Acknowledgments
We would like to thank Sabine Frank, Susan Notohamiprodjo and Alberto Villagran for their assistance preparing this chapter.
Introduction
In recent years, numerous imaging modalities have been developed to diagnose cardiac disease noninvasively and to measure structural, functional, and bio chemical performance of cardiac tissue. Especially, applications of imaging procedures to assess myocardial perfusion under rest and stress conditions have substantially added to noninvasive diagnosis and assessment of prognosis in patients with suspected and documented coronary artery disease. With the advent of cardiac magnetic resonance imaging (CMR), high-resolution structural and functional imaging has become available. Biochemical and molecular tissue characterization with specific radiotracers has been enabled by the introduction of single photon emission computed tomography (SPECT) and positron emission tomography (PET). Driven by rapid acceptance of PET/CT in patients with oncological diseases, hybrid imaging systems have been developed to promote noninvasive multimodality characterization of disease processes. PET/CT has become a routine imaging procedure in oncology, while PET/MR plays an increasing clinical role in neuroimaging combining anatomic and functional measurements. Cardiac patients also benefitted from multimodality approaches such as PET/CT. PET/CT offers the integrated assessment of regional myocardial perfusion as well as coronary anatomy linking functional measurements with accurate delineation of individual vessel anatomy. This combined approach does not only optimize sensitivity and specificity of the diagnostic process but also guides the therapeutic approach of selective revascularization in patients with advanced disease ( ).
PET/MR on the other hand is currently considered as very attractive cardiovascular research instrumentation, which can provide unique biomarkers of tissue composition and molecular signatures specific to a given disease process. It is hoped that multimodal imaging biomarkers may not only support early diagnosis of disease but also individual selection of patients for possible therapeutic interventions. In this chapter, we will highlight some of the emerging concepts of clinical PET/MR and discuss technical challenges to exploit the full potential of combining two powerful imaging modalities to the benefit of patients with cardiovascular disease.
Finally, given the complexity and heterogeneity of cardiac diseases as well as the usage of different tracers, the specific as well as rather generic protocols will be briefly touched at the end.
Detection of coronary artery disease
Myocardial infarction remains a major cause of mortality in developed countries. However, in recent years there has been a marked decrease of sequels of ischemic heart disease due to the improved early diagnosis and prognostic information provided by modern imaging modalities. Now, coronary artery disease can be detected early by using a variety of imaging procedures. Measurements of left ventricular function, regional perfusion and wall motion allow sensitive and specific detection of impaired coronary flow reserve. Most prominent are the measurements of regional myocardial perfusion, using either contrast agents or radiopharmaceuticals by MR, CT or SPECT, PET. Assessing the homogeneity of myocardial perfusion under rest and stress condition, allows identification of regional impaired coronary flow reserve. Such functional measurements of coronary physiology support the anatomic delineation of regional coronary lesions and have been shown to provide an important adjunct in the decision-making process of regional coronary revascularization ( ). Measurements of coronary flow reserve by noninvasive imaging techniques are accepted gatekeepers for interventional procedures as documented in many guidelines for treating patients with coronary artery disease ( ). Myocardial perfusion imaging (MPI) employing PET provides very accurate delineation of regional perfusion. Several tracers are available for qualitative and quantitative assessment of regional tracer distribution ( ). Attenuation corrected images allow the quantification of blood flow under rest and stress condition in absolute units, which has been extensively validated in preclinical and clinical models. Myocardial perfusion PET imaging with the generator-produced radiopharmaceutical rubidium-82 has become a major diagnostic routine tool in nuclear cardiology ( ). In most clinical settings, the application of MPI has to be restricted to patients with “medium probability” of disease as gatekeeper for interventions. In patients with “low likelihood” of coronary artery disease, the risk of developing coronary artery disease will primarily be based on visualization of coronary calcification and CT-angiography (CTA) while in patients with “high likelihood” of disease invasive diagnostic procedures are indicated. This stepwise approach of applying imaging modalities has been very successful in managing patients with suspected and documented CAD ( ; ). The application of quantitative flow measurements is useful to follow patients under therapy and to assess the significance of a given anatomic coronary alteration in the context of high-risk interventions. The clinical utility of PET and MR in hybrid instrumentation for the assessment of regional perfusion is limited in contrast to PET/CT due to the lack of diagnostic coronary angiography by MR. However, PET, as the most quantitative and most accurate method to assess myocardial blood flow, can be used to cross-validate MR techniques, to assess coronary flow reserve, and help to optimize methods providing validation of advanced MR flow measurements kinetics ( ; ) ( Fig. 5.1 ).
