Cardiovascular Imaging
João V. Vitola
Dominique Delbeke
Introduction
Evaluation of suspected or known heart disease remains one of the most important applications of nuclear cardiology. This chapter reviews the main basic and clinical concepts involving nuclear cardiology and application of techniques to diagnose heart disease and stratify cardiac risk. In the last decades, technological developments have provided many innovative diagnostic tools for the improved identification and measurement of disease, not only in nuclear medicine but also in cardiac imaging in general. Despite all of these advances, the evaluation of coronary artery disease (CAD) involves a great deal of individual clinical judgment. Before proceeding with any diagnostic test, it is essential to obtain a thorough medical history and to perform a complete physical examination, formulate a diagnostic hypothesis, determine the pretest probability of disease, and define the best strategy of investigation for each patient. Nuclear cardiac studies and other complementary modalities are extremely helpful when well indicated and are invaluable diagnostic tools for evaluating the cardiac patient in current medical practice.
Although the delivery of optimal patient care is the ultimate goal, cost-efficient utilization of limited resources is a growing concern. The goal of avoiding unnecessary use of expensive invasive procedures has focused more attention on the use of noninvasive or less invasive diagnostic and therapeutic modalities, including nuclear imaging. Ultimately, it is the treating physician’s responsibility to rationalize the utilization of resources for optimal patient care at reasonable cost.
Atherosclerosis and Impaired Coronary Blood Flow Reserve (CBFR)—The Basis of Nuclear Cardiology
Human atherosclerosis is a dynamic process that begins early and progresses throughout life. Risk factors, smoking, hypertension (HTN), hypercholesterolemia, diabetes mellitus (DM), or a positive family history of coronary artery disease (CAD) are well known to accelerate the atherosclerotic process, which naturally affect all human beings (1). Atherosclerotic lesions may or may not affect myocardial blood flow (MBF) to a certain region of the heart, depending basically on the degree of impairment of the dilatory capacity of the coronary arteries and, importantly, the quantity and quality of collateral vessels. In terms of myocardial area at risk, these two factors are more important than the degree of vessel obstruction alone. In this context a moderately obstructive lesion of 50%, involving importantly the vessel wall, to the point of impairing its ability to dilate in response to exercise, may cause more myocardial ischemia than a 90% obstruction with a rich collateral circulation.
With progression, atherosclerotic lesions may impair coronary blood flow reserve (CBFR), initially affecting MBF during stress/exercise and at a later stage at rest. The stress tests most commonly used for evaluation of CBFR include the treadmill test (TMT) alone and rest/stress myocardial perfusion scintigraphy (MPS) using various methods for stress testing, including exercise, dipyridamole, adenosine, or dobutamine. Alternative protocols include low-level physical exercise combined with dipyridamole or adenosine.
TMT for Evaluation of CAD
A multitude of parameters have been studied and validated over the last four decades. TMT is still useful and several parameters can be measured, including total exercise time, magnitude of increase in blood pressure (BP), indicative of good cardiac function; heart rate (HR), indicative of good perfusion of the conduction system; the magnitude of ST-segment shifts; the presence of chest pain on exertion; and the cardiac rhythm abnormalities during exercise. Prognostic data can be obtained from the TMT using the Duke Treadmill Score (DTS), which can be calculated as follows for the standard Bruce protocol (2):
DTS = exercise time – (5 × ST deviation) – (4 × angina index)
Angina index can be classified as: no angina = 0, nonlimiting angina = 1, limiting angina = 2.
The scores typically range from –25 to +15. These values classify patients in the following categories: low risk, ≥+5; moderate risk, –10 to +4; and high risk, ≤-11.
In addition, the prognostic information obtained from a TMT is very important. Failure to achieve 85% of the maximum age-predicted heart rate (MPHR) and a low chronotropic response is predictive of adverse cardiovascular events (3). The average sensitivity and specificity of TMT for the diagnosis of CAD is 67% and 72% respectively, according to two meta-analyses (4,5). In addition to low sensitivity and low specificity, the TMT has additional limitations. The exercise electrocardiogram (ECG) is not interpretable in patients with left-bundle-branch block (LBBB) and pacemakers, which are usually referred to MPS for pharmacologic stress. Furthermore, some baseline ECG abnormalities will make any additional changes during exercise poorly specific (high number of false positives). This includes the changes that may occur in left ventricular hypertrophy (LVH), the effect of digitalis, the presence of preexcitation syndrome (Wolf-Parkinson-White syndrome), or prior myocardial infarction (MI). Furthermore, the sensitivity of TMT is decreased, especially when related to a limited capacity to achieve an adequate increment of myocardial oxygen consumption due either to limited exercise capacity or because of concurrent treatment with some medications such as calcium channel blockers and beta blockers.
TMT is less accurate in women than in men owing to a higher number of false-positive exercise ECG results (6). A meta-analysis determined the weighted average sensitivities and specificities of exercise ECG, exercise 201Tl, and exercise echocardiography in women (7) and demonstrated that the use of cardiac imaging increases overall accuracy. The lower specificities may be due to a digoxin-like effect of circulating estrogens, resulting in varying changes in the ST segment and
leading to a higher false-positive rate of exercise ECG stress testing in women (8).
leading to a higher false-positive rate of exercise ECG stress testing in women (8).
TABLE 3.1 Contraindications for various types of stress | ||
---|---|---|
|
In summary, the TMT is a good proven modality for diagnosis and determination of prognosis in patients suspected to have CAD, but a wide variety of limitations restricts its utility, requiring the use of alternative or complementary modalities in many patients.
Utilization of Nuclear Cardiology Procedures
Nuclear cardiology procedures allow evaluation of myocardial perfusion, viability, and function using single photon emission
computed tomography (SPECT) (see Fig. 3.A), gated SPECT, positron emission tomography (PET), and radionuclide ventriculography (RVG). These techniques continue to evolve. The indications for cardiac radionuclide imaging have been extensively reviewed and discussed in the ACC/AHA “Guidelines for the Clinical Use of Radionuclide Imaging” (13) and the American College of Cardiology Foundation/American Society of Nuclear Cardiology (ACCF/ASNC) appropriateness criteria for SPECT MPI (14).
computed tomography (SPECT) (see Fig. 3.A), gated SPECT, positron emission tomography (PET), and radionuclide ventriculography (RVG). These techniques continue to evolve. The indications for cardiac radionuclide imaging have been extensively reviewed and discussed in the ACC/AHA “Guidelines for the Clinical Use of Radionuclide Imaging” (13) and the American College of Cardiology Foundation/American Society of Nuclear Cardiology (ACCF/ASNC) appropriateness criteria for SPECT MPI (14).
![]() Figure 3.A Schematic representation of tomographic sections through the heart along the short axis, vertical long axis, and horizontal long axis. [From Vitola and Delbeke (11), by permission from Springer-Verlag.] |
MPS makes it possible to evaluate the CBFR, detect ischemia, and provide risk stratification, including the degree, location, and extent to which CAD is affecting MBF. Since its beginning in 1973 the prognostic and diagnostic value of MPS has been well established in the literature (15).
Many technological developments occurred since then, with progressively improved software and hardware for better performance and interpretation of gated SPECT studies. Excellent reviews discussing the performance of commonly used commercial software packages for SPECT analysis have recently been published (16,17,18). Normal left ventricular volumes utilizing two commonly used software packages to analyze gated SPECT studies are shown in Table 3.2.
Recent hardware developments include small dedicated cardiac gamma cameras, such as the Digirad Cardiac 3XPO (Digirad, Poway, CA), CardiArc (CardiArc, Lubbock, TX), and D-SPECT (Spectrum Dynamics; Haifa, Israel), that allow fast acquisition protocols (20).
