In this Chapter, we illustrate the anatomy and discuss several important pathologic conditions of the acromioclavicular joint, the rotator cuff tendons, and the rotator interval as well as the glenohumeral joint.
Acromioclavicular Joint
The acromioclavicular (AC) joint is a synovial joint that is predisposed to trauma, mechanical stress, degenerative changes, and developmental abnormalities. The articulation is reinforced by the AC ligament and the coracoclavicular (CC) ligament. The CC ligament is in turn composed of conoid and trapezoid ligaments. The conoid ligament is cone-shaped and medially located extending from the conoid tubercle on the posterior clavicle to the base of the coracoid. It is the most important ligament for supporting the AC joint against injury and superior displacement. The trapezoid ligament is quadrilateral-shaped beginning anterior and lateral to the conoid ligament on the clavicle and inserting on the coracoid process. Its main function is resisting the AC joint against compression. Together these ligaments serve as important stabilizers of the AC joint.
The AC joint is subject to a wide array of pathology, which can clinically simulate other, more commonly encountered shoulder pathology such as rotator cuff tear (1). It is therefore important to be familiar with the entire spectrum of AC joint pathology and their respective imaging findings. The next section examines several of the most commonly encountered types of AC joint pathology.
Trauma
Acromioclavicular Joint Separation
Acromioclavicular joint injuries occur in greater than 10% of shoulder injuries and are most commonly the result of a fall on the adducted shoulder (2). The Rockwood classification of AC joint injury is based on the degree of damage to the AC and CC ligaments (Fig. 7.1).
Type I AC separation is a clinical diagnosis. There is no ligamentous disruption and therefore no plain radiographic findings. However, MRI may demonstrate soft tissue edema. Type II to VI injuries may be diagnosed on routine radiographs using established normal ranges of the AC and CC distances of 3 to 7 mm and 11 to 13 mm, respectively. An anterior–posterior (AP) radiograph, which includes bilateral AC joints, is the most useful in permitting assessment of symmetry of the joints.
Rockwood type I and II injuries are treated conservatively, whereas Rockwood types IV, V, and VI injuries demand surgical intervention. Rockwood type III injury comprises 40% of all AC joint injuries, and its treatment is controversial (3). As a result of detailed depiction of soft tissue structures provided by MRI, its application may help differentiate type II and type III AC joint injuries, a distinction that carries great clinical significance (4). With type III AC injuries, MRI demonstrates disruption of CC ligaments and hemorrhage and edema within the CC interspace. Although there is currently no established role for MRI in AC joint injury, it is emerging as an important problem-solving tool for the decision between conservative and operative treatment of AC joint separation (Fig. 7.2).
Fracture of the Distal Clavicle
Its superficial location and thin midshaft make the clavicle a common site of injury, typically in persons younger than 25 who are playing contact sports (5). The most common mechanism of injury is a direct fall on the shoulder. Most commonly the fracture occurs in the middle third, comprising 80% of clavicle fractures in children and adults. Fractures of the distal third of the clavicle account for approximately 15% of clavicle fractures.
Neer (6) has classified these fractures into three types (Fig. 7.3). The most common distal clavicle fracture is the type I fracture, a stable fracture between the distal clavicle and the CC ligaments. Type II fractures are unstable with tearing of the CC ligaments and upward displacement of the medial fragment. These fractures are prone to delayed union or nonunion and require surgical fixation. Type III fractures are stable intra-articular fractures of the AC joint that are difficult to diagnose and may be confused with first-degree AC separation (Fig. 7.4). It may present as late degenerative arthrosis of the AC joint or osteolysis of the distal clavicle.
Osteoarthritis
Osteoarthritis (OA) is one of the most common and best-recognized categories of AC joint pathology. OA may be primary or secondary. In the glenohumeral and AC joints, the main causes of secondary OA are trauma and mechanical disturbances (7). Although AC joint OA is a well-recognized cause of shoulder pain, it has also been demonstrated in the asymptomatic population (8). AC joint OA has also been suggested as a cause of impingement syndrome and rotator cuff tears, because osteophytes that arise from the undersurface can impinge on the rotator cuff tendons leading to tendinopathy and tear.
