Fig. 22.1
Biological factors supporting plaque vulnerability (Nighoghossian et al. 2005) (Permission to use figure and legend granted by Lippincott, Williams & Wilkins; http://lww.com)
These various stages of atherosclerotic plaque development with specific histopathological characteristics may result in clinically recognisable events.
Further in this chapter, we will categorise the nuclear imaging techniques using different radiopharmaceuticals that are currently available to visualise these various stages. We will discuss the specific properties of each with regard to visualisation of the different pathways associated with vulnerability, including inflammation, proteolysis, apoptosis, angiogenesis, and lipid accumulation in carotid plaque formation.
22.3 Functional Imaging of Carotid Artery Plaque with SPECT/CT and PET/CT
Nuclear imaging modalities are capable of visualising molecular processes. Clinical positron emission tomography (PET) has in general an advantage over single-photon emission computed tomography (SPECT) in having a 2–3 times better spatial resolution, unless the use of a collimator.
The spatial resolution for PET is approximately 5 mm and is only useful in larger arteries. Also PET reveals the capability to quantify tracer activity in the region on interest. Restricted resolution can be partially counteracted by coregistration of scintigraphic images with CT (SPECT/CT and PET/CT hybrid camera systems) or with MRI. CT and MRI will be helpful for partial volume correction, but most importantly the advantage of coregistration is precise anatomic information concerning tracer uptake and to visualise aspects that are not depicted by PET and SPECT, such as stenosis and the plaque extent. If PET and SPECT are to gain a position as a clinical instrument in the search for the vulnerable plaque, specific tracers will be needed to image components which play an important role in the formation and progression of vulnerable plaques (Table 22.1) (Glaudemans et al. 2010).
Table 22.1
Radiopharmaceuticals for imaging atherosclerosis
Radiopharmaceutical | Target |
---|---|
Lipid accumulation | |
99mTc-LDL/oxLDL/ac-LDL | Foam cells |
99mTc-LOX-1-mAb | Foam cells |
99mTc-β-VLDL | Lipoproteins |
125I/99mTc-MDA2, 125I-IK17 | Lipids |
Inflammation | |
99mTc/125I-MCP-1 | Macrophages and monocytes |
99mTc/123I-IL-8 | Neutrophils |
123I-IL-1 RA | Monocytes and lymphocytes |
123I- or 99mTc-IL-2 | Lymphocytes |
99mTc-fucoidan | P-selectin |
18F-FDG | Glucose activity |
Calcification | |
18F-sodium fluoride | Active mineral deposition |
Thrombosis | |
111In-platelets | Platelets |
99mTc-apcitide/P280 | Activated platelets |
99mTc-DMP444 | Activated platelets |
99mTc-fibrin-binding domain (FBD) | Fibrins |
99mTc-labelled fibrin α-chain peptide | Fibrins |
Apoptosis | |
99mTc-annexin A5 | Apoptotic cells |
Angiogenesis | |
111In-bevacizumab | VEGF activity |
123I-VEGF165 | VEGF receptor |
99mTc-endothelin | Smooth muscle cells |
11C-L-159,884 | Angiotensine-1 receptor |
89Zr- bevacizumab | VEGF activity |
18F-galacto-RGD | αvβ3 integrin receptor |
99mTc-MMP-tracers | Metalloproteinase (several subtypes) |
22.3.1 Inflammation
Inflammation plays a key role in early atherosclerotic plaque development and in plaque destabilisation. Especially, macrophages play a central role in plaque pathogenesis. By ingesting lipids, they transform into foam cells and produce a large array of pro- and anti-inflammatory cytokines. The highly inflammatory vulnerable plaque is characterised by an abundance of inflammatory cells and proteins that are all potential targets for molecular imaging tracers. Therefore, molecular imaging of inflammatory activity in the plaque may predict vulnerability and stroke risk. The most widely studied imaging method to identify inflammatory processes in carotid artery plaques is radionuclide scintigraphic imaging by PET. Radionuclide tracers for macrophage metabolism, macrophage recruitment, and inflammatory markers have also been developed and have potential to image inflammatory activity (Masteling et al. 2011). 