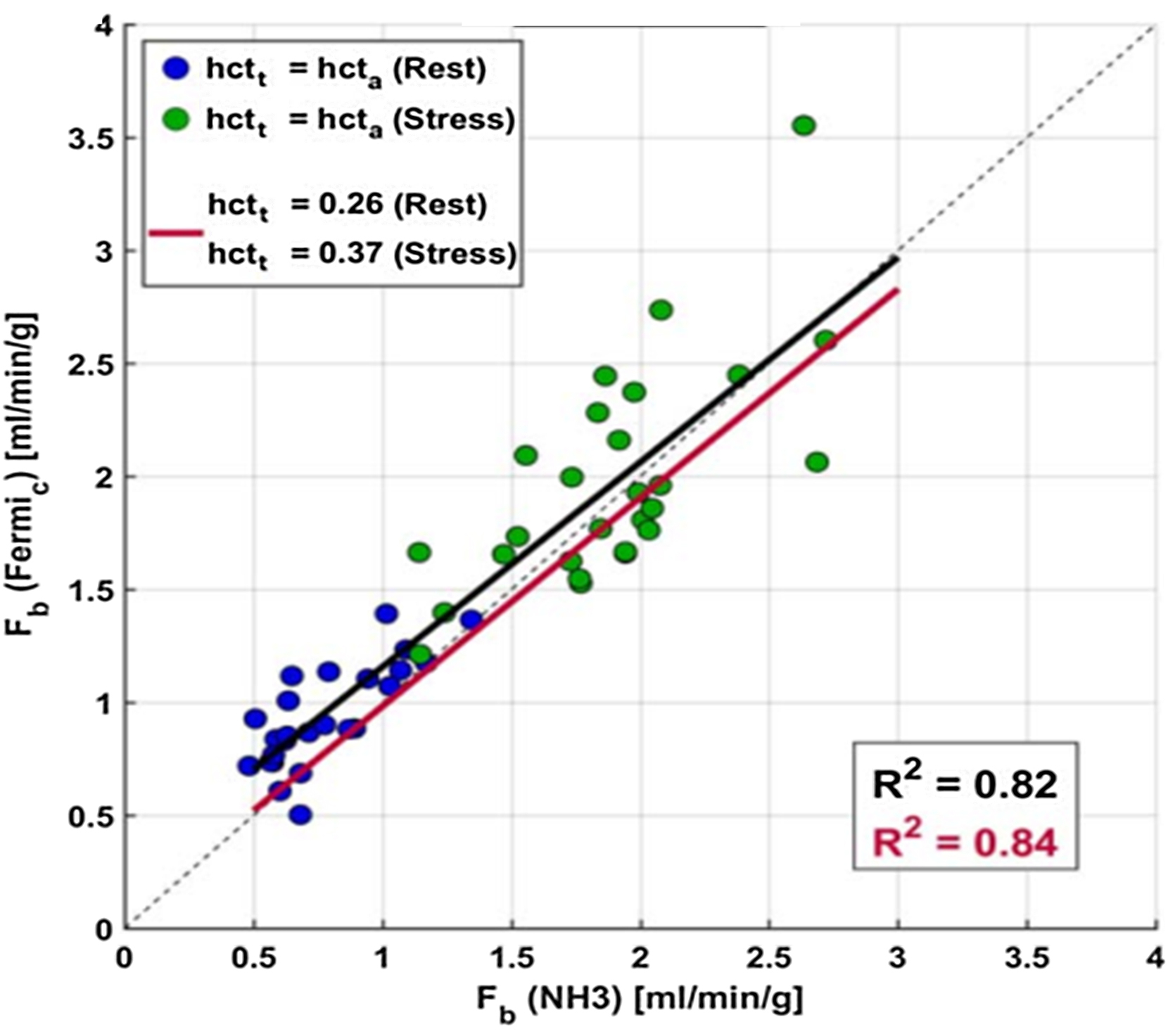
Beyond this methodological aspects, examples for the synergetic use of both modalities can be found: Fig. 5.2 depicts an example of simultaneous PET and MR coronary blood flow measurements employing adenosine stress in a patient with left ventricular hypertrophy and subcritical 3-vessel coronary artery disease.
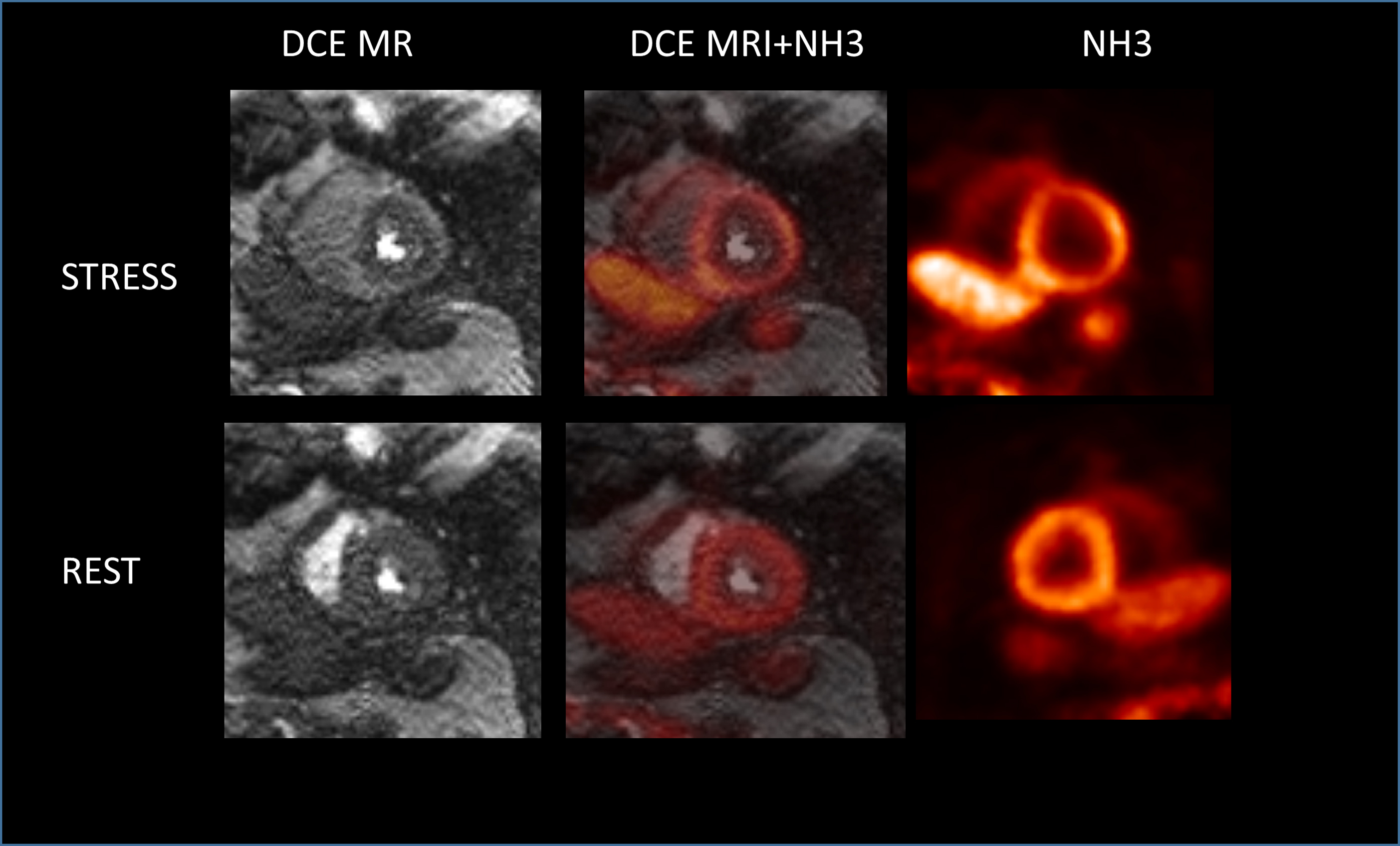
Keypoints
- •
PET with radiotracers such as N-13 ammonia and 0–15 water offers validated regional quantification of myocardial perfusion under rest and stress conditions for accurate diagnosis of CAD
- •
PET/MR provides simultaneous perfusion measurements under identical physiologic conditions yielding perfect crossvalidation of CMR blood flow measurements
- •
PET/MR is inferior to PET/CT for diagnosis of CAD due to low-resolution coronary angiography currently obtainable by CMR
Assessment of tissue viability and infarct size
For many years, PET has been advocated and used for the identification of residual tissue viability in patients with advanced coronary artery disease ( ). By using 18F-fluorodeoxyglucose, the extent of viable tissue can be delineated even in the presence of severe functional impairment. Such measurements have shown to be predictive for functional recovery as well as long-term outcome of patients with advanced ischemic cardiomyopathy ( ). The signal of increased FDG-uptake in cardiac tissue has also been applied for detection of inflammatory reactions in patients with acute myocardial infarction since activated inflammatory cells display an up-regulated glycolytic metabolism. This metabolic imaging concept, however, requires standardization of intrinsic metabolic activity of the myocardium by providing a special diet suppressing glucose metabolism of myocardial cells such as specific lipid rich diets ( ; ).