Evaluation of ventricular function is critical in many clinical situations, including patients with CAD and valvular diseases (21). Both global and regional wall motion can be accurately evaluated with gated blood pool studies by radionuclide ventriculography (RVG). In addition, ventricular size, right and left ventricular ejection fractions (RVEF and LVEF), and regurgitation indexes can be calculated. The RVG technology and other nonperfusion applications in nuclear cardiology have been reviewed and summarized in a report by the task force of the ASNC (22). Recent technical developments of SPECT technology applied to gated blood pool studies allow more accurate evaluation of ventricular function, especially right ventricular function and diastolic dysfunction.
TABLE 3.2 Comparison between ECTb and QGS for assessment of left ventricular function from gated myocardial perfusion SPECT: LV functional parameters for subjects at low likelihood for CAD | ||||||||||||||||||||||||||||||||||||||||||||||||||||
---|---|---|---|---|---|---|---|---|---|---|---|---|---|---|---|---|---|---|---|---|---|---|---|---|---|---|---|---|---|---|---|---|---|---|---|---|---|---|---|---|---|---|---|---|---|---|---|---|---|---|---|---|
|
Multimodality imaging with integrated PET/CT and SPECT/CT systems offers the possibility for simultaneous evaluation of anatomy and function and is one of the most exciting new developments in imaging technology. Currently most PET/CT imaging systems are equipped with 16-slice CT scanners, but integrated PET/CT systems with 64-slice CT scanners are available for advanced cardiac applications and SPECT/ 64-slice multidetector computed tomography (MDCT) systems are under development. Cardiac PET/CT and SPECT/CT technology allows evaluation in one imaging setting of coronary calcium scoring (CCS), coronary artery anatomy with contrast-enhanced coronary CT angiography (CTA), rest/stress MPS, and localization of the hypoperfused regions to specific coronary arteries with the help of fusion images of the coronary tree superimposed on a 3D map of perfusion (23) (see discussion of Case 3.13). The ACC/AHA have recently published guidelines for the use of CCS (24) and cardiovascular CT imaging (25) as well as appropriateness criteria for the use of cardiovascular CT (26). The anatomic and functional information obtained with combined MPS/coronary CTA is complementary. Debate continues regarding the cost-effectiveness of the combined approach and whether the sequential approach of MPS and coronary CTA, with one test guiding the other, would be more cost-effective in specific clinical scenarios.
The choice of one of the tests discussed above to evaluate a specific patient will depend on several factors, including availability of technology, local experience with a given modality, and the pretest probability of disease, as well as patient-specific factors such as body habitus and resting ECG abnormalities. Updated imaging guidelines for nuclear cardiology procedures have been published (27), as well as training guidelines for physicians in training (28), clinical competence for physicians in practice (29), and technologist training in nuclear cardiology (30). Relevant literature on this topic and recent guidelines are discussed further on in this chapter.
Commonly Used Radiopharmaceuticals for MPS
201Tl as a Perfusion Agent. 201Tl is an analog of potassium, after the initial experiences using potassium-43 (15), which enters viable myocardial cells by passive diffusion and also by an active mechanism involving the sodium-potassium adenosine triphosphatase pump (31). Only 4% to 5% of the injected dose of 2.0 to 3.5 mCi concentrates in the myocardium, the remainder being distributed to skeletal muscle and other tissues. The physical half-life of thallium 201 (201Tl) is approximately 72 hours, but its half-life in the myocardium is significantly shorter. During its decay, 201Tl emits low-energy x-rays of approximately 70 keV. 201Tl is usually administered at peak stress and distributes in the myocardium proportionally to blood flow at stress.
One of the most clinically important characteristics of 201Tl is its redistribution over time. Redistribution is a phenomenon by which an agent dynamically crosses the cell membrane, recirculates into the coronary vessels, and becomes concentrated in the myocardium proportionally to resting blood flow. This property forms the basis of stress-redistribution imaging protocols used to diagnose CAD with 201Tl. With 201Tl, redistribution is significant, and acquisition of stress images should begin soon after the isotope is injected, preferably within 10 to 20 minutes. The longer the redistribution time, the more likely it is that 201Tl will redistribute within viable cells with an intact cell membrane—cells that were ischemic and had decreased uptake during stress. Therefore 201Tl is often used to differentiate viable tissue from scar tissue. In this regard, 201Tl has advantages over agents labeled with technetium 99m (99mTc), which do not redistribute significantly (see Case 3.18 for a detailed discussion on the use of 201Tl for viability). The pharmacokinetics of 201Tl have been investigated and discussed by Krahwinkle (32).
99mTc-Labeled Agents. Cellular uptake of cationic perfusion agents, such as 99mTc-sestamibi and tetrofosmin, is mediated by a nonspecific charge-dependent transfer of lipophilic cations across the sarcolemma but is independent of Na+/K+ channels. Therefore cellular uptake is not affected by cation channel inhibitors. Intracellularly, 99mTc-sestamibi appears to bind to the mitochondria in myocardial cells. Damaged nonviable cells do not maintain membrane potential, so 99mTc-sestamibi does not accumulate within nonviable cells.
99mTc-sestamibi rapidly clears from the blood pool with a peak activity at 1 minute postinjection. Ninety-five percent of
activity is cleared from the plasma at 5 minutes postinjection. Myocardial uptake is 1% of the injected dose following a resting injection and 1.4% following an injection during exercise. 99mTc-sestamibi has a slow clearance rate from the heart. Its effective half-life is 3 hours. After it binds to the mitochondria, there is very little redistribution, approximately 2% after 1 hour and 5% after 6 hours. Images can be acquired up to 6 hours postinjection. This allows evaluation of patients with acute chest pain syndrome (see Case 3.9) and patients with evolving myocardial infarction (MI) in whom thrombolytic therapy is planned. Although the kinetics of 99mTc-sestamibi are affected by cell metabolism and viability (33), a disadvantage of 99mTc-sestamibi is its underestimation of the extent of viable myocardium in comparison with 201Tl studies using a reinjection or 24-hour redistribution protocol. This limitation of 99mTc-sestamibi to detect viable tissue in some patients has been overcome significantly by treatment with nitrates prior to injecting the tracer (see Case 3.21 for details). Another limitation of 99mTc-sestamibi is prominent excretion into the bowel via the hepatobiliary system, which occasionally can cause difficulties in the evaluation of the inferior wall. Delayed images, especially after a meal filling the stomach, can be sufficient to improve image quality in these cases.
activity is cleared from the plasma at 5 minutes postinjection. Myocardial uptake is 1% of the injected dose following a resting injection and 1.4% following an injection during exercise. 99mTc-sestamibi has a slow clearance rate from the heart. Its effective half-life is 3 hours. After it binds to the mitochondria, there is very little redistribution, approximately 2% after 1 hour and 5% after 6 hours. Images can be acquired up to 6 hours postinjection. This allows evaluation of patients with acute chest pain syndrome (see Case 3.9) and patients with evolving myocardial infarction (MI) in whom thrombolytic therapy is planned. Although the kinetics of 99mTc-sestamibi are affected by cell metabolism and viability (33), a disadvantage of 99mTc-sestamibi is its underestimation of the extent of viable myocardium in comparison with 201Tl studies using a reinjection or 24-hour redistribution protocol. This limitation of 99mTc-sestamibi to detect viable tissue in some patients has been overcome significantly by treatment with nitrates prior to injecting the tracer (see Case 3.21 for details). Another limitation of 99mTc-sestamibi is prominent excretion into the bowel via the hepatobiliary system, which occasionally can cause difficulties in the evaluation of the inferior wall. Delayed images, especially after a meal filling the stomach, can be sufficient to improve image quality in these cases.