18F-fluorodeoxyglucose (FDG) is a glucose analogue that is taken up by glucose-using cells and accumulates in proportion to metabolic activity. FDG-PET has extensively been used in cancer patients. Some cancer patients undergoing FDG-PET showed uptake of FDG in the large arteries and they were retrospectively identified as having risk factors for atherosclerosis (Yun et al. 2002). This suggested that atherosclerotic plaques may be suitable for FDG-PET imaging. In atherosclerosis, FDG-PET is thought to identify only those plaques that are most actively inflamed and at highest risk for instability and therefore is a potential method for noninvasive identification of an unstable carotid plaque (Fig. 22.2). An early clinical study using FDG-PET in carotid artery plaques was performed in 2002, showing more accumulation of FDG in unstable plaques (Rudd et al. 2002). FDG-PET signals have also shown to correlate with histological macrophage staining or macrophage markers in human atherosclerotic plaques (Masteling et al. 2011). In another clinical study, 12 patients with a recent TIA underwent FDG-PET and high-resolution MRI. FDG uptake was high in 83 % of patients with lesions that were compatible with the patients’ presenting symptoms (Davies et al. 2005). In a recent study, new symptomatic carotid stenosis, inflammation-related FDG uptake was associated with early stroke recurrence, independent of the degree of stenosis. Plaque FDG-PET may identify patients at highest risk for stroke recurrence, who may be selected for immediate revascularisation or intensive medical treatment (Marnane et al. 2012). Bucerius et al. showed that carotid inflammation as revealed by FDG-PET is also highly prevalent in the coronary artery disease population and is associated with obesity, age over 65 years, history of hypertension, smoking, and male gender. Artery wall FDG uptake increased when components of the metabolic syndrome clustered (Bucerius et al. 2011). Rominger et al. found out in a large cohort of 932 cancer patients increased FDG uptake in major arteries emerged as the strongest predictor of a subsequent vascular event. Concomitant severe vascular calcifications seemed to impart a particularly high risk (Rominger et al. 2009). FDG uptake within an arterial wall or plaque can be quantified in several ways, but there is always a partial volume error (PVE), depending on the spatial resolution of the imaging technique that is being used (Rousset et al. 1998), whereas PET has an advantage over SPECT in having a 2–3 times better spatial resolution. Izquierdo-Garcia et al. studied the reproducibility of methods of quantification by MR-guided FDG-PET in symptomatic carotid artery plaques and compared quantification methods to a gold standard technique using the Patlak analysis (Izquierdo-Garcia et al. 2009; Patlak et al. 1983). MR-guided FDG-PET showed to be a highly reproducible technique. A major disadvantage of FDG-imaging is its nonspecificity. To this context, a FDG-positive scan of the carotid area may be as well caused for example physiological muscle or brown fat uptake. CT and MRI may be helpful to distinguish vascular from extravascular uptake. However, there is still a need for more specific PET tracers which are suitable for the detection of the unstable carotid plaque.
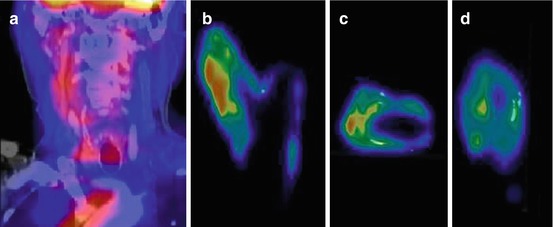
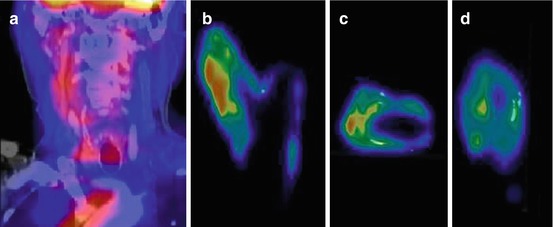
Fig. 22.2
Clinical fused PET/CT image from a patient showing FDG uptake in the affected right carotid artery (a). Coronal (b), transverse (c), and sagittal plane (d) of corresponding μPET images in the same patient showing also patchy FDG uptake and calcified areas (white depositions) (Masteling et al. 2011)
While ample data exist about the distribution of FDG uptake in the arterial tree and its colocalisation with plaque calcification, only limited data exist for choline and fluorocholine. 18F-labelled fluorocholine (18F-fluorocholine) has been recently introduced for imaging of the brain and diagnosis of prostate cancer disease. Choline is taken up into cells by specific transport mechanisms and phosphorylated by choline kinase. Afterwards, it is metabolised to phosphatidylcholine and eventually incorporated into the cell membrane. Increased choline uptake has been shown in tumour cells and activated macrophages.