With the advent of contrast-enhanced magnetic resonance imaging the delayed wash-out of contrast agents in the myocardium has been associated with expanded extracellular space ( ). In myocardial scar tissue, the extracellular space is significantly larger as compared to normal contracting myocardium. Therefore, late contrast enhancement (LGE) of cardiac tissue is indicative of injured and scarred myocardium and has been extensively validated as marker of myocardial infarction. Numerous studies have indicated that CMR technology offers very sensitive detection of small islands of myocardial infarction ( ). Such discrete areas of late enhancement in the presence of normal left ventricular function have shown to be predictive for increased cardiovascular risk ( ). Taking PET and MR measurements together, the extent of myocardial infarction and viable tissue can be assessed simultaneously ( Fig. 5.3 ). Several studies have indicated that in the presence of subendocardial scar the metabolism in epicardial tissue is upregulated, providing evidence of residual viable tissue ( ). The combination of both methodologies has been used to crossvalidate them but also to assess ventricular function as well as the extent of reversible and irreversible myocardial injury by one hybrid imaging modality. The combined identification of viable myocardium has been demonstrated to optimize the predictive information of imaging for functional recovery following revascularization. Rischpler et al. investigated 28 patients with recent acute myocardial infarction within 5–7 days after PCI. Follow-up MR was performed 6 months later. Concordant identification of viable myocardium was associated with better functional outcome. 18% of dysfunctional segments displayed discordant infarction on viability. “PET nonviable segments” recovered less than “PET viable segments” supporting the predictive role of residual metabolic activity ( ). Future prospective studies in larger patient population are needed to address the additional diagnostic or prognostic value by adding two imaging modalities together in patients with history of myocardial infarction and impaired left ventricular function.