99mTc-tetrofosmin is used in a similar way to 99mTc-sestamibi. The myocardial uptake is approximately 1.2% of the injected dose both at rest and during exercise. Mitochondrial membrane potential plays a major role in the myocardial uptake and retention of tetrofosmin, as it does for 99mTc-sestamibi (34). Blood clearance and hepatic excretion are rapid compared with 99mTc-sestamibi. Therefore 99mTc-tetrofosmin causes less hepatic artifact, but the myocardial uptake plateaus at a slightly lower flow rate than 99mTc-sestamibi. Overall, in clinical practice these two tracers are felt to be equivalent.
The isotope 99mTc is widely used in nuclear medicine because the detectors and electronics of conventional gamma cameras are optimized for the 140-KeV photons emitted by 99mTc. In addition, 99mTc is inexpensive and readily available. The short 6-hour half-life of 99mTc permits it to be given in higher dosages than 201Tl, resulting in higher count statistics with resultant better image resolution and quality and less attenuation by intervening soft tissues. The higher count rate also allows high-quality gated images to be acquired in order to assess wall motion and ventricular function simultaneously with perfusion. Owing to the absence of significant redistribution, both supine and prone (or right lateral) imaging can be accomplished in instances where diaphragmatic attenuation artifact may pose a problem.
The energy emitted by the various isotopes may affect the choice of agent. For example, in patients with a large body habitus, it may be wise to use a higher-energy tracer, such as 99mTc-sestamibi, because there is less soft tissue attenuation than with a lower-energy-emitting tracer such as 201Tl. Owing to the lower dose used (most commonly) for the rest study, more soft tissue attenuation may be noted on the resting images; usually this presents no clinical dilemma.
The disadvantages of 99mTc-sestamibi and tetrofosmin compared to 201Tl are reduced linearity with flow, increased hepatic and splanchnic uptake, and less common lung uptake as an indicator of LV dysfunction (see Cases 3.2 and 3.5 for more details).
18F-FDG as a PET Agent. 18F-FDG is still considered as the reference standard for evaluation of myocardial viability. This is supported by a large literature, as discussed in Cases 3.19 and 3.20. However 18F-FDG is not as available as the SPECT radiopharmaceuticals (201Tl or 99mTc isonitriles). In addition, 18F-FDG myocardial imaging requires further preparation, such as glucose loading (see Case 3.20), adding 30 to 60 minutes to the procedure.
82Rb as a PET Agent. Rubidium 82 (82Rb) is a potassium analog and can be eluted from a strontium-82 generator, making it more easily available and more commonly used clinically than nitrogen-13 (13N) ammonia, which requires a cyclotron on site. Although 82Rb-PET cardiac imaging is reimbursed by third-party payers, the generator (CardioGen-82, Bracco Diagnostics, Inc., Geneva) is expensive and must be replaced monthly, limiting the use of 82Rb-PET imaging to large centers that have the volume of referrals to justify the cost. A theoretical limit in resolution of the 82Rb (compared with 13N ammonia) images occurs because the energy of the positrons emitted is higher than that of 13N and the distance traversed by 82Rb positrons is longer than that of 13N before the annihilation process. 82Rb has a short half-life of 78 seconds, allowing acquisition of rest and stress images back to back and completion of the study within 30 to 45 minutes. However, because of the short half-life, the optimal time for acquisition of the images is critical and technically demanding. Occasionally, 82Rb images may be of limited quality because of low count images or blood pool activity. These two factors together explain the lower quality of 82Rb images compared with 13N-ammonia images. Because of the short half-life, stress is limited to pharmacologic agents, which is another limitation of 82Rb.
The resting study should be performed first to reduce the impact of residual stress effects. The dose administered depends of the type of PET system [bismuth germanate oxide (BGO), lutetium oxyorthosilicate oxide (LSO), or gadolinium oxyorthosilicate (GSO) crystals] and imaging mode (the 2D imaging mode requires a higher dose than the 3D). Typically in the 2D imaging mode with a BGO PET system, ∼50 mCi of 82Rb is administered intravenously and the images are acquired starting at 70 to 90 seconds after administration for patients with a LVEF >50% and 90 to 120 seconds after administration for patients with a LVEF <50%. The emission images are usually acquired for 5 minutes and are ECG-gated.
13N-Ammonia as a PET Agent. 13N-ammonia requires a cyclotron for its production and has a half-life of 10 minutes, requiring a timely production related to the time of administration. It is rapidly cleared from the blood pool and diffuses intracellularly proportionally to MBF. Its retention depends on its metabolic incorporation into glutamine, thus cellular viability. For 13N-ammonia, the transmission images can be acquired
immediately before or after the emission images if the attenuation software can adequately correct for residual emission activity. For the resting study, gated emission images are acquired 1.5 to 3.0 minutes after 10 to 20 mCi of 13N-ammonia is administered intravenously. The images are usually acquired for 5 to 15 minutes. The stress study can be performed using the same protocol during pharmacologic stress after a 1-hour wait- ing period to allow for decay of the resting 13N-ammonia dose. 13N-ammonia also allows quantitative measurement of CBF and CBFR using compartmental modeling and kinetic analysis. The possibility of evaluating absolute MBF and CBFR using vasodilators also offers a means of investigating endothelial function and vascular smooth muscle relaxation as well as detecting early atherosclerosis. Quantitative measurement of MBF with PET is dependent on accurate attenuation correction (AC) and lack of motion of the patient during the scanning period as well as careful calibration of the imaging system. To measure the absolute myocardial perfusion rate, the arterial input function can be derived from a region of interest placed in the LV cavity. The dose of 13N-ammonia must be infused over 30 seconds and dynamic images must be acquired with varying frame durations for 4 minutes starting at the time of infusion of 13N-ammonia. Various kinetic models have been developed and validated to measure absolute MBF but are not practical in the clinical setting and are beyond the scope of this chapter.
immediately before or after the emission images if the attenuation software can adequately correct for residual emission activity. For the resting study, gated emission images are acquired 1.5 to 3.0 minutes after 10 to 20 mCi of 13N-ammonia is administered intravenously. The images are usually acquired for 5 to 15 minutes. The stress study can be performed using the same protocol during pharmacologic stress after a 1-hour wait- ing period to allow for decay of the resting 13N-ammonia dose. 13N-ammonia also allows quantitative measurement of CBF and CBFR using compartmental modeling and kinetic analysis. The possibility of evaluating absolute MBF and CBFR using vasodilators also offers a means of investigating endothelial function and vascular smooth muscle relaxation as well as detecting early atherosclerosis. Quantitative measurement of MBF with PET is dependent on accurate attenuation correction (AC) and lack of motion of the patient during the scanning period as well as careful calibration of the imaging system. To measure the absolute myocardial perfusion rate, the arterial input function can be derived from a region of interest placed in the LV cavity. The dose of 13N-ammonia must be infused over 30 seconds and dynamic images must be acquired with varying frame durations for 4 minutes starting at the time of infusion of 13N-ammonia. Various kinetic models have been developed and validated to measure absolute MBF but are not practical in the clinical setting and are beyond the scope of this chapter.
Advantages of PET versus SPECT Radiopharmaceuticals
The advantages of PET over SPECT perfusion radiopharmaceuticals include (a) better resolution and therefore better sensitivity, (b) more accurate attenuation correction (AC); (c) true stress LV EF; (d) shorter imaging protocols; (e) optimal evaluation of perfusion and viability in conjunction with 18F-FDG; and (f) a smaller radiation dose to patients compared to 201TP (23) (see discussion of Cases 3.14, 3.19 and Table 3.3).