Recently, Bucerius et al. reported the first data correlating 18F-fluorocholine uptake and morphological wall alterations in aortic and common iliac arteries of five patients (Bucerius et al. 2008).
Their first observations suggested that fluorocholine uptake and calcification sites are mostly not collocated, similar to the findings of previous studies with FDG.
An another previous study found out that 11C-choline uptake and calcification in the aortic and common carotid arterial walls are common in elderly men (Kato et al. 2009). Radiotracer uptake and calcification are, however, only rarely colocalised. 11C-choline has the potential to provide information about atherosclerotic plaques independent of calcification measurement with CT.
Another radionuclide tracer that has been used in carotid artery patients is 99mtechnetium-interleukin-2 (99mTc-IL-2) SPECT. Higher serum IL-2 levels are associated with increased carotid artery IMT, a predictor of stroke and vascular disease (Elkind et al. 2005). 99mTc-IL-2 is therefore also used for imaging carotid atherosclerosis in humans (Fig. 22.3). Fourteen patients (16 plaques) eligible for endarterectomy underwent 99mTc-IL-2 scintigraphy before surgery. Another nine patients (13 plaques) received atorvastatin or a standard hypocholesterolaemic diet and scintigraphy was performed before and after 3 months of treatment. 99mTc-IL-2 accumulated in vulnerable carotid plaques and the accumulation correlated with the amount of IL-2R + cells within the plaque (measured ex vivo by histology). Also, the amount of 99mTc-IL-2 within the plaque was influenced by lipid-lowering treatment with a statin (Annovazzi et al. 2006). 99mTc-IL-2 seems a promising tracer that could provide useful information for the selection of infiltrated vulnerable plaques at risk of rupture. However, it is not yet commercially available and a major drawback is the complexity and duration of the labelling procedure (Signore et al. 2003). Furthermore, the clinical development of 18F-IL-2 could be of additional importance because of its higher resolution (Di Gialleonardo et al. 2012). Therefore, of all radionuclide tracers, 18FDG has currently the most validated and clinical potential to identify inflamed plaques in patients with carotid artery disease, although target finding is not very specific.
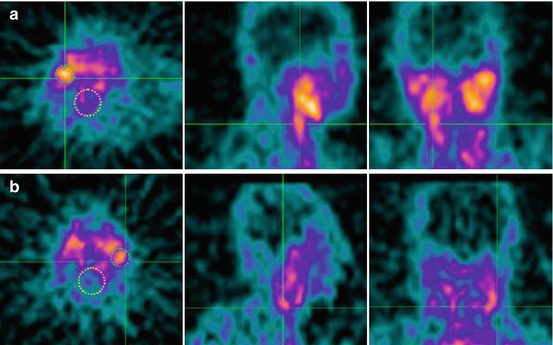
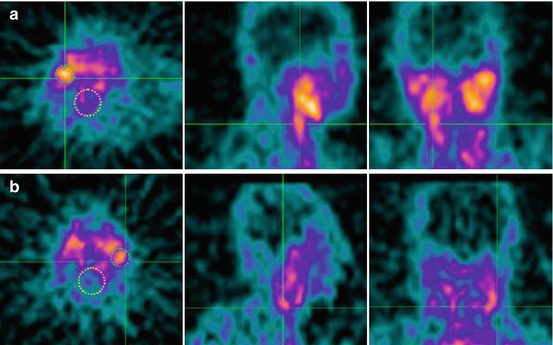
Fig. 22.3
99mTc-IL2 scintigraphy in two patients with a plaque in the right (a) and the left (b) carotid. Images are transaxial, sagittal, and coronal views at the level of the carotid bifurcation. Circles are drawn on the carotid region (small) and larger circles are drawn on the vertebral regions, serving as background to calculate the uptake ratio (carotid/background) (Annovazzi et al. 2006)
22.3.2 Lipid Accumulation
Lipid accumulation not only plays a role in the initial phases of atherogenesis, but is also important in the stability of the atherosclerotic plaque. Unstable lesions were shown to have a much greater area occupied by lipids (Dangas et al. 1998). In addition, elevated oxidised low-density lipoprotein (LDL) levels play a role in the transition from stable to unstable plaques and are associated with higher risk for atherosclerotic complications. In carotid artery disease, technetium-99m-labelled LDL (99mTc-LDL) was used for identification of plaque and appeared to accumulate depending on plaque composition (Lees et al. 1988). Another study