Plain radiographs are useful in identifying findings of AC joint OA such as joint space narrowing, marginal osteophytes, and subchondral sclerosis and cystic changes. MRI also provides comprehensive assessment of the full extent of the degenerative process as well as its effect on the underlying rotator cuff (9). Additional
information provided by MRI includes cartilage loss, capsular hypertrophy, joint effusion, and subchondral bony edema in the acromion and distal clavicle (Fig. 7.5).
information provided by MRI includes cartilage loss, capsular hypertrophy, joint effusion, and subchondral bony edema in the acromion and distal clavicle (Fig. 7.5).
Treatment of AC joint OA is usually conservative with rest, heat, nonsteroidals, physical therapy, and intra-articular steroid injections. For those who fail the first 6 to 12 months of conservative therapy, operative treatment with distal clavicle resection (Mumford procedure) can be performed (10).
Subacromial Enthesophyte
An anterior acromial spur, or enthesophyte, extends from the anteroinferior surface of the acromion in a medial and inferior direction. These enthesophytes can be identified on AP radiographs. They arise in or adjacent to the acromial attachment of the coracoacromial ligament and are thought to represent traction osteophytes. Anterior–inferior acromial enthesophytes, although less common than AC joint osteophytes, have a higher predictive value for rotator cuff pathology. The keeled acromion is an aggressive acromial variant in which a central, longitudinal downward-sloping spur on the acromial undersurface leads to significant risk of bursal-sided and full-thickness rotator cuff tear (11). The diagnosis of a keeled acromion requires a high index of suspicion and knowledge of its imaging presentation (Fig. 7.6). These spurs should be removed during subacromial decompression.
Distal Clavicle Osteolysis
Distal clavicle osteolysis (DCO) is characterized by bony resorption of the distal clavicle that can be associated with a wide variety of causes that include posttraumatic, overuse, stress-induced, and systemic etiologies. Clinical presentation of DCO can overlap with other pathologic entities of the shoulder and includes pain, decreased strength, restricted motion, and local crepitation. In the posttraumatic variety of DCO, patients typically present after minor trauma (12). Overuse or stress-induced DCO has been reported in athletic populations such as weightlifters and vigorous racquet sport participants. Systemic disease associated with DCO includes hyperparathyroidism, rheumatoid arthritis, collagen vascular disorders, infection, multiple myeloma, and congenital disorders.
According to Levine et al. (13), the osteolytic process follows a natural course of several months period. The earliest radiographic findings include soft tissue swelling, demineralization of the distal clavicle, and loss of the subchondral cortical margin of the clavicle (Fig. 7.7). Subsequently, there is progression to focal erosions and osteolysis with loss of bony substance from the distal clavicle. Rarely there are erosions of the acromial surface as well. Eventually, the reparative phase begins and there is reconstitution of the cortex with smooth tapering of the distal clavicle. Some degree of AC joint widening is permanent.
Some have proposed that MRI is more sensitive than plain radiographs in detecting DCO because increased T2 signal in the distal third of the clavicle has been shown to precede radiographic changes (1). Other MRI findings of DCO include cortical abnormalities such as thinned or fragmented cortex, subchondral cysts, capsular hypertrophy and distention, and widening of the AC joint. These findings are not specific and correlation of clinical history with radiographic and MRI findings is important to make the correct diagnosis.
In general, DCO is a self-limited process and conservative treatment with cessation of exacerbating activities, nonsteroidals, and steroid injections is sufficient. For those patients with refractory pain, resection of the distal clavicle (Morrow procedure) can be performed with good results (14).