Stress Modalities to Test Coronary Blood Flow Reserve in Nuclear Cardiology
Exercise Stress
Exercise stress testing is most commonly performed using the TMT and is discussed above, under “TMT for Evaluation of CAD.”
Pharmacologic Stress
Dipyridamole as a Stress Agent. Pharmacologic stress represents approximately 40% of the MPS studies performed in the United States (35,36). Dipyridamole inhibits the action of an enzyme called adenosine deaminase, responsible for the degradation of endogenously produced adenosine. Dipyridamole also blocks the reuptake of adenosine by cells, which again will contribute to elevation of adenosine, causing vasodilation. Dipyridamole increases MBF approximately three- to fourfold compared with baseline.
Indications for vasodilator stress are (a) inability to exercise, (b) failure to achieve 85% maximum predicted heart rate (MPHR) in the absence of typical angina or >2 mm ST-segment depression, (c) concurrent beta-blockade (or calcium antagonist) therapy (relative indication), and (d) presence of LBBB or pacemaker. Contraindications are listed in Table 3.1.
Theophylline and other xanthines (i.e., pentoxifylline) should be withheld for at least 72 hours and all caffeine and caffeinated beverages/foods for 24 to 48 hours prior to vasodilator infusion. Special attention should be given to the stopping of coffee, tea, chocolate, and soft drinks. Patients taking oral dipyridamole (as antiplatelet therapy) should stop it for 24 to 48 hours prior to vasodilator infusion. Since caffeine is cleared by the liver, special care should be taken in patients with hepatic failure, especially those evaluated prior to liver transplant. In these cases, exercise or dobutamine stress should be considered.
Dipyridamole is infused over 4 minutes at a dose of 0.56 to 0.84 mg/kg and the radiopharmaceutical is administered 7 minutes after the start of the infusion.
Special care should be taken to watch for the occurrence of severe bronchospasm and higher-degree atrioventricular (AV) block; these may occur with dipyridamole and require prompt discontinuation of the infusion and treatment.
The safety of dipyridamole has been reviewed in a series of 73,806 patients (37). Dipyridamole (at a dose of 0.56 mg/kg) causes side effects in about 50% of individuals, flushing being the most frequent in 43% of patients, chest pain (nonspecific for ischemia) in 20%, and headache in 12% (38). The half-life of dipyridamole is approximately 45 minutes. Patients receiving dipyridamole may experience symptoms after completion of the infusion when they have already left the laboratory. Administration of aminophylline prevents these occurrences in most cases. Aminophylline is administered at 1.0 to 1.5 mg/kg slow IV push until symptoms resolve, with a maximum dose of 250 mg.
The sensitivity and specificity of dipyridamole stress for the detection of CAD (>50%) have been described to be in the same range as those for exercise.
Adenosine as a Stress Agent. Adenosine promotes vasodilation by activation of vascular A2 receptors, which has an effect on cyclic AMP, reduces the influx of calcium into the intracellular space, and consequently relaxes smooth muscle cells of the coronary arteries. Adenosine increases MBF approximately four- to fivefold above baseline in myocardium supplied by normal arteries, whereas the MBF increases less in myocardium supplied by diseased arteries. The ischemic territories can be identified on MPS as areas of decreased tracer uptake. Ischemic areas can be identified on MPS by heterogenous tracer distribution owing to a differential capacity of vessels to dilate without causing true myocardial ischemia. True myocardial ischemia may occur with administration of adenosine or dipyridamole, when there is a “coronary steal phenomenon.” The steal phenomenon occurs in patients who have part of their myocardium supplied by collateral vessels owing to an occluded or critically stenotic coronary artery (39). When there is true ischemia, ST-segment shifts may be observed during the
test, as well as clinical evidence of ischemia with typical angina. Wall motion abnormalities may develop as a result of the steal phenomenon during infusion of vasodilators.
test, as well as clinical evidence of ischemia with typical angina. Wall motion abnormalities may develop as a result of the steal phenomenon during infusion of vasodilators.
Adenosine is infused IV with a pump in a volume of 50 mL of saline at a rate of 140 μg/kg per minute over 4 to 6 minutes; the rate can be decreased to 100 μg/kg per minute without a loss in sensitivity in the event of significant symptoms. BP, HR, and ECG are monitored every minute. If heart block progresses or does not resolve, infusion should be terminated. Studies comparing 4- versus 6-minute infusions of adenosine demonstrated similar sensitivity for detection of CAD, and the 4-minute infusion was better tolerated (40). The radiopharmaceutical is administered IV 3 minutes into the 6-minute adenosine infusion. BP, HR, and ECG are monitored every minute for 10 to 20 minutes or until the patient’s hemodynamic status returns to baseline.
Approximately 81% of patients undergoing adenosine infusion have some side effects, flushing being the most frequent and occurring in 37% of patients, which is followed by dyspnea in 35% and AV block in 8%. The safety of adenosine stress has been demonstrated in a large prospective study of 9,256 consecutive patients (41,42).
Adenosine has a very short half-life of less than 10 seconds. This does not necessarily mean that all side effects occurring with adenosine will resolve after cessation of infusion. Once the adenosine receptors have been activated, a cascade of events is triggered, and therefore side effects may be much more prolonged than could be suggested by the drug’s short half-life. The antidote to adenosine is aminophylline, 25 mg/min slow IV push, until symptoms resolve, with a maximum dose of 250 mg. In view of the brief half-life of adenosine, termination of the infusion is often (but not always) adequate to manage adverse events. If possible, wait 2 to 3 minutes after radiopharmaceutical injection to terminate adenosine infusion and give aminophylline. In case of very severe ischemic symptoms, administration of sublingual or intranasal nitroglycerin, 0.4 mg, may be necessary following aminophylline administration (43).
For detection of CAD in general, the sensitivity and specificity of adenosine stress have been described in the same range as exercise stress (44,45,46); however, there is some evidence that a normal SPECT study in patients undergoing vasodilator stress may not have the same negative predictive value as compared with exercise stress (47). This may be related to the higher intrinsic risk of the population usually referred for vasodilator stress. Ischemic ECG changes with normal SPECT images during vasodilator stress is uncommon but may be helpful in identifying patients at an increased risk for future cardiac events (48,49).
Dobutamine as a Stress Agent. When there is a contraindication for using adenosine or dipyridamole, dobutamine is usually utilized. Dobutamine is an inotropic agent frequently used in intensive care units to increase cardiac output, BP, and urinary output. Dobutamine has a mild effect on alpha-1 receptors, strong effect on beta-1 receptors, and moderate effect on beta-2 receptors. In the heart, dobutamine activates beta-1 receptors (50). Dobutamine causes increased HR and myocardial contractility, promoting coronary hyperemia through mechanisms similar to exercise. It is a fast-acting drug with the effect starting approximately 2 minutes into infusion. Its hemodynamic effects depend highly on the dose infused. At a low-dose of 5 to 10 μg/kg per minute it activates beta-1 and alpha-1 receptors, which increase myocardial contractility without significant effects on HR. Doses above 10 to 20 μg/kg per minute activate alpha-1 receptors, which increases both the HR and myocardial contractility, resulting in an increase of cardiac output. Doses higher than 30 μg/kg per minute increase significantly the development of ventricular arrhythmias. Dobutamine also causes a reduction of systemic vascular resistance mediated by activation of the beta-2 receptors, which may actually cause the BP to decrease in some cases. The hyperemic effect of dobutamine alone increases MBF approximately twofold above baseline, which is an effect considered lower than that obtained with dipyridamole or adenosine. However, it has been demonstrated that the administration of dobutamine combined with atropine has an hyperemic effect similar to that of dipyridamole (51). In the nuclear laboratory, dobutamine infusion has been indicated mainly to evaluate CAD in patients with chronic obstructive pulmonary disease (COPD) who are unable to exercise adequately, as is usually the case in severe COPD. Contraindications for the use of dobutamine are listed in Table 3.1.