in carotid artery plaques compared 99mTc-oxLDL uptake between normal carotid arteries and patients with a carotid artery stenosis. They found significantly higher uptake of 99mTc-oxLDL in carotid plaques compared with normal carotid arteries. However, relationship between 99mTc-oxLDL uptake and plaque vulnerability by comparison among stable and unstable plaques was not assessed (Iuliano et al. 1996). Although clinical experience in imaging lipid accumulation is limited, some results suggest that at-risk plaques with high lipid content may be detected by noninvasive imaging in the future.
22.3.3 Proteolysis
Release of proteolytic enzymes such as matrix metalloproteinases (MMPs) and cathepsin cysteine proteases (CCPs) has been suggested as a mechanism of cap erosion and thus plaque destabilisation. Several studies in atherosclerotic plaques have shown proteolytic activity in relation to plaque instability (Loftus et al. 2000; Morgan et al. 2004). Therefore, noninvasive visualisation and quantification of MMP and CCP activity is of great potential in risk assessment of carotid artery pathology. Radiolabelled molecules designed to specifically target proteolytic activity have been developed for SPECT and PET. For example, radiolabelled MMP inhibitors that bind to a broad spectrum of MMPs have been studied in mice and showed higher uptake in carotid artery stenosis compared to normal arteries (Schafers et al. 2004). Histological analysis showed colocalisation of the specific tracer and MMP-9. In rabbits, MMP activity could be detected by noninvasive SPECT imaging and correlated well with immunohistochemically verified macrophage infiltration and presence of MMP-2 and MMP-9 within the atherosclerotic plaque. In addition, statin therapy and dietary modification showed to decrease MMP activity as visualised by SPECT and again immunohistochemical correlations were high (Fujimoto et al. 2008). This indicates that SPECT may be an important tool for monitoring the effects of new therapy strategies. However, most of the tracers have only been validated in animal studies and only 111In-DTPA-N-TIMP-2 has been used in human (Van de Wiele and Oltenfreiter 2006). The development of new tracers in atherosclerotic plaques is complicated by a so-called poor target-to-background ratio, which means that background activity makes it hard to distinguish plaque uptake from surrounding healthy tissue.
22.3.4 Apoptosis
Apoptosis also plays an important role as a feature of human atherosclerotic plaque progression. The risk of plaque rupture depends in part upon the presence of a necrotic core, containing lipids, dead cells, and debris with a thin fibrous cap (Libby and Theroux 2005). Carotid artery plaques with an increased necrotic core and a thin fibrous cap due to apoptosis of macrophages or smooth muscle cells are known to be instable and are at high risk for rupture.
Imaging of apoptosis in carotid artery plaques has mainly been studied in animal models by targeting markers of apoptosis such as annexin A5. In a rabbit model, technetium-99m-labelled annexin A5 showed a higher uptake in atherosclerotic lesions compared with controls (Kolodgie et al. 2003). In another rabbit study, annexin A5 (111In-labelled annexin A5) was imaged in combination with MMP activity (99mTc-labelled matrix metalloproteinase inhibitor (MPI)) using SPECT/CT (Haider et al. 2009). Annexin A5 and MPI uptake were both visualised in atherosclerotic animals and were interrelated.
