Breast
Shannon M. MacDonald
Alphonse G. Taghian
The advantage of proton radiation is well established for tumors that lie adjacent to critical structures and require high doses of radiation.1, 2, 3, 4, 5 This past experience, along with the recognition that normal tissue sparing provided by protons will likely benefit other tumor sites, has led to the investigation of proton therapy for several other malignancies. Currently, the use of proton therapy for breast cancer is being explored, but experience to date is limited. To our knowledge, only one study regarding clinical outcomes has been published and most of the available data are in the form of dosimetric comparisons.6, 7, 8, 9 Owing to the prevalence of this malignancy and the potential for long-term survival, knowledge regarding proton radiation for breast cancer is likely to expand quickly. As proton therapy becomes more widely available, outcomes will be better and more frequently reported. We will gradually learn which subset of patients will derive the greatest benefit from proton therapy and if the degree of benefit obtained will justify the costs involved.
LATE EFFECTS OF BREAST IRRADIATION
The rationale for the use of proton radiation for the treatment of breast cancer is to decrease late toxicity by reducing the dose delivered to normal tissues. Estimating the risk of late complications for this group of patients is difficult. The latency between radiation treatment and the appearance of long-term side effects is high and therefore lengthy follow-up is necessary to gather adequate data and draw meaningful conclusions. Although many reports of late complications exist, these data are generally from studies utilizing outdated techniques. Modern radiation delivery may lower the occurrence of late side effects, but it is too early to perform adequate assessments. We must assume a risk, even with current radiation treatment, and strive to decrease the amount of radiation delivered to areas and organs known to result in late toxicities for patients with breast cancer in the past.
Earlier radiation trials reported an increase in morbidity and mortality due to cardiac disease, predominantly in patients treated for left-sided breast cancer.10, 11, 12 Some authors even suggest that increased mortality from ischemic heart disease was the reason for the absence of a survival benefit for patients treated in the earlier postmastectomy radiation trials.13,14 More recent postmastectomy studies utilizing modern techniques and fractionation schedules have demonstrated survival benefits and no increase in cardiac mortality.15,16 However, the increased use of cardiotoxic chemotherapy over the past several years adds yet another confounding factor to determining the effect of radiation therapy on cardiac outcomes. Doxorubicin (Adriamycin) and trastuzumab (Herceptin), particularly when used in combination, are known to increase the risk of cardiac disease.17,18 These agents were not included in the chemotherapeutic regimens used in the aforementioned trials. Currently, doxorubicin and Herceptin are both included in standard chemotherapeutic regimens and are often administered in combination.19 It is not known how radiation therapy in the setting of these agents will affect cardiovascular outcomes. The maximal cardiac sparing achieved through proton therapy has the potential to decrease this risk.
Symptomatic radiation pneumonitis is a subacute side effect of radiation for treatments involving lung tissue.20 This complication is reported in approximately 1% of patients treated for breast cancer. However, the risk of radiation pneumonitis has been shown to increase with treatment of the regional lymph nodes and/or concurrent chemotherapy and rates as high as 20% have been reported in patients treated with concurrent paclitaxel and radiation.21, 22, 23 Radiation pneumonitis generally resolves without treatment, but may require hospitalization or a course of steroids.
There is limited data on second malignancy following the treatment of breast cancer, but available studies do indicate an increased risk.24 Radiation-induced sarcomas have been reported with rates ranging between 0.2% to 0.48% at 10 to 15 years.25,26 Regardless of the modality used, it would be difficult to limit this risk for whole breast radiation, but avoiding irradiation of excess breast tissue through the use of protons when delivering partial breast irradiation (PBI) could decrease this risk. Increased rates of lung cancer and squamous cell carcinoma of the esophagus have also been reported.24,27, 28, 29, 30 Although the risk of a radiation-induced malignancy is relatively small, considering the large number of patients treated and the gravity of a second malignancy, it cannot be ignored.