The patient should be off beta blockers for 5 to 7 days, preferably slowly discontinuing such medication so as to prevent rebound effects. Other antianginal medications (calcium channel blockers and nitrates) should also be discontinued, as discussed earlier in this chapter. The protocol most commonly used for MPS with dobutamine starts with an infusion rate of 10 μg/kg per minute, increasing by an additional dose of 10 μg/kg per minute every 3 minutes, to a maximum dose of 40 μg/kg per minute. Atropine may be used to increase HR, starting at the second stage (52). Usually, target HR is achieved at the rate of 20 to 30 μg/kg per minute with a small dose of atropine, around 0.5 mg. The higher the dobutamine dose given, the higher the incidence of arrhythmias and side effects; the addition of atropine helps to achieve the target HR at lower dobutamine doses. The radiopharmaceutical is injected once the target HR, preferably maximum HR, is achieved and the infusion of dobutamine is continued for another minute.
Dobutamine leads to side effects in about 75% of patients, 39% having chest pain, which can be treated with sublingual nitroglycerin, and 45% having supraventricular tachycardia or ventricular ectopy (53). Ventricular tachycardia (VT) occurs in 4% to 5% of patients. Other side effects include headache (7%), dyspnea (6%), flushing (<1%), nausea, and anxiety; these are usually well tolerated and do not necessitate interruption of the infusion. Symptomatic hypotension occurs rarely and can be treated with the infusion of saline. Severe side effects of dobutamine can be reversed by beta blockers such as esmolol (0.2 mg/kg over 1 minute) or an IV bolus of metoprolol (2.5 to 5 mg). However, patients undergoing dobutamine infusion often have COPD. Beta blockers, especially at higher doses, are known to worsen COPD and should be avoided if possible. When dobutamine induces VT, amiodarone or electric cardioversion may be necessary, especially if hemodynamic instability is present. Despite the relative high incidence of
cardiac arrhythmia with dobutamine, in a study of 3,578 patients reported in the literature, there were no reports of death, MI, or ventricular fibrillation (54). The safety of dobutamine stress has also been reported in the elderly population (55) as well as in heart transplant recipients (56).
cardiac arrhythmia with dobutamine, in a study of 3,578 patients reported in the literature, there were no reports of death, MI, or ventricular fibrillation (54). The safety of dobutamine stress has also been reported in the elderly population (55) as well as in heart transplant recipients (56).
Normal myocardial perfusion studies with dobutamine are associated with a good prognosis and low cardiac event rate, less than 0.8% per year. Patients with fixed and reversible perfusion defects have a risk of 6.8% and 8.1% for major cardiac events respectively. If both fixed and reversible cardiac defects are present, the risk is 11.6% (57,58).
A2A Receptor Agonists as Stress Agents. New vasodilator stress agents, called A2A receptor agonists, have been developed. The coronary vasodilatory effect of adenosine and dipyridamole is mediated primarily by A2A receptors present in the vascular wall. Agents that selectively or preferentially stimulate A2A receptors have the advantage of improved patient safety and comfort. A number of highly potent and selective A2A-receptor agonists have now been synthesized and are in various stages of clinical development (59,60,61,62). Recently the results of a large first phase III trial evaluating regadenoson became available (63). In this trial 784 patients underwent two sets of gated SPECT MPS. The initial stress MPS study was done with adenosine and patients were subsequently randomized, in a double-blinded fashion, to either regadenoson (two-thirds of patients) or adenosine (one-third of patients). The dose of regadenoson was given as a rapid bolus (<10 seconds) while that of adenosine was 140 μg/kg per minute given as an IV infusion for 6 minutes. A summed symptom score of flushing, chest pain, and dyspnea was lower with regadenoson than with adenosine (P = 0.018). There were no serious side effects and no high-degree AV block with regadenoson. Regadenoson as a bolus provides diagnostic information on the presence and severity of reversible defects that is comparable to the information provided by a 6-minute infusion of adenosine. Regadenoson has been recently approved by the FDA in the USA.
Commonly Used Protocols for MPS
Protocols for 201Tl-SPECT MPS (Fig. 3.B)
201Tl migrates intracellularly and begins to be redistributed approximately 20 minutes after its injection. Regions of the myocardium that had decreased uptake of the radiotracer on the poststress images but which are viable will appear normal on resting redistribution images. Areas of nonviable cells resulting from previous MI will have decreased uptake of 201Tl on both the poststress and resting images. These are matched, or fixed, defects. With the stress-redistribution protocol (Fig. 3.B, top), 3 to 4 mCi of 201Tl is injected at peak stress and the images are acquired after 10 to 20 minutes. The redistribution images are then acquired after a 3- to 4-hour interval. Because this protocol overestimates the number of fixed defects by as much as 50%, the reinjection protocol has become the standard. With the reinjection protocol (Fig. 3.B, middle), a second dosage of the tracer (1 to 1.5 mCi) is given 2 to 3 hours after the stress testing is completed. After 10 to 30 minutes, a second set of images, called reinjection images, is acquired. In extremely ischemic cells, significant uptake of 201Tl into these segments may be seen only when a longer period (24 hours) of redistribution is allowed (64). Often patients evaluated for viability cannot be stressed due to clinical constraints because of symptoms of congestive heart failure (CHF). For these patients, viability can be evaluated using the rest/redistribution 201Tl protocol (Fig. 3.B, bottom). 201Tl (3 to 4 mCi) is injected at rest and images are acquired 4 and sometimes 24 hours later (see Case 3.18 for further details on 201Tl imaging for viability).
![]() Figure 3.B Protocols for 201Tl-SPECT MPS. [From Vitola and Delbeke (11), by permission from Springer-Verlag.] |
Protocols for 99mTc-Sestamibi and Tetrofosmin SPECT MPS (Figure 3.C)
Two separate administrations of radiopharmaceutical are necessary for the stress-induced and resting images because they are retained in the myocardium without redistribution over time. Ideally, rest and stress studies are performed on two different days (Figure 3.C, top) with a 20- to 30-mCi dose for each study, gating both image acquisitions. However,
in some cases this is not practical, especially for patients needing to travel long distances. A 1-day protocol (Fig. 3.C, middle and bottom) can then be used. A lower dose is administered for the first study (typically 8 to 10 mCi), which can be started by stress or rest as demonstrated. The second study can be performed 1 hour later with a threefold higher dose (25 to 30 mCi). Images are typically acquired 30 to 60 minutes after administration of the radiopharmaceutical at rest and 15 to 30 minutes after physical exercise. After pharmacologic stress using vasodilators, there is also vasodilation of the splanchnic circulation, increasing liver uptake, therefore a longer waiting period of 45 to 90 minutes before acquisition of the images reduces interference of hepatic uptake with the inferior wall of the myocardium. Longer intervals may be necessary in individual cases.
in some cases this is not practical, especially for patients needing to travel long distances. A 1-day protocol (Fig. 3.C, middle and bottom) can then be used. A lower dose is administered for the first study (typically 8 to 10 mCi), which can be started by stress or rest as demonstrated. The second study can be performed 1 hour later with a threefold higher dose (25 to 30 mCi). Images are typically acquired 30 to 60 minutes after administration of the radiopharmaceutical at rest and 15 to 30 minutes after physical exercise. After pharmacologic stress using vasodilators, there is also vasodilation of the splanchnic circulation, increasing liver uptake, therefore a longer waiting period of 45 to 90 minutes before acquisition of the images reduces interference of hepatic uptake with the inferior wall of the myocardium. Longer intervals may be necessary in individual cases.
