar fracture. This is believed to be the result of the institution of immediate closed reduction and percutaneous pinning (19). In the setting of compartment syndrome, however, the MRI findings can be somewhat nonspecific. These include swelling of the affected extremity with abnormalities of signal intensity within the involved compartment, generally high signal intensity on fluid-sensitive sequences. Diagnosis is established by a combination of physical examination and intracompartmental pressure measurements. The
former reveals tense induration of the compartment in question. Intracompartmental pressure measurements should not exceed 30 mmHg. If it does, fasciotomy is indicated.
former reveals tense induration of the compartment in question. Intracompartmental pressure measurements should not exceed 30 mmHg. If it does, fasciotomy is indicated.
Transcondylar Fracture
The transcondylar fracture is considered a rare injury and has been grouped with the supracondylar fracture. Indeed, the transcondylar fracture can resemble a supracondylar fracture. The fracture line in a transcondylar fracture, however, usually extends transversely across the condyles and often has an intra-articular component. These fractures are frequently unstable and unite slowly when treated conservatively. The transcondylar fracture can be treated with percutaneous pinning. This injury, especially with intra-articular extension, can be complicated by avascular necrosis.
Intercondylar Fracture
Intercondylar fractures in adults present the most difficult challenge of all fractures encountered in the distal humerus (Figs. 12.13 and 12.14). These fractures are relatively rare and are typically observed in patients older than age 50 years (22, 23). They are produced by direct trauma to the elbow in which the ulnar articular surface is driven against the articular surface of the distal humerus (24). The classification system for this type of fracture is based on radiographic assessment and includes four types: (i) Type 1 fractures are undisplaced fractures extending between the capitellum and
trochlea; (ii) Type 2 fractures are displaced T or Y fractures with the fracture extending from the groove on the articular surface of the trochlea, proximally between the condyles, then dividing transversely or obliquely across the shaft, separating the condyles from each other without rotation; (iii) Type 3 fractures are fractures with rotational displacement of the condyles; and (iv) Type 4 fractures are those with severe comminution of the articular surface and wide separation of the condyles (25). The goal of imaging is to show the extent of bone damage and planes of the fracture lines. This is probably best achieved through the use of multidetector computed tomography.
trochlea; (ii) Type 2 fractures are displaced T or Y fractures with the fracture extending from the groove on the articular surface of the trochlea, proximally between the condyles, then dividing transversely or obliquely across the shaft, separating the condyles from each other without rotation; (iii) Type 3 fractures are fractures with rotational displacement of the condyles; and (iv) Type 4 fractures are those with severe comminution of the articular surface and wide separation of the condyles (25). The goal of imaging is to show the extent of bone damage and planes of the fracture lines. This is probably best achieved through the use of multidetector computed tomography.
Type 1 fractures are usually immobilized with a plaster splint with gradual motion instituted after healing has occurred. Type 2 and 3 fractures are treated by open reduction and internal fixation. Type 4 fractures, also referred to as “the bag of bones,” is usually treated nonoperatively. Poor results posttreatment are the result of heterotopic ossification, infection, ulnar nerve palsy, fixation failure, and nonunion. Imaging should be directed at the detection of these potential and not infrequently encountered complications in the posttreatment intercondylar fracture (24, 26).
Condylar Fractures
Condylar fractures are considered uncommon injuries that occur primarily in the setting of the skeletally immature patient. Lateral-sided injury occurs more frequently than its medial counterpart (Fig. 12.15). As a result of the involvement of both articular surface as well as the condyle, both restriction of motion and instability can result from this injury, especially if the fracture fragment is large. Fragment size and presence or absence of disruption of the lateral trochlear ridge, the structure that separates the trochlea and capitellum, comprise the elements on which the classification system for this type of fracture is based. In Type I fracture, the fragment is small and the lateral trochlear ridge is not involved. In Type II fracture, however, the fragment is larger and contains the separated condyle and extends through the lateral trochlear ridge. Type II injury allows translocation of the radius and ulna in a medial to lateral direction and can result in a fracture–dislocation (27, 28). When the condyle is displaced, open reduction and internal fixation are the best treatment.
Capitellar and Trochlear Fractures
Although trochlear fractures are extremely rare, fracture of the capitellum is one of the most common purely intra-articular fractures that occur about the elbow (Figs. 12.16 and 12.17). The mechanism of injury involves a direct force to the capitellum transmitted through the radial head resulting in a varying size shear fracture. It is important to differentiate fractures of the capitellum from fractures of the lateral condyle, because the latter often result in significant instability of the ulnohumeral joint. Fractures of the capitellar articular
surface alone, although producing an intra-articular fragment, do not result in instability.
surface alone, although producing an intra-articular fragment, do not result in instability.