TREATMENT OF EARLY-STAGE BREAST CANCER
Radiation therapy is an important component of breast conserving therapy for early-stage breast cancer and ductal carcinoma in situ providing markedly decreased local recurrence when compared to segmental excision alone.31, 32, 33 Conventional treatment generally consists of whole breast radiation to a dose of 50 to 50.4 Gy in fractions of 1.8 to 2.0 Gy. This is often followed by a boost dose to the tumor bed. Modern techniques allow for better avoidance of cardiac and pulmonary structures; however, adequate treatment requires that a portion of the ipsilateral lung be included in the treatment field. For patients with left-sided breast cancer, a small portion of the heart is also generally included in the field. Three-dimensional (3-D) treatment planning allows for visualization of cardiac structures leading to a greater capability to both minimize radiation to the heart and to obtain dosimetric information. However, very little data exists regarding the amount of cardiac tissue that can be safely irradiated. Investigators at Duke University have reported results from a prospective clinical trial correlating functional imaging to 3-D radiation therapy dose distributions. Preradiation and postradiation single photon emission computed tomography (SPECT) was used to obtain quantitative information regarding perfusion, wall motion, and ejection fraction.34, 35, 36 Perfusion defects were seen in 10% to 20% of patients with <5% of the left ventricle in the radiation field and in 50% to 60% of patients with >5% of the left ventricle in the field. The authors also found that wall motion abnormalities and cardiac symptoms were more common in patients with perfusion defects. Further follow-up is needed to determine if these changes will predict for clinical cardiac events, but the incidence of perfusion defects, seen even when a small volume of the left ventricle is treated, certainly support continued efforts to minimize cardiac irradiation. For these reasons, proton therapy offers a potential benefit for patients with early-stage breast cancer.
To date, no clinical outcomes have been reported for whole breast irradiation (WBI) with proton therapy; however a comparison study of dosimetry has been performed.37 Fogliata et al. analyzed the potential benefit of proton therapy for patients with highly concave breast tissue. For patients with this anatomy, it is generally more challenging to achieve homogeneous breast tissue coverage while avoiding excess lung tissue and, in certain cases, cardiac structures. This study compared five different irradiation techniques; 3-D two-field tangential photon treatment, 3-D three-field tangential photon treatment, two-field intensity-modulated radiotherapy (IMRT), three-field IMRT, and single-field proton treatment delivered with the spot scanning technique. Five patients with invasive ductal carcinoma, two with right-sided breast cancer and three with left-sided breast cancer were studied. The planned dose delivered to the breast tissue was 50 Gy at 2 Gy per fraction. Ipsilateral lung, heart (for patients with left-sided breast cancer), and contralateral breast tissue were defined and dose volume histograms generated for each planning technique. Figure 21.1 depicts isodose distributions on axial CT slices for all five techniques and clearly demonstrates superior target conformality and lung tissue sparing when proton beam radiation is used. Only the proton plan fell within the International Commission on Radiation Units and Measurements (ICRU) recommended doses for homogeneity (−5% to +7%). Conformality indices (CX) were calculated for all plans. Increasing conformality was achieved with increasing sophistication of the planning method. Protons provided the best conformity, CX 1.5, whereas two-field conventional photon planning provided the lowest degree of conformity, CX 2.5. Protons also provided the greatest sparing of normal tissues. The mean lung dose for two-field conventional planning was 13 Gy. For three-field conventional planning, two-field IMRT, and three-field IMRT, the dose ranged from 9 to 10 Gy. Proton therapy decreased this dose to 3.5 Gy (RBE). Similarly, the lowest maximum heart dose for patients with left-sided breast cancer was achieved with proton therapy. Maximum doses for two-field conventional planning and three-field conventional planning were 33.5 Gy and 24.9 Gy, respectively. For IMRT, two-field and three-field planning delivered a maximum heart dose of 33.2 Gy and 23.4 Gy, respectively. Protons reduced this dose to 19.3 Gy (RBE). Planning in each case was performed to avoid the contralateral breast. Therefore, regardless of technique, the contralateral breast did not receive any primary radiation.
Proton therapy provided superior target coverage with reduced normal tissue volume and dose using only a single field. This reduces the complexity of setup and potentially decreases setup time and error. Increased moist desquamation with single-field conventional proton therapy for PBI at the Massachusetts General Hospital/Francis H. Burr Proton Facility has led to the decision to use multiple fields for breast irradiation. However, the ability of the spot scanning
technique to achieve a reduced dose proximal to the target volume and/or the use of smaller fraction size may permit acceptable skin dose with single-field treatment.
technique to achieve a reduced dose proximal to the target volume and/or the use of smaller fraction size may permit acceptable skin dose with single-field treatment.