Os Acromiale
The acromion has four ossification centers, which appear at the age of 15 and should fuse by age 22 to 25 years. These are the preacromion, mesoacromion, meta-acromion, and basiacromion (Fig. 7.8). The ossification centers initially fuse with the scapular spine and subsequently with each other in a medial direction (15). Os acromiale is a developmental abnormality that results when one of these ossification centers fail to fuse. A large study by Sammarco (15) showed the incidence of os acromiale to be 8% with a third being bilateral. On plain radiography, the axillary view is the best view for identifying an os acromiale. On MRI, the classic finding of os acromiale is the “double joint” sign or the “pseudo-AC” joint sign (Fig. 7.9). Although the majority of patients with os acromiale are asymptomatic, patients may present with impingement-like symptoms or localized tenderness. For symptomatic patients who fail conservative management, there are a variety of surgical options, including excision of the free fragment, subacromial decompression, and open reduction with internal fixation (16).
Rotator Cuff Tendons and Rotator Interval
Hydroxyapatite Deposition Disease
Hydroxyapatite deposition disease (HADD) is a crystal-induced arthropathy in which hydroxyapatite crystals are deposited in the periarticular soft tissues such as tendons. The deposition of hydroxyapatite and related crystals can be a primary or secondary process (17). End-stage renal disease, collagen vascular disease, and tumoral calcinosis are several of the conditions that can result in secondary deposition of hydroxyapatite. The exact etiology of the primary form has been postulated to result from various conditions such as degenerative, vascular, traumatic, metabolic, and hereditary factors. The vascular etiology has received special attention because the zone of relative avascularity predisposes to hydroxyapatite deposition (18).
The shoulder is the most common site of involvement for HADD, although any joint in the body can be affected. Other commonly involved tendinous sites include the short head of the biceps tendon at the tip of the coracoid process, the long head of the biceps tendon at the superior margin of the glenoid, and the pectoralis major tendon at the proximal humeral shaft (19).
The typical patient is the middle-aged person between the fourth and sixth decades of life with equal sex distribution. The clinical presentation ranges from asymptomatic incidental finding to severe acute pain to chronic dull pain. Limited range of motion can also be present. Hydroxyapatite and related calcium phosphate crystals (such as octacalcium phosphate, carinate apatite, and calcium triphosphate crystals) are not birefringent and form amorphous clumps that stain red with alizarin red S. The definitive diagnosis requires electron microscopy, electron diffraction studies, or specialized staining, which are impractical in the everyday clinical setting. Therefore, the diagnosis of HADD is oftentimes made presumptively on the basis of radiographic findings (17).
Several staging systems exist for HADD. Uhthoff and Loehr (20) proposed that the disease can be divided into
precalcific, calcific, and postcalcific phases with the calcific phase further subdivided into formative, resting, and resorptive stages. During the precalcific phase, fibrocartilaginous transformation begins. Crystal deposition continues through the formative phase and terminates during the resting phase. The resorptive phase is marked by appearance of vascular channels and macrophage and giant cell removal of calcium deposits. Tendon healing occurs during the postcalcific phase.
precalcific, calcific, and postcalcific phases with the calcific phase further subdivided into formative, resting, and resorptive stages. During the precalcific phase, fibrocartilaginous transformation begins. Crystal deposition continues through the formative phase and terminates during the resting phase. The resorptive phase is marked by appearance of vascular channels and macrophage and giant cell removal of calcium deposits. Tendon healing occurs during the postcalcific phase.