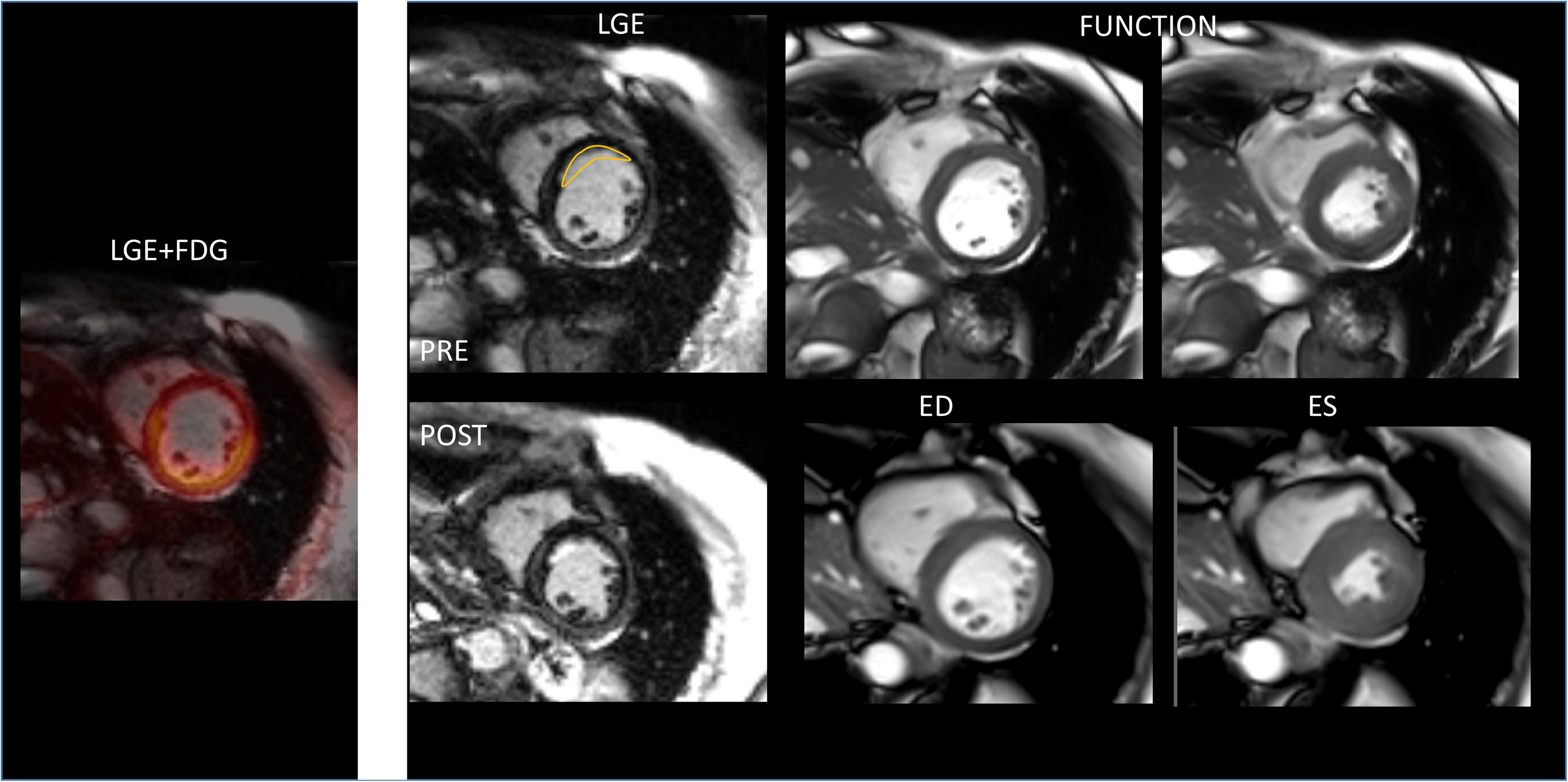
Key points
- •
PET/MR represents the most sophisticated approach to define regional tissue viability in patients with ischemic cardiomyopathy combining markers of glucose metabolism and structural tissue characterization
- •
At present, there is no prospective clinical validation of this hybrid approach in the prediction of clinical outcome in patients with CAD
- •
PET/MR may be clinically helpful in high-risk patient populations with advanced CAD considered for cardiac transplantation
Assessment of inflammatory response to acute ischemic injury
There is increasing knowledge about the inflammatory reaction to acute ischemic injury. It has been shown in animal experiments that an acute ischemic injury is associated with the cardiac infiltration of inflammatory cells, which support the healing process but also contribute to the adverse remodeling leading to ischemic cardiomyopathy ( ). Therefore, there is increasing interest in characterizing the inflammatory reaction as a consequence of acute ischemia by imaging biomarkers in order to identify patients with exaggerated immune response, who may potentially benefit from antiinflammatory therapy ( ; ). For identifying such patients with enhanced immunological response, the imaging of inflammatory cells may provide an important biomarker. Animal studies have shown that, within few hours after myocardial infarction, inflammatory cells migrate into the myocardium and start demarcating the area of ischemic injury ( ). These cells undergo transformation during this reparative process and, during the tissue healing and scarring process, they secrete bio-signals, some of which also prompt neo-angiogenesis. Several markers of cell response including imaging markers of macrophages, angiogenic markers, as well as cytokines, have shown that imaging can be used to identify various aspects of the biological response to acute ischemia ( ; ; ). Especially, the high sensitivity of PET provides the opportunity to visualize these molecular processes by a variety of specific radiopharmaceuticals. However, no clear relationship between these exiting new imaging signals and outcome of ventricular function has been established in prospective trials. The first studies, based on FDG, indicate that high FDG uptake in the area of previous myocardial infarction is associated with impaired outcome of left ventricular function, suggesting that high inflammatory response may be detrimental to further tissue repair. Rischpler et al. studied 49 patients with PET/MR within 5 days after acute myocardial infarction and successful PCI followed by CMR 6–9 months later. The PET/MR studies were performed after dietary preparations to suppress myocardial glucose metabolism. Regional FDG uptake exceeded the area of late gadolinium enhancement (LGE) by CMR. The intensity of FDG uptake correlated inversely with functional outcome. These first results suggest a possible role of FDG uptake as biomarker for the extent of inflammatory reaction to acute ischemia ( ). Fig. 5.4 shows a patient example with acute MI studied by PET/MRI at day 5 after the ischemic event.