In a clinical pilot study of four patients with a history of TIA caused by symptomatic carotid artery stenosis, annexin A5 uptake corresponded well with histopathological characterisation of vulnerability of the endarterectomy specimens (Kietselaer et al. 2004). Unstable plaques showed higher uptake of annexin A5 while in stable plaques no uptake of annexin A5 was seen after SPECT imaging. Although obviously more clinical studies in apoptotic markers are needed, so far 99mTc-annexin A5 is one of the few tracers that have been used in the clinical setting of acute vascular disease. It should be mentioned that annexin A5 is not specific for apoptosis only, as many forms of vascular stress may lead to an annexin V signal. Caspase-3-dependent pathway also plays an important role in apoptosis. Specific targeting of caspase-3 may be more specific, but not yet validated in human (Faust et al. 2007).
22.3.5 Angiogenesis
In atherosclerotic plaques, the formation of microvessels has been studied as a possible contributing factor to plaque destabilisation and rupture. Neo-vessels in carotid artery plaques are fragile and prone to rupture and may cause plaque growth and intraplaque haemorrhage, resulting in a high-risk plaque (Takaya et al. 2005). Several angiogenic cytokines such as vascular endothelium growth factor (VEGF), integrins, or angiotensin may be potential targets for molecular imaging of angiogenesis in plaque formation (Holm et al. 2009; Slart et al. 2008). Angiotensin 1 receptor (Szabo et al. 2001) and VEGF radionuclide tracers (Nagengast et al. 2007) have already been used in other diseases in which angiogenesis plays an important role, but not in atherosclerosis. For example, noninvasive imaging of angiogenesis by radionuclide VEGF tracers (Blankenberg et al. 2006) has been performed.
Hypoxic conditions in atherosclerotic angiogenesis can be imaged with radiolabelled 2-nitroimidazoles, 18F-FAZA, 18F-MISO, and 61Cu-ATSM (Kurihara et al. 2012). To date, it is unclear if the concentration of hypoxia in plaques will be sufficient to visualise hypoxia. VEGF and VEGF-receptor expression is also induced in response to hypoxia, and therefore these VEFG receptors represent reasonable targets for imaging of mediators of ischaemia-induced angiogenesis, also in artherosclerotic plaques (Virmani et al. 2005). Previous studies used 111In-VEGF121 in different animal models, for example, in rabbits (Lu et al. 2003). The anti-VEGF monoclonal antibody VG76e has been labelled with the PET tracer 124I and following successful validation in a mouse model (Collingridge et al. 2002). A humanised monoclonal antibody, bevacizumab, binds strongly to VEGF by the tyrosine kinase receptor and neutralises all isoforms of the ligand VEGF-A (Ferrara and vis-Smyth 1997; Gerber and Ferrara 2005). This makes bevacizumab also an interesting antibody to visualise angiogenesis. 111In- bevacizumab and 89Zr-bevacizumab reveal high uptake in vascularised tissues (Nagengast et al. 2007). It is also feasible to visualise VEGF expression within carotid endarterectomy specimens using 89Zr-bevacizumab PET. 89Zr-bevacizumab accumulation in plaques is specific and tissue-to-background ratio correlates with immunohistochemistry scores (Golestani et al. 2012) (Fig. 22.4). Also radiolabelled RGD peptides as integrin alpha(v)beta3-targeted PET tracers may play an important role for the imaging of angiogenesis (). Integrin alpha(v)beta3 is expressed on the plasma membrane in an active status in which it binds its ligands and transduce signals when exposed activating external stimuli of angiogenesis in atherosclerosis such as VEGF (Laitinen et al. 2009).