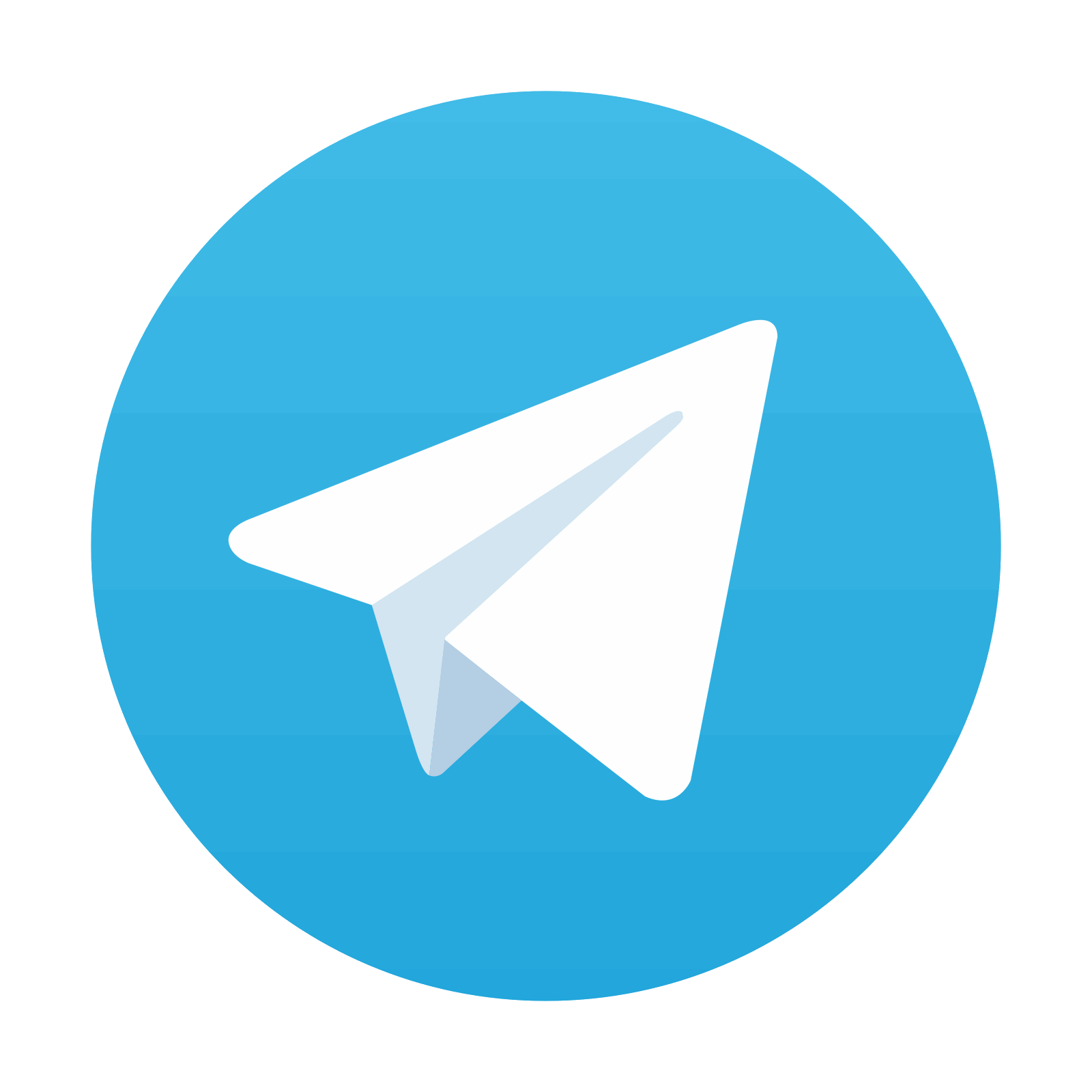
Stay updated, free articles. Join our Telegram channel

Full access? Get Clinical Tree
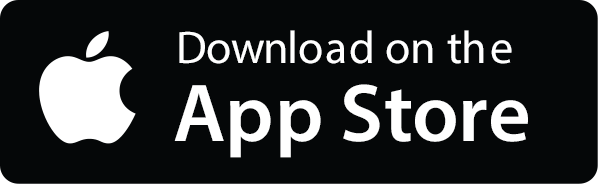
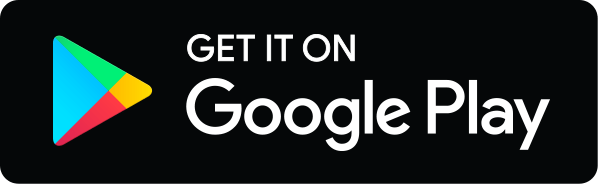