Moseley, on the other hand, divided the natural progression of HADD into an initial silent phase, mechanical phase, and a chronic stage of adhesive periarthritis phase (21)
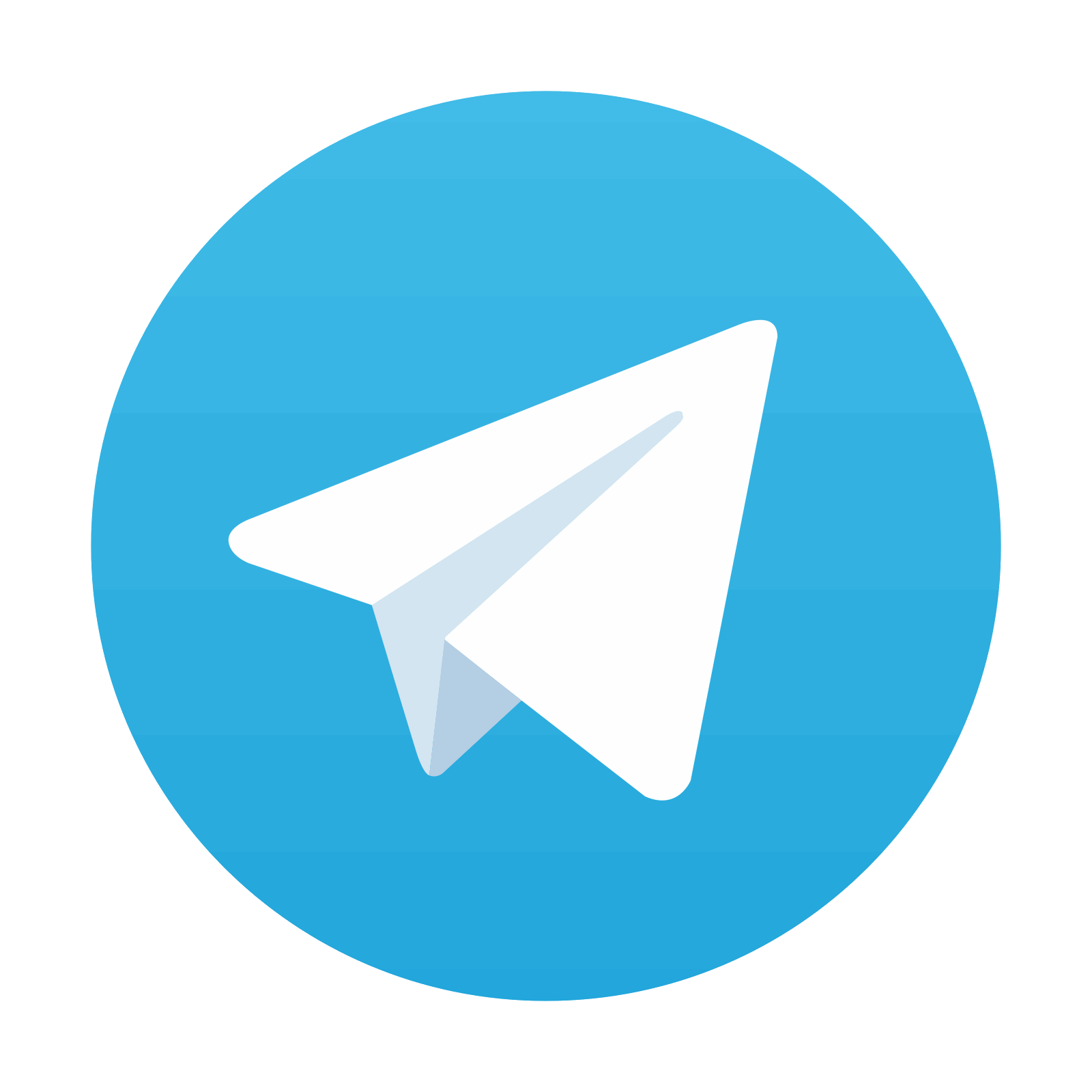