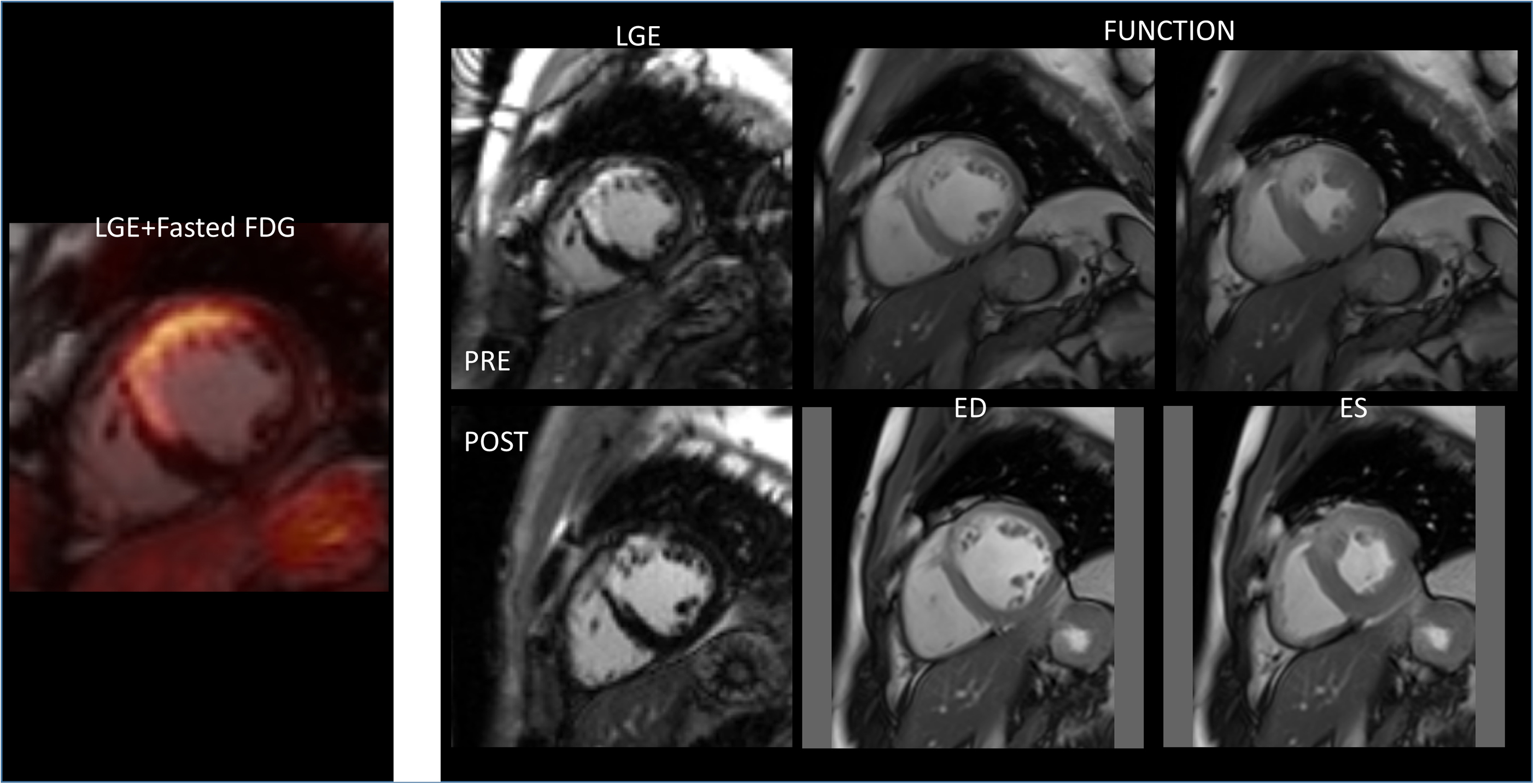
These clinical results are in agreement with animal PET/MR data obtained by Lee et al. demonstrating that areas of LGE had high FDG uptake. Inflammatory cells were primarily responsible for the increased FDG uptake as compared to infarct tissue confirming the hypothesis of acute cell infarction in ischemically injured tissue ( ). There is increasing clinical interest of applying antiinflammatory medication in patients with acute myocardial infarction in order to maximize tissue recovery. However, the first large prospective studies have provided controversial results ( ). At the present time, there is no established antiinflammatory therapy available for patients with acute myocardial infarction. However, this noninvasive characterization of inflammation clearly benefits from the combination of PET and MR because the MR signal (T2) may be associated with tissue edema as marker of acute inflammatory response followed by late enhancement, which is specific for scarring ( ). In addition, the high-resolution approach provided by MR may allow very careful delineation of regional myocardial structure and function following an ischemic injury.
Keypoints
- •
Myocardial infarction is associated with acute inflammatory reaction which results in a highly regulated repair process
- •
PET/MR can uniquely identify and quantitate areas of inflammation and scar in patients with AMI. Together with measurements of left-ventricular function the extent and severity of ischemic injury can be assessed by one integrated imaging modality.
- •
PET/MR is very valuable research tool to monitor molecular, physiological and structural consequences of ischemia using specific markers of inflammation (macrophages, cytokine, receptor expression) neo-angiogenesis, and scarring (ECV, multiparametric MR).
Myocarditis
Cardiac magnetic resonance imaging (CMR) is currently used as standard procedure to diagnose myocardial inflammation. Myocarditis is an inflammatory disease of the myocardium caused by various conditions including viral infections, auto-immune reactions, toxin exposures and drugs or idiopathic factors ( ). Myocarditis occurs more commonly in young subjects, particularly in young men. Additional underlying histopathological changes may vary in different types of myocarditis. Generally, it represents as an inflammatory process related to disproportional immune response against the myocardium, which leads in the long term to myocardial injury ( ). During the acute phase, membrane destruction of the myocytes, induced by various factors, triggers immune response with infiltration of lymphocytes, and macrophages releasing cytokines responsible for high inflammatory changes including hyperemia, edema, and necrosis. At a later phase, inflammation is replaced by collagen deposition with subsequent fibrosis and scar formation in the affected myocardial regions. Definite diagnosis of myocarditis requires endo-myocardial biopsies, which, however, are limited due to the heterogeneous process of disease resulting in low diagnostic accuracy ( ). Therefore, imaging techniques such as CMR and PET have been applied to address various aspects of this disease process by localizing the inflammatory progress. CMR imaging relies on three diagnostic criteria: early and LGE (EGE and LGE) as sign of more permanent changes as well as edema (T2 weighted sequences), which is clearly a sign of early inflammatory reaction. The “Lake Louise criteria” have been proposed; they are based on the relationship of edema as by T2 measurements and by early and late enhancement analysis based on T1 weighted images ( ; ). Relative comparisons of myocardial MR activity versus skeletal muscle activity allow “semiquantitative criteria” to be employed for the diagnosis of myocarditis. An early gadolinium enhancement (EGE) ratio between myocardium and skeletal muscle is obtained by dividing myocardial by skeletal muscle enhancement. Most studies use a value of 4.0 as criterion for myocarditis. The reported values for sensitivity and specificity vary around 70% ( ). The signal contrast is most prominent in the first 2 weeks of myocarditis but subsequently decreases ( ).