![]() Figure 3.C Protocols for 99mTc-sestamibi and Tetrofosmin SPECT MPS. [From Vitola and Delbeke (11), by permission from Springer-Verlag.] |
In the rest/stress protocol, there is no contamination on the second study from the previous 99mTc-sestamibi injection. Despite some limitations of same-day versus separate-day protocols, the rest/stress same-day 99mTc-sestamibi protocol provides high diagnostic accuracy for the detection of CAD (65). If stress imaging is performed first and is normal, rest imaging may not be necessary—an advantage of the stress/rest sequence. If a 2-day rest/stress protocol is used with the higher dose (25 to 30 mCi) of 99mTc-sestamibi, performance of the resting study may be unnecessary if the stress images are normal. However, attenuation artifacts, which are frequent in nuclear medicine and vary from patient to patient, may warrant the acquisition of both rest and stress studies to facilitate accurate interpretation. When stress images are acquired with attenuation correction (AC), rest images may become unnecessary.
Dual-Isotope Rest 201Tl/Stress 99mTc-Sestamibi or Tetrofosmin SPECT MPS Protocol (Fig. 3.D)
Using the dual-isotope protocol, the resting study is acquired 10 to 20 minutes following administration of 3 mCi 201Tl, after which the patient can be immediately stressed; the 99mTc-labeled agent (25 to 30 mCi) is administered at peak stress. An additional rest 201Tl image can be obtained at 24 hours if necessary to increase sensitivity to detect viable tissue/hibernating myocardium (see Case 3.18 for further details). The dual-isotope protocol is used less commonly because of the higher radiation dose due to 201Tl compared with rest/stress 99mTc-radiopharmaceutical protocols (see Table 3.3).
Protocols Using Vasodilator Stress and Low-Level Exercise Combined
Pharmacologic alternatives must be considered in patients with poor exercise tolerance and/or those who are unable to reach at least 85% of their MPHR. Protocols combining vasodilators (dipyridamole and adenosine) with exercise have been established in the past several years (66,67,68). The vasodilator effect of dipyridamole or adenosine has been described to be greater than that of exercise alone except in cases of nonresponders. There are some data suggesting that exercise can contribute to the identification of these individuals, but this is under investigation (69,70).
Compared with exercise, which is the most physiologic stimulus, dipyridamole or adenosine stimuli alone have several limitations, including low sensitivity of the ECG for ischemia and frequent side effects (38). These vasodilators induce dilatation of the splanchnic vasculature, resulting in a higher concentration of radiopharmaceutical in the liver and intestinal tract (71). Exercise promotes a redistribution of blood flow to the skeletal musculature and away from intra-abdominal organs such as the liver (72). These effects result in a higher ratio of heart-to-liver activity on images obtained after exercise compared with those obtained after vasodilator infusion alone (73). Some studies have shown that the addition of low-workload exercise helps to decrease dipyridamole’s side effects (74,75,76,77). Other studies, using adenosine, have shown similar effects, reducing side effects (78) and arrhythmias (79) and decreasing 99mTc-sestamibi concentration in the liver (79), resulting in better image quality. The images can also be acquired earlier, after administration of the radiopharmaceutical, in patients undergoing a combined exercise/vasodilator protocol compared with vasodilator alone (66,80).
![]() Figure 3.D Dual-isotope rest 201Tl/stress 99mTc-sestamibi or tetrofosmin SPECT MPS protocol. [From Vitola and Delbeke (11), by permission from Springer-Verlag.] |
The indication for this combined protocol include patients unable to exercise to 85% MPHR but able at least to walk and those with concurrent use of medications that may limit HR increase. It is important to note that patients with LBBB or pacemakers should undergo vasodilator stress alone to reduce the false-positive rate associated with exercise (81).
Most patients for the combined protocol are exercised at low workload as per their abilities, such as the first and second stage of a standard or modified Bruce. Some other lower-workload protocols, such as Kattus or Naughton, may be applied. If the patient tolerates it, the workload is increased, but if he or she is unable to exercise at a higher level, then exercise should be maintained at a lower level (limited to stage 1 or 2 of the protocols described). With exercise plus dipyridamole, the maximum action of the drug occurs between 6 and 9 minutes into the infusion; therefore the infusion may start before the exercise (approximately at 3 minutes into the infusion), with the tracer being injected at 6 to 9 minutes from the start of infusion (66). With adenosine, the infusion is started at the same time as the exercise protocol and the radiopharmaceutical is administered at 2 to 3 minutes into the drug infusion while the patient continues to exercise to maximum tolerance (68).
Significant ST-segment changes during low workload (the first 3 minutes of a Bruce protocol) are signs of severe disease and are associated with a higher rate of adverse cardiac events (82). Importantly, the magnitude of ST-segment depression at this low workload can stratify patients into different risk categories, with approximately 80% of patients with 3 mm of ST-segment depression having cardiac events within 6 years.
The impact of adjunctive adenosine infusion during exercise MPS has been studied as part of a multicenter trial where 35 patients were enrolled prospectively and underwent both exercise MPS and exercise MPS with a 4-minute adenosine infusion on a separate day (BEAST trial) (83). The summed stress scores (SSS) and summed difference scores (SDS) were greater in the exercise-plus-adenosine group than the exercise-only group. The study concludes that the combined protocol resulted in a greater amount of myocardial ischemia detected on the SPECT images while allowing for the assessment of functional capacity.
Attenuation Correction
Attenuation correction is recommended when available and improves normalcy rate. In 2001, the ASNC and Society of Nuclear Medicine (SNM) issued a joint position statement after reviewing the literature, using the current transmission maps acquired with radioisotope sources (84). The addition of AC improves specificity from an average of 64% to 81%, and normalcy rates from an average of 80% to 89% without a loss of sensitivity. Attenuation correction can also be performed using CT attenuation maps from integrated imaging systems (see Cases 3.14 and 3.22)
Radiation Dose Considerations
With the technical development of coronary computed tomography angiography (CTA) and hybrid nuclear/CTA procedures, radiation doses from cardiovascular imaging procedures have become a concern to both patients and physicians. In 1998, the ACC has published a consensus document discussing radiation safety in the practice of cardiology (85). The issues regarding radiation dosage from various cardiac nuclear and radiographic procedures have recently been summarized (86). The dual-isotope protocol and other protocols using 201Tl are utilized less commonly nowadays because of the higher radiation dose of 201Tl compared with rest/stress 99mTc-radiopharmaceutical protocols (Table 3.3).
Choice of Diagnostic Tests Based on Prevalence of Disease
The appropriateness of noninvasive testing must be considered in light of Bayes’ theorem, which expresses the posttest likelihood of disease as a function of sensitivity and specificity of the test and the prevalence (or pretest probability) of disease in the population being tested (Fig. 3.E). Algorithms used to evaluate patients for myocardial ischemia depend on the pretest probability of the presence of CAD. Patients can be classified into low, intermediate, or high pretest probability. Placing a patient into one or another category depends greatly on the physician’s judgment, taking into account the patient’s age, gender, coronary risk factors, and symptoms.