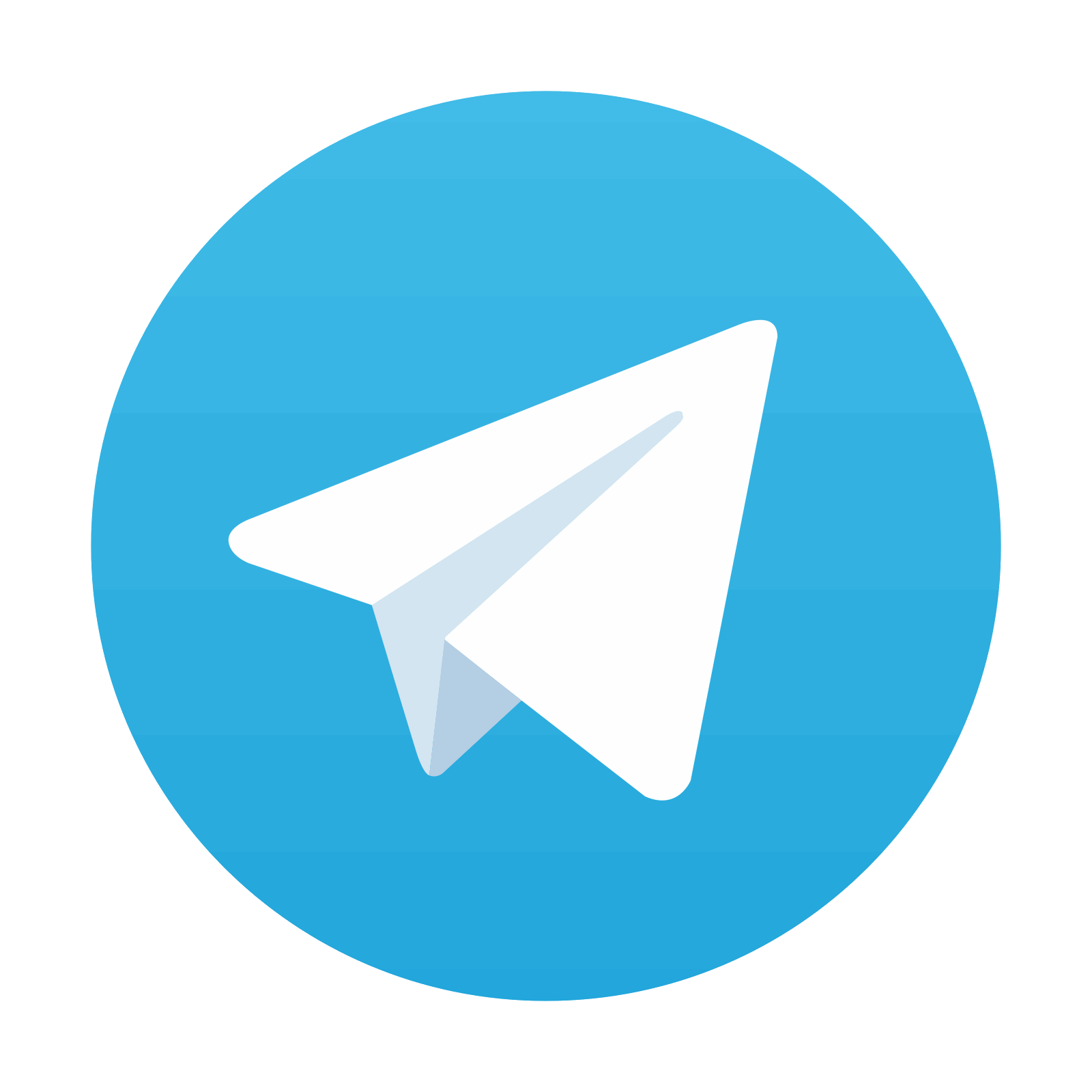
Stay updated, free articles. Join our Telegram channel

Full access? Get Clinical Tree
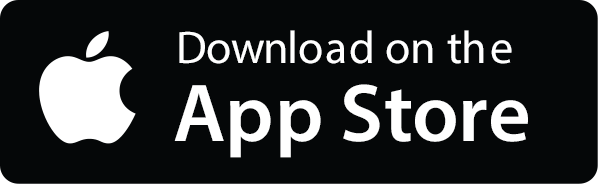
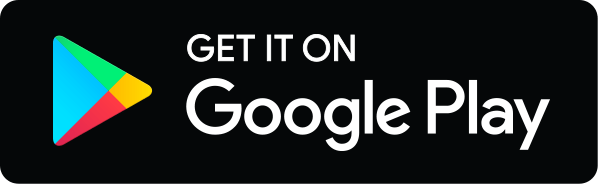