A global myocardial signal enhancement ratio of 45% is also considered as a sign of myocarditis. Signal enhancement is defined as signal postcontrast—signal precontrast divided by signal precontrast. The LGE can also be observed but its sensitivity for myocarditis in the acute setting is thought to be only around 60%, correlating with recovery of patients without permanent damage to the heart.
Mahrholdt et al. showed that LGE is most commonly located in the lateral LV-wall. The distribution of LGE may be related to the infecting pathogen ( ). Herpes Virus 6 infection is associated with midwall septal LGE. There is increasing development of parametric imaging mapping procedures, which out-perform the classical “Lake Louise criteria” (LLC). The pooled diagnostic performance of CMR shows a sensitivity, specificity and diagnostic accuracy of 70%, 91% and 79% for T2 mapping while T1 mapping resulted in values of 82%, 91% and 86%. T1 mapping appears to produce the most accurate results. The dynamic nature of the disease process has to be kept in mind. Early imaging is more influenced by edema (T2w), while the latter disease process is more reflected by T1w signal. In concordance, FDG-PET signal may also more likely reflect the acute inflammation while LGE reflects the tissue repair process. PET applications rely on the use of FDG, which provides the advantage of highlighting acute biological signal associated with infiltration of inflammatory cells, which demonstrate high coagulated flocs resulting in an increased regional FDG uptake ( ). Therefore, the metabolic information is complimentary with CMR and reflects tissue alterations secondary to inflammation. PET, specifically, can delineate the acute cellular infiltration and activation, which allows specific differentiation between acute and chronic phase of myocarditis ( Fig. 5.5 ).
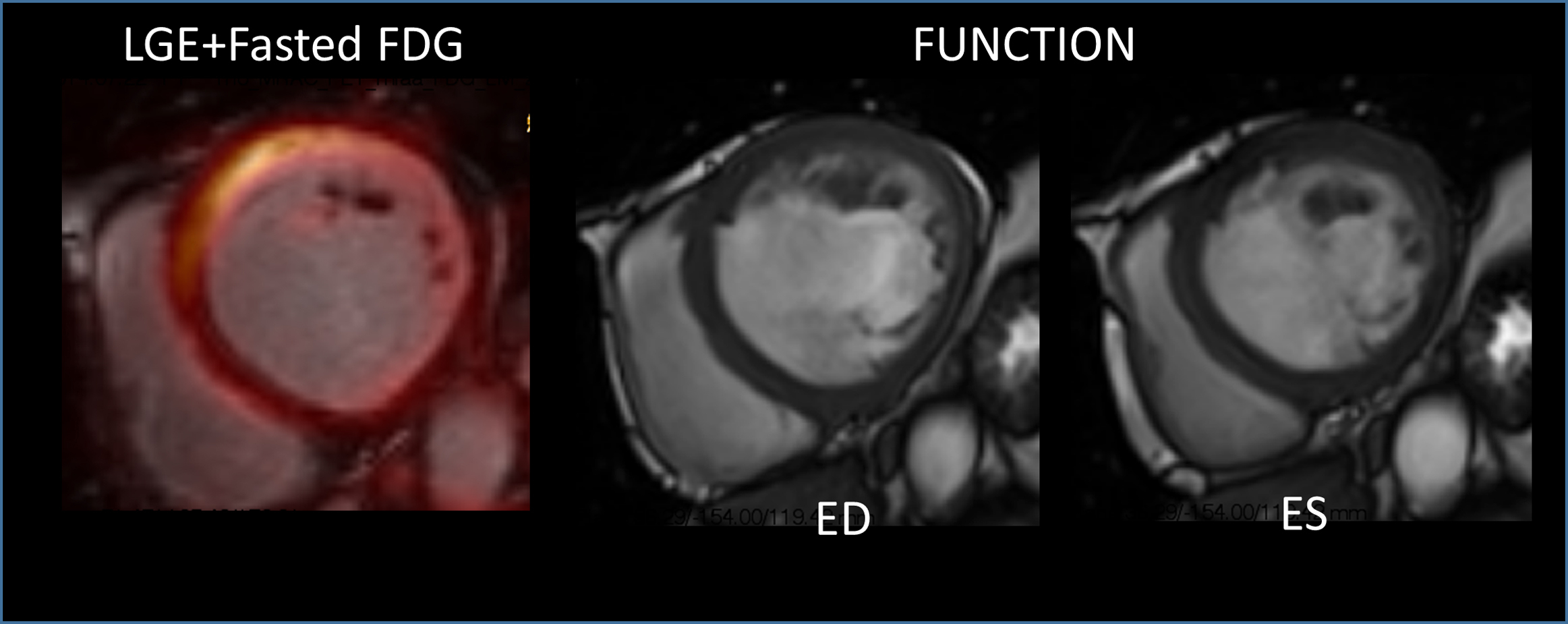
Nensa et al. evaluated prospectively PET and CMR in 65 patients with suspected myocarditis using an integrated PET/MR scanner, The PET/MR study was successfully completed in 55 of 65 patients. Limited dietary suppression of myocardial glucose metabolism was the main cause of non-diagnostic study (eight cases). Compared to CMR (LGE and/or T2 imaging), sensitivity of FDG PET was 74% and specificity 97% ( ). There was a high regional agreement of both CMR and PET abnormalities yielding a kappa value of 0.73. However, there were patients with myocarditis proven by biopsy, who did not show corresponding CMR abnormalities in areas of increased FDG uptake. Thus, FDG-PET may provide complimentary information by its high sensitivity for inflammatory cell infiltration, which may be useful for early diagnosis of the inflammatory process prior the occurrence of structural changes detectable by CMR. Future and larger longitudinal studies will be required to address this hypothesis. Parametric imaging techniques integrating CMR and PET information may optimize the diagnostic performance of both tests. First data linking spatial distribution of imaging signals with infectious agents may help to specifically diagnose not only the inflammatory process, but also disease subtypes ( ). Finally parametric imaging will be helpful for guiding biopsy to obtain molecular characterization of the inflammatory process.