![]() Figure 12.17 Capitellar fracture. Proton density-weighted fat-suppressed A: sagittal (arrow) and B: axial (arrowheads) images through the elbow show a minimally displaced fracture of the capitellum. |
Classification of fractures of the capitellum depends on the size of the articular fragment and the degree of comminution. A Type 1 fracture constitutes a large fragment of bone and articular cartilage, a Type 2 fracture is characterized by a small shell of bone and articular cartilage, and a Type 3 fracture is a comminuted fracture.
In general, the diagnosis is based on radiographic examination. Often the anteroposterior radiograph will
not define the fracture, especially if it is Type 2 and small. Small fragments residing in the superior joint may be confused with fragments of the radial head, but radial head fragments rarely migrate to the superior joint space.
not define the fracture, especially if it is Type 2 and small. Small fragments residing in the superior joint may be confused with fragments of the radial head, but radial head fragments rarely migrate to the superior joint space.
Methods of treatment include closed reduction, open reduction with and without internal fixation, excision of fragments, or insertion of a prosthesis. Closed reduction is almost never successful. Advocates of fragment excision believe that the operation is simple and offers a simple definitive procedure. Advocates of open reduction and internal fixation, however, point to the fact that with excision, a raw osseous surface is created that is prone to late valgus deformity.
Epicondylar Fractures
Isolated fractures of the epicondyles are rarely encountered in the adult and are more frequently seen in the child or adolescent. In the setting of the skeletally immature patient, these injuries fall under the category of the chondroepiphyseal injury (29–32). When this injury does occur in the adult, it happens more on the medial side than the lateral and is believed to be the result of a direct force applied to the epicondyle. Radiographs reveal a fracture fragment that is displaced in a distal or anterior direction as a result of the unopposed action of the forearm flexor muscles.
Chondroepiphyseal Injury
Pediatric elbow injuries that result from repetitive throwing-type activities are common. Several studies have documented that 20% of pitchers aged 10 to 14 years experienced elbow pain at one time or another during their careers. Many pediatric throwing injuries occur at or about secondary ossification centers. The appearance of these secondary ossification centers in boys generally occurs at the following ages: capitellum at 2 years of age, radial head at 5 years, medial epicondyle at 7 years, trochlea at 9 years, olecranon at 10 years, and finally, the lateral epicondyle at 11 years. In girls, these centers tend to appear half to 1 year earlier. Fusion occurs in the early to midteens in boys and the early teens in girls.
Similar to the adult, the cause of most throwing injuries in the adolescent is secondary to the mechanics of throwing, which results in medial tension overload, extension overload, and lateral compression. Medial-sided
injuries are the most common. The chronic forces of valgus overload produce age-dependent changes such as apophysitis in childhood and epicondylar avulsion in the more mature athlete. With repetitive valgus overload, lateral-sided injuries such as Panner’s disease and osteochondritis dissecans of the capitellum and radial head, respectively, can result. Finally, posterior patterns of injury consistent with olecranon apophysitis and posteromedial impingement can be seen (33).
injuries are the most common. The chronic forces of valgus overload produce age-dependent changes such as apophysitis in childhood and epicondylar avulsion in the more mature athlete. With repetitive valgus overload, lateral-sided injuries such as Panner’s disease and osteochondritis dissecans of the capitellum and radial head, respectively, can result. Finally, posterior patterns of injury consistent with olecranon apophysitis and posteromedial impingement can be seen (33).
Accurate diagnosis of injuries of the elbow in the pediatric population is difficult. This is the result of the large number of developing ossification centers, the variable imaging appearance of those centers as well as the cartilaginous component of the centers rendering them invisible on conventional radiography. MRI offers a diagnostic role in this setting, allowing delineation of injury types.
Medial Apophysitis
Medial apophysitis, or true “Little League elbow,” is a valgus stress fracture of the medial epicondylar physis and tends to occur in patients 10 years or younger who participate in sports with throwing activities (31). Apophyses are nonarticular and do not contribute to joint formation or longitudinal growth. They serve as the site of origin or insertion of major muscle–tendon units. In skeletally immature athletes, apophysitis develops instead of tendinosis at the site of tendon insertion (34). These athletes are still learning proper throwing mechanics and expose the elbow to abnormal and excessive forces while oftentimes throwing unlimited numbers of pitches at full effort. The presenting complaint is that of deteriorating throwing performance. Physical examination findings include swelling of the elbow, flexion contracture, and pain over the medial epicondyle, all exacerbated by throwing. As the cartilaginous medial epicondyle is ossifying, repetitive tugging of the flexor pronator mass on the medial epicondyle produces a series of traction injuries, much like Osgood-Schlatter disease at the tibial tubercle of the knee.