TABLE 3.3 Radiation dosage from cardiovascular procedures | ||||||||||||||||||||||||||||
---|---|---|---|---|---|---|---|---|---|---|---|---|---|---|---|---|---|---|---|---|---|---|---|---|---|---|---|---|
|
The other important parameter is the absence or presence of symptoms. For asymptomatic patients, risk stratification is commonly evaluated using the Framingham Risk Score (FRS), which is an estimate of 10-year risk for hard coronary events (MI or coronary death). The FRS is calculated based on age, gender, systolic blood pressure, total and HDL cholesterol levels in plasma, and the presence of risk factors including diabetes and smoking (87). Asymptomatic patients with an intermediate FRS (10-year risk of hard coronary events between 10% and 20%) should be considered for coronary calcium scoring (CCS) to further risk-stratify them as recommended by the ACC/AHA (24) and ASNC (88).
After a review of the literature, including 28,948 patients, Diamond and Forrester (89) have reported the prevalence of CAD based on the patient’s age, gender, and symptoms. With respect to symptoms, the patients were classified as asymptomatic, nonanginal, atypical, and typical chest pain according to three characteristics of chest pain (substernal location, induction by exercise, relieved by nitroglycerin). When the three characteristics were present, the patients were classified as having typical chest pain. Their results demonstrated that the prevalence of CAD in the asymptomatic population ranges from 0% to 20%. As another example, the pretest likelihood of disease for men 30 to 60 years of age is much higher than that for women of the same age, of course secondary to the influence of gender on CAD development. If symptoms of typical chest pain are present as opposed to those of atypical chest pain, the pretest probability of CAD is markedly increased. Factors such as smoking, family history of CAD, sedentary lifestyle, or presence of hypercholesterolemia, HTN, or DM are known to contribute to the risk of CAD and increase the pretest probability even further. The aggressiveness of further evaluation depends on the pretest probability.
Once the pretest probability of disease is estimated, an algorithm can be created to evaluate patients with chest pain. The posttest probability for CAD depends on the sensitivity and specificity of the test being performed (90).
Patients with Low Pretest Probability of CAD
According to Diamond and Forrester, a positive result obtained with MPS study or another method of evaluating myocardial ischemia (e.g., TMT and stress echocardiography) is more likely to be a false positive if the patient has a low pretest probability for CAD. This suggests that a positive MPS study in asymptomatic patients with low risk factors (low pretest probability) does not necessarily establish the presence of CAD, while a negative test effectively excludes the presence of CAD. Several studies do support that theoretical conclusion (91). Therefore the approach to the evaluation of patients with a low-pretest probability is to begin with a resting ECG and a TMT. If there are abnormalities on the resting ECG, making it uninterpretable during a TMT, other options including imaging should be considered. MPS is one of the most sensitive and specific methods for the noninvasive evaluation of myocardial ischemia, considering that MPS evaluates physiology while coronary angiography primarily evaluates anatomy. Pooled data from 19 studies (92) have shown a sensitivity of 83% to 98% (with a mean of 92%) and a specificity of 53% to 100% (with a mean of 77%) for detecting ischemia.
Coronary CTA may become the first test of choice in these low-probability patients because of its high negative predictive value (NPV) excluding CAD. However, when coronary CTA is abnormal, the functional impact on perfusion should be further evaluated with MPS (93).
Patients with Intermediate Probability for CAD
MPS has optimal discriminative value in the patient population with an intermediate pretest probability of CAD in the range of 40% to 70% (Fig. 3.E). This population includes (a) patients with nonanginal chest pain and a positive or nondiagnostic
exercise ECG; (b) asymptomatic patients with significant risk factors, abnormal resting ECG, or positive exercise ECG; (c) patients with atypical chest pain; and (d) patients with typical chest pain and a negative exercise ECG. For patients in this category, MPS may be more helpful as an initial evaluation than it would be in those with a low or high pretest probability. A positive result is more likely to be a true-positive finding in a patient with intermediate probability than in a patient with low probability. A negative result in a patient with intermediate probability is more likely to be a true-negative finding than in a patient with high pretest probability. This hypothesis was also supported by several studies (94,95).
exercise ECG; (b) asymptomatic patients with significant risk factors, abnormal resting ECG, or positive exercise ECG; (c) patients with atypical chest pain; and (d) patients with typical chest pain and a negative exercise ECG. For patients in this category, MPS may be more helpful as an initial evaluation than it would be in those with a low or high pretest probability. A positive result is more likely to be a true-positive finding in a patient with intermediate probability than in a patient with low probability. A negative result in a patient with intermediate probability is more likely to be a true-negative finding than in a patient with high pretest probability. This hypothesis was also supported by several studies (94,95).
![]() Figure 3.E Probability of CAD and posttest probability of CAD for abnormal and normal results of quantitative 201Tl stress imaging (sensitivity 90%, specificity 95%). The curve describes the difference between posttest probability of a normal and an abnormal test result, indicating the range of disease prevalence for which 201Tl stress imaging discriminates most effectively between the presence or absence of disease. 201Tl stress imaging is most useful when the pretest prevalence of CAD is 40% to 70%. For example, in a patient with a pretest probability of disease of 60%, a positive 201Tl stress test increases the probability of CAD to 90%, while a negative test decreases it to about 15%. ▪ = abnormal; ▲ = posttest probability difference; • = normal. [Reproduced with permission from Hamilton et al. (90).] |
Coronary CTA may also be considered in this group of patients (25) and may show nonobstructive atherosclerosis when MPS is normal. The presence of atherosclerosis may lead to more aggressive medical therapy and require greater patient compliance with treatment.
Patients with a High Pretest Probability of CAD
Patients with a high pretest probability should almost always be investigated, at least once, more aggressively and invasively. According to Bayes’ theorem, a negative MPS in a patient with a high pretest probability for CAD, for example, a symptomatic patient, is more likely to have a false-negative than a true-negative result. Coronary angiography is often used as the initial evaluation in these patients. The advantage of performing nuclear before proceeding to invasive would be to identify the culprit lesion responsible for the patient’s symptoms and to document a baseline status useful for therapeutic follow-up.
In some cases, the angiographic findings are already known and the question is whether or not the lesions found compromise MBF and to what extent. Information obtained with MPS allows accurate estimation of the prognosis. It is essential to remember that CAD is not synonymous with myocardial ischemia and that anatomic findings do not always provide information about the physiologic significance of coronary lesions.
This introduction has reviewed some of the basic concepts of how nuclear cardiology, through coronary reserve testing, is able to detect and evaluate CAD, as well as stressing the importance of understanding the patient’s pretest probability of CAD before proceeding to any given test and the implications this has for the interpretation of a given result. The remainder of this chapter reviews important topics for the everyday practice of nuclear cardiology using real-life cases as a basis for discussion.
Case 3.1
History: A 69-year-old male with a 2-year history of progressive typical exertional angina presented for preoperative evaluation prior to excision of a parotid gland tumor. Cardiac risk factors included HTN, DM, tobacco use, age, and gender. The resting ECG was normal. An exercise 201Tl SPECT (Fig. 3.1) was performed. He exercised for 8.5 minutes without chest pain using a standard Bruce protocol. He achieved 78% of his MPHR. His stress ECG was positive for myocardial ischemia.
Findings: There is a severe reversible defect involving the mid- and distal anteroseptal region, the entire apex, and the inferior wall.
Discussion: A reversible defect indicates myocardial ischemia and is defined as a regional area of relative decreased 201Tl uptake (or other perfusion tracers) with stress that resolves, improves, or is normal at rest. Because of this patient’s typical anginal symptoms, age, and numerous cardiac risk factors, he was found to have a high pretest probability of myocardial ischemia and had been referred initially for coronary angiography, which revealed a chronic occlusion of the mid-left anterior descending coronary artery (LAD) with distal filling via collaterals from the right coronary artery (RCA); a 70% lesion was noted at the mid-RCA.