Keypoints
- •
CMR is an established imaging modality to detect and to localize myocarditis and to follow patients longitudinally using criteria of contrast enhancement (EGE; LGE) and edema (T2).
- •
PET/MR may provide more specific information on myocardial infiltration of inflammatory cells and may therefore offer early diagnosis and therapy monitoring
- •
Parametric data analysis integrating multimodal imaging signals may help to phenotype subtypes of myocarditis
Assessment of cardiac amyloidosis
There is a clinical need for imaging methods in order to specifically depict infiltration of the myocardium by amyloidosis. There are two subtypes of cardiac involvement entailing light-chain amyloidosis (AL) as well as transthyretin amyloidosis (TTR). The later type of cardiac involvement is associated with cardiomyopathy and poor clinical prognosis ( ). Application of bone seeking radiopharmaceuticals such as 99 m technetium pyruvate DBDHMDP in cardiac amyloid has already proven to be useful for the diagnostic process ( ). More recently, F-18 fluoride has been employed as PET radiotracer to identify the cardiac involvement ( ). A recent meta-analysis of published F-18 fluoride PET studies revealed a pooled sensitivity of 63% and specificity of 100% compared to biopsy.
In clinical routine, CMR imaging is currently considered the method of choice to assess the impairment of global left ventricular function and to localize the infiltration of amyloid. CMR diagnosis is again based on early extension of the extracellular space, which can be depicted by pre- and postcontrast T1 mapping. These CMR imaging biomarkers have both diagnostic and prognostic value in cardiac amyloidosis. However, the CMR measurements are limited since they cannot distinguish between various subtypes of cardiac amyloidosis. By combining MR and PET measurements, such specific differentiation may become clinical reality. Recent studies by Andrews et al. applied PET/MR in a subgroup of 53 patients with proven ATTR as well as ER amyloidosis ( ). The study revealed that F-18 fluoride uptake was low in normal myocardium producing a negative myocardial contrast since the blood pool activity is higher than the myocardial tracer uptake. Only in patients with TTR cardiac amyloidosis, there was a statistically significant increase of uptake within septal areas of the left ventricle as compared to normal volunteers and light chain cardiac amyloidosis patients. The difference of PET signals was most pronounced in areas also identified by LGE. This very specific application of PET/MR indicates complimentary information, which can be obtained and actually help to identify patients who have poor clinical prognosis and may potentially benefit from specific therapies, such as the newly approved drug tafamidis in the case of transthyretin amyloidosis ( ). The costs of tafamidis therapy regimen are high, underlining the importance of evaluating the effectiveness of this therapy by imaging biomarkers. Multimodal biomarkers that can select patients for therapy and monitor response in individual patients will be clinically useful given the current price tag of these therapies. With the availability of new treatments and the better outcome in the case of intervention on “early stage” disease, however, imaging faces the unmet clinical need of achieving early diagnoses ( Fig. 5.6 ).
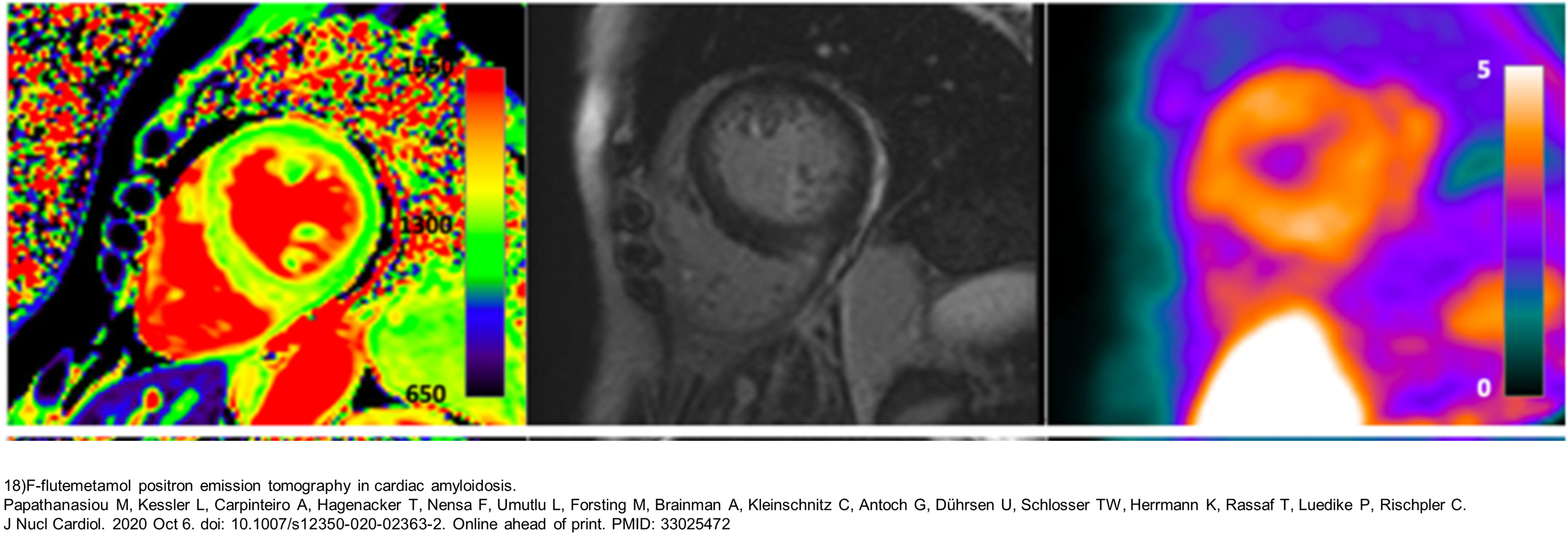
Keypoints
- •
Cardiac amyloidosis represents an under diagnosed cardiac disease
- •
Transthyretin cardiac amyloidosis is associated with poor prognosis, but new drugs promise specific molecular treatment options
- •
PET/MR with F-18 Fluoride may provide noninvasive differentiation of AL and TTR cardiac amyloidosis
Sarcoidosis
Cardiac involvement by sarcoidosis, as granulomatous disease with ill-defined etiology, can cause heart failure, conduction abnormalities and arrhythmias as well as sudden death. The prevalence of cardiac involvement of systemic sarcoidosis remains unclear because a significant number of patients remain asymptomatic. In addition, diagnosis of cardiac involvement by biopsy or imaging is challenging.