Radiographs comparing the injured extremity with the contralateral elbow may show separation or fragmentation of the epicondylar growth plate (Figs. 12.18 and 12.19). Occasionally accelerated growth of the medial epicondylar growth plate is noted. In a study of 343 adolescents who participated in high-performance Little League baseball, radiographs showed hypertrophy of the medial humeral epicondyle in all pitchers and catchers as well as 90% of fielders. Separation of the medial epicondyle was found in 63% of pitchers, 70% of catchers, and 50% of fielders. Fragmentation was seen in 19% of pitchers, 40% of catchers, and 15% of fielders. Only approximately 50% of the adolescents with the radiographic findings of separation or fragmentation of the medial epicondyle proved to be symptomatic. These findings raise the possibility that these skeletal lesions may be an adaptive reaction to the valgus stress of throwing and emphasize the need for close clinical and physical examination correlation when making the diagnosis of Little League elbow (35).
Medial Epicondyle Avulsion Fracture
Acute, macrotraumatic avulsion fractures of the medial epicondyle occur primarily in adolescents as the medial epicondyle begins
to fuse (Fig. 12.20). They are the result of a violent valgus force of the elbow with simultaneous forearm flexor muscle contraction. Patients report having felt a sudden “pop” or “giving way” of the elbow during the throw followed by the acute onset of pain. The physical examination reveals isolated pain and swelling over the medial epicondyle. Many patients will have locking or catching on flexion or extension with decreased range of motion of the elbow. Two types of injuries have been described based primarily on patient age and imaging findings, the Woods and Tullos (36) classification. Type I lesion occurs in children younger than 15 years of age and involves a large fragment typically made up of the entire epiphysis, which displaces and rotates. The implication in this lesion is that there has been failure of the anterior band of the ulnar collateral ligament. Type II lesion occurs in adolescents 15 years of age or older and produces a small fracture fragment. In these patients, the physis is closed and the soft tissue injury is that of the common flexor tendon rather than the ulnar collateral ligament (31, 33).
to fuse (Fig. 12.20). They are the result of a violent valgus force of the elbow with simultaneous forearm flexor muscle contraction. Patients report having felt a sudden “pop” or “giving way” of the elbow during the throw followed by the acute onset of pain. The physical examination reveals isolated pain and swelling over the medial epicondyle. Many patients will have locking or catching on flexion or extension with decreased range of motion of the elbow. Two types of injuries have been described based primarily on patient age and imaging findings, the Woods and Tullos (36) classification. Type I lesion occurs in children younger than 15 years of age and involves a large fragment typically made up of the entire epiphysis, which displaces and rotates. The implication in this lesion is that there has been failure of the anterior band of the ulnar collateral ligament. Type II lesion occurs in adolescents 15 years of age or older and produces a small fracture fragment. In these patients, the physis is closed and the soft tissue injury is that of the common flexor tendon rather than the ulnar collateral ligament (31, 33).
![]() Figure 12.20 Medial epicondyle ossification center avulsion. Frontal radiograph shows wide separation of the medial epicondyle ossification center (arrow) from the adjacent bone. |
In general, nondisplaced fractures through the medial epicondyle are treated conservatively, whereas displaced fractures are treated by open reduction and internal fixation. Disagreement exists over the amount of displacement necessary to indicate operative treatment. Many advocate operative treatment with greater than 2 mm of displacement (33, 37). Others believe that surgery is indicated in the setting of any instability demonstrated on physical examination (38). Still others have demonstrated that nonsurgical treatment in patients with greater than 5 mm of displacement produce similar long-term results to those treated surgically (39).
In the setting of an avulsed medial epicondyle, the interpretation of radiographs must be closely correlated with the age of the patient and degree of ossification of the secondary ossification centers. Comparison of the injured to asymptomatic side can be helpful in this endeavor. MRI can be instrumental in the detection of injury to the centers of ossification, the degree of injury as well as associated soft tissue injury, all findings that may alter the treatment plan offered to the patient (40).