Following the coronary angiography, an exercise 201Tl SPECT study was ordered to determine the extent of myocardial ischemia and viability to the vascular territories found abnormal on angiography. MPS demonstrated significant ischemia, and the patient was referred for treatment with percutaneous coronary angioplasty (PTCA) of the mid-RCA lesion. MPS may often be useful, as in this patient, in determining the physiologic importance of known sites of CAD.
201Tl as a perfusion agent and the different 201Tl protocols are discussed in the introduction to this chapter.
Diagnosis: Myocardial ischemia due to two-vessel CAD detected by 201Tl imaging.
Case 3.2
History: A 73-year-old woman presented with a 1-month history of recurrent nausea and diaphoresis. She denied chest pain and had no history of CAD. Cardiac risk factors included age, HTN, hypercholesterolemia, and peripheral vascular disease (PVD). The resting ECG was normal. Using a 1-day protocol, an adenosine 99mTc-tetrofosmin SPECT (Fig. 3.2) was performed. No chest pain occurred, and the stress ECG was negative for myocardial ischemia.
Findings: Distribution of the radiopharmaceutical is homogeneous throughout the myocardium on both sets of images, compatible with normal perfusion at both stress and rest. The polar plots reveal similar normal perfusion.
Discussion: The normal MPS is indicative of an excellent prognosis, even in the presence of an abnormal ECG or abnormal coronary angiography (96). A study of 652 patients who had a normal stress SPECT study alone and were followed for 2 years demonstrated that the overall cardiac event rate in these patients was <1% (97).
The overall sensitivity, specificity, and accuracy of 99mTc-tetrofosmin or 99mTc-sestamibi SPECT for the detection of myocardial ischemia is similar to that of 201Tl imaging. The normal perfusion scan is indicative of an excellent prognosis, even in the presence of an abnormal coronary angiogram. The shorter half-life of 99mTc-labeled agents compared with 201Tl allows a higher dosage to be administered. This, combined with the higher energy of 99mTc, results in a higher count density than is possible using 201Tl. Image quality is improved, and attenuation artifacts are less frequent and less severe. The higher count rate also allows high-quality gated images to be acquired in order to assess wall motion and ventricular function simultaneously with perfusion. Owing to the absence of significant redistribution, both supine and prone (or right lateral) imaging can be accomplished in instances where diaphragmatic attenuation artifact may pose a problem. A major disadvantage of 99mTc-labeled agents is that their use underestimates the extent of viable myocardium in comparison with 201Tl imaging using a reinjection or 24-hour redistribution protocol, which can be overcome by nitrate administration (see Case 3.21 regarding the use of nitrate 99mTc-sestamibi for viability).
Diagnosis: Normal 99mTc-tetrofosmin rest/stress study.
Case 3.3
History: A 70-year-old male with no prior history of CAD presented with a 2-week history of atypical chest pain. Cardiac risk factors included age, gender, HTN, and hypercholesterolemia. The resting ECG was normal. An exercise 99mTc-sestamibi SPECT (Fig. 3.3 A) was performed. The patient exercised for 9.5 minutes on a standard Bruce protocol without chest pain. Target HR was achieved. The stress ECG was suggestive of myocardial ischemia.
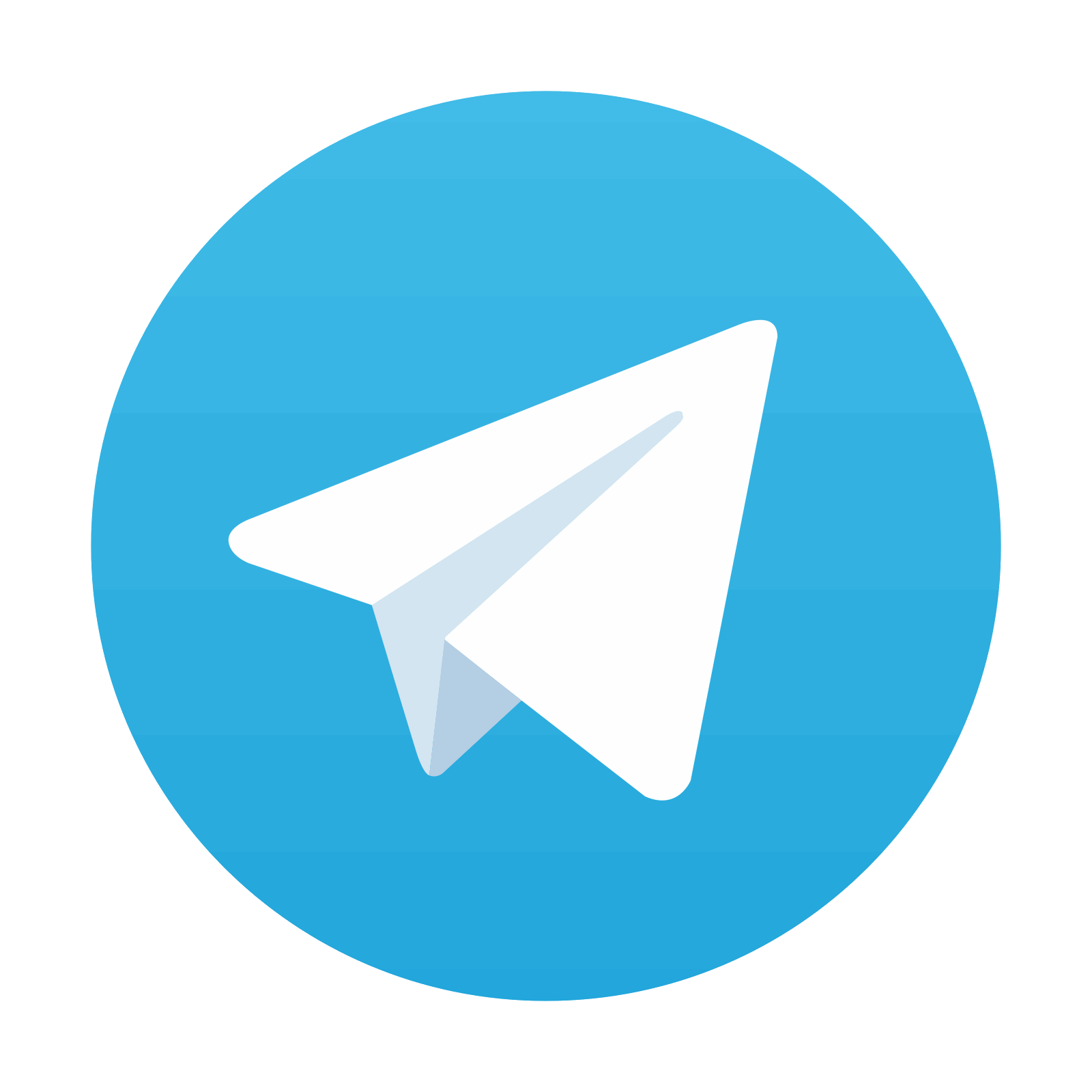
Stay updated, free articles. Join our Telegram channel

Full access? Get Clinical Tree
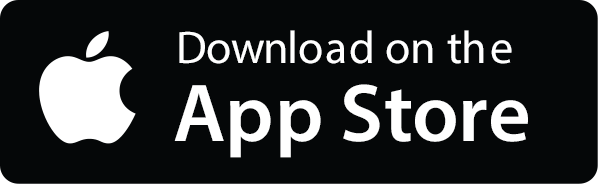
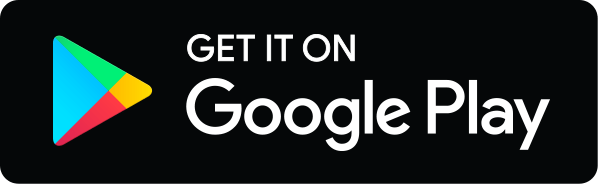