The more common cardiac presentation is 3 rd degree heart block, occurring in 25%–30% of patients. About 15%–50% of deaths associated with sarcoidosis have been attributed to cardiac involvement ( ). The diagnosis by cardiac biopsy is limited by low sensitivity due to the patchy nature of disease ( ). ECG changes and echocardiography are commonly utilized as supporting diagnostic tools but lack specificity. The Heart and Rhythm Society (HRS) Expert Consensus Statement proposes guidelines for diagnosis of cardiac involvement by various imaging technologies including PET to identify inflammatory conditions by increased local FDG accumulation. FDG PET has been extensively studied with reported values for sensitivity and specificity between 70% and 100% ( ; ).
Because of the patchy nature of disease, careful dietary preparation is necessary to maximize the diagnostic performance of FDG-PET imaging. The SNMMI-ASNC consensus document suggests special diet for patients with suspected cardiac involvement with sarcoidosis ( ). At least two high-fat/low carbohydrate meals are recommended followed by 4–12 h fasting ( ). Fig. 5.5 shows an active cardiac sarcoidosis case. Because of the difficulties of suppressing normal cardiac glucose metabolism by dietary means, alternative tracers for the identification of sarcoid granulomatosis have been evaluated. F-18 Fluoro-thymidine (FLT) has been shown in a small cohort of patients to out-perform FDG PET in the diagnosis of cardiac and neural involvement ( ). Future studies evaluating specific tracer approaches are needed to avoid the challenges of dietary control of glucose metabolism in individual patients.
Cardiac MR imaging allows accurate assessment of myocardial involvement by sarcoidosis and provides structural localization of granulomata by LGE imaging. However, LGE reflects changes in extracellular volume, but does not identify the inflammatory disease process. Therefore, the combination of PET/MR offers complimentary information. Since therapeutic options not only include immunosuppressive treatment, but also implantation of automatic cardiac defibrillators, optimization of diagnostic processes is mandatory.
A recent study by Dweck et al. compared the diagnostic usefulness of PET/MR imaging in a cohort of 25 patients with suspected cardiac involvement of sarcoidosis ( ). 18 patients had a proven diagnosis by biopsy of extracardiac tissue. Seven patients displayed a high probability of cardiac sarcoidosis based on clinical presentation, imaging results and electrocardiographic abnormalities. The cardiac FDG-PET findings were compared to a control group of fasted 19 patients studied with PET/CT. Eight patients were positive on PET and MR, suggesting cardiac sarcoidosis, while eight patients were negative by both imaging methods. Eight patients (6 focal, 2 diffuse) were positive based on FDG uptake. Similar FDG pattern was also observed in the control group. However, the maximum FDG target to normal myocardium ratio was higher in the patients with suspected cardiac sarcoidosis. These findings emphasize the need for strict dietary control to suppress normal myocardial FDG uptake in order to enhance diagnostic confidence. However, abnormal FDG uptake in CMR negative areas of left ventricular myocardium may be a marker of active inflammation and, thus, indicative of early cardiac involvement, which may represent a therapeutic target.
No prospective studies exist to evaluate the prognostic role of FDG PET. However, retrospective studies suggest that a positive FDG-PET study is associated with risk of ventricular arrhythmias and death ( ). Among 118 patients with cardiac sarcoidosis, an abnormal FDG-PET finding was associated with hazard ratio of 3.9 ( P < .01) with adverse events (death or sustained ventricular tachycardia). Similar results were also observed in another study where 40/114 patients (35%) displayed LGE. LGE and extent of LGE identified patients with subsequent death, aborted SCD or ICD discharge as single variants in multivariate analysis. T2 imaging identified 14% of patients with active disease ( ).
In another study in 51 patients, combining CMR and PET yielded high sensitivity (94%) for detection of cardiac sarcoidosis. Over a median follow-up time period of 2.2 years, 18 cardiac events were observed; PET and LGE were independent predictors of cardiac events, but abnormalities present on both imaging modalities were the strongest predictors of major adverse cardiac events ( ). Despite these promising first observations employing PET and MR, prospective clinical studies are needed to confirm the diagnostic and prognostic value of PET/MR in patients with suspected cardiac sarcoidosis ( Fig. 5.7 ).