Radial Head and Neck Fractures
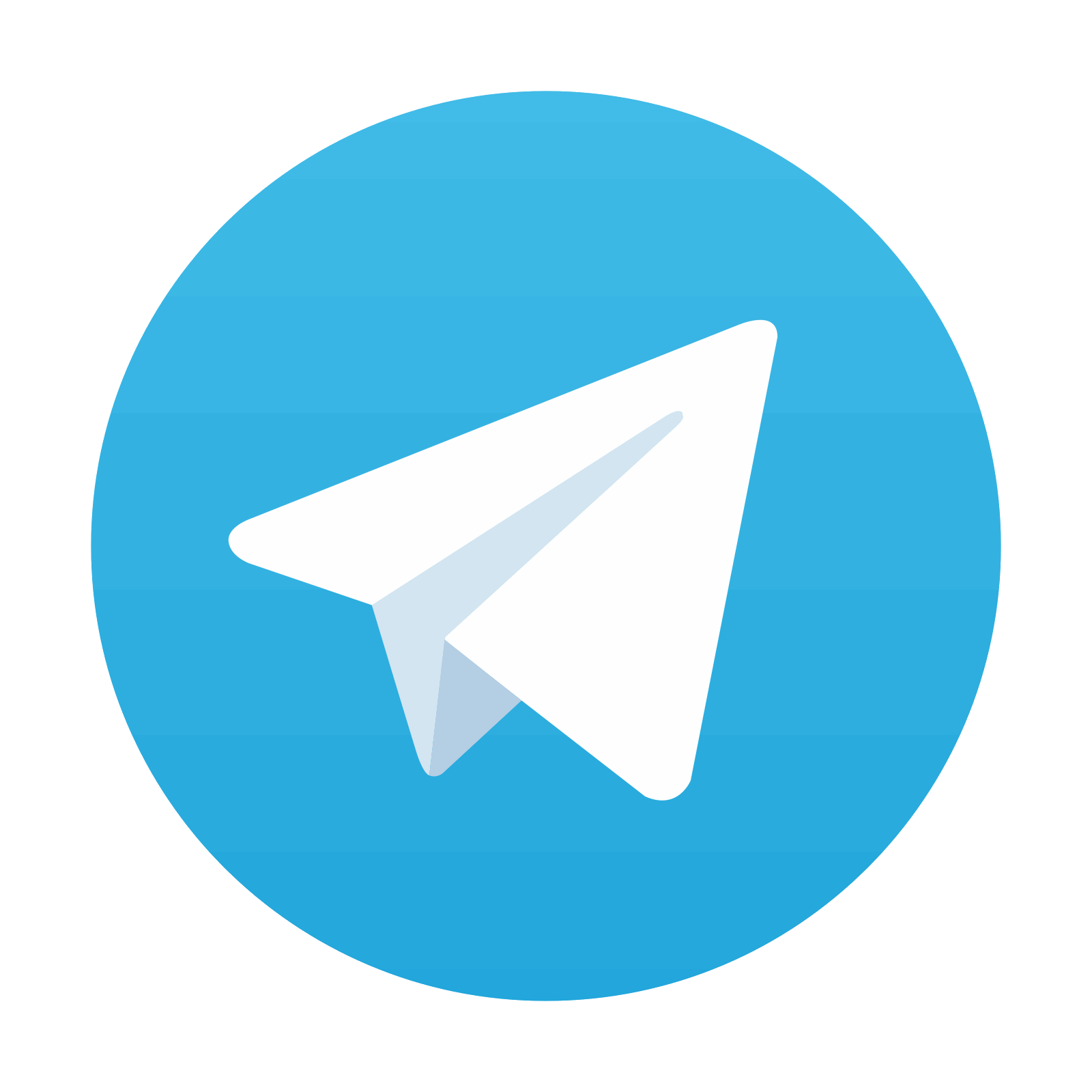
Stay updated, free articles. Join our Telegram channel

Full access? Get Clinical Tree
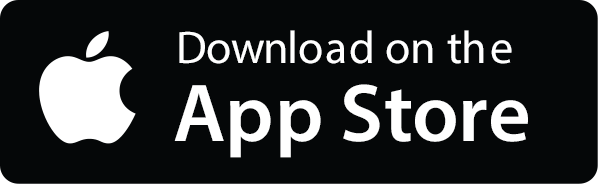
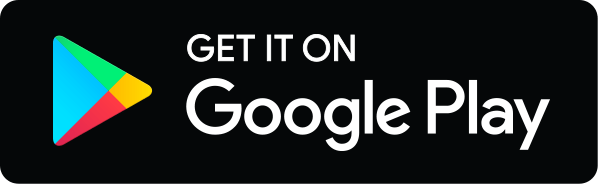