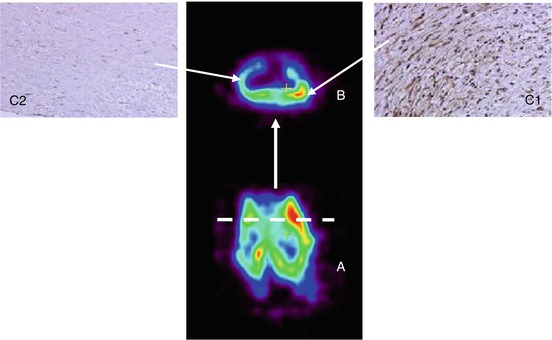
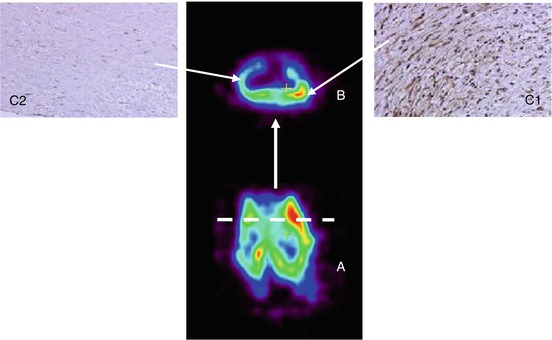
Fig. 22.4
(A) Coronal view of carotid endarterectomy specimen incubated with 89Zr-bevacizumab using μPET. (B) Dashed lines show the levels of transverse views. (C1) Immunohistochemistry of a slide with high 89Zr-bevacizumab uptake shows intense staining of VEGF in CEA specimens. ( C2) Immunohistochemistry of a slide with low 89Zr-bevacizumab uptake shows diminished VEGF staining. VEGF vascular endothelial growth factor
22.3.6 Thrombosis
In carotid artery plaques, thrombotic activity is associated with stroke and TIAs (Sayed et al. 2009). Unstable carotid artery plaques express a variety of thrombomodulatory factors and expression of these factors is higher in unstable plaques compared to stable plaques (Spagnoli et al. 2004). In a large series of carotid endarterectomy specimens, thrombotic activity was seen in 74 % and 35 % of patients with ischaemic stroke and TIAs, respectively, and only in 14 % of asymptomatic patients. In stroke patients, thrombotic activity was seen until several months after the first cerebrovascular event (Sayed et al. 2009). These findings suggest that thrombotic activity plays a crucial role in plaque rupture and the pathogenesis of stroke (Hermus et al. 2010). Several nuclear tracers are available to image thrombus activity (Table 22.1); however, most are evaluated in older studies.
22.3.7 Plaque Calcification
Calcification not only plays a role in stabilising the plaque but is also related to cardiovascular risk (Mauriello et al. 2011). The association of aortic calcification with cardiovascular events and all-cause mortality has been emphasised in several reports. Arterial calcification has been traditionally determined by CT, whereas inflammation mainly mediated by macrophage activity has been assessed by FDG-PET over the last few years. The increasing use of 18F-sodium fluoride (18F-NaF) PET has raised the theoretical possibility of studying active mineral deposition in the atherosclerotic plaque perhaps years or even decades earlier than previously. To date there have been some clinical studies (Derlin et al. 2010, 2011a, b; Dweck et al. 2012) that have explored the feasibility of using 18F-NaF PET/CT in assessing the calcification component of atherosclerosis. Derlin et al., in a retrospective study, (Derlin et al. 2011b) evaluated the correlation of 18F-NaF accumulation in the common carotid arteries of neurologically 269 oncologic asymptomatic patients with cardiovascular risk factors. 18F-NaF accumulation in the common carotid arteries was analysed both qualitatively and semiquantitatively by measuring the blood-pool-corrected standardised uptake value (target-to-background ratio) and comparing it with cardiovascular risk factors and calcified plaque burden. 18F-NaF uptake was observed at 141 sites in 94 (34.9 %) patients. Radiotracer accumulation was colocalised with calcification in all atherosclerotic lesions. 18F-NaF uptake was significantly associated with age (P < 0.0001), male sex (P < 0.0001), hypertension (P < 0.002), and hypercholesterolemia (P < 0.05). The presence of calcified plaque correlated significantly with these risk factors but also with diabetes (P < 0.0001), history of smoking (P = 0.03), and prior cardiovascular events (P < 0.01). There was a highly significant correlation between the presence of 18F-NaF and number of present cardiovascular risk factors (r = 0.30, P < 0.0001). It was concluded that carotid 18F-NaF uptake is a surrogate measure of calcifying carotid plaque, correlates with cardiovascular risk factors, and is more frequent in patients with a high-risk profile for atherothrombotic events but demonstrates a weaker correlation with risk factors than does calcified plaque burden. This study provides a rationale to conduct further prospective studies to determine whether 18F-NaF uptake can predict vascular events or if it may be used to monitor pharmacologic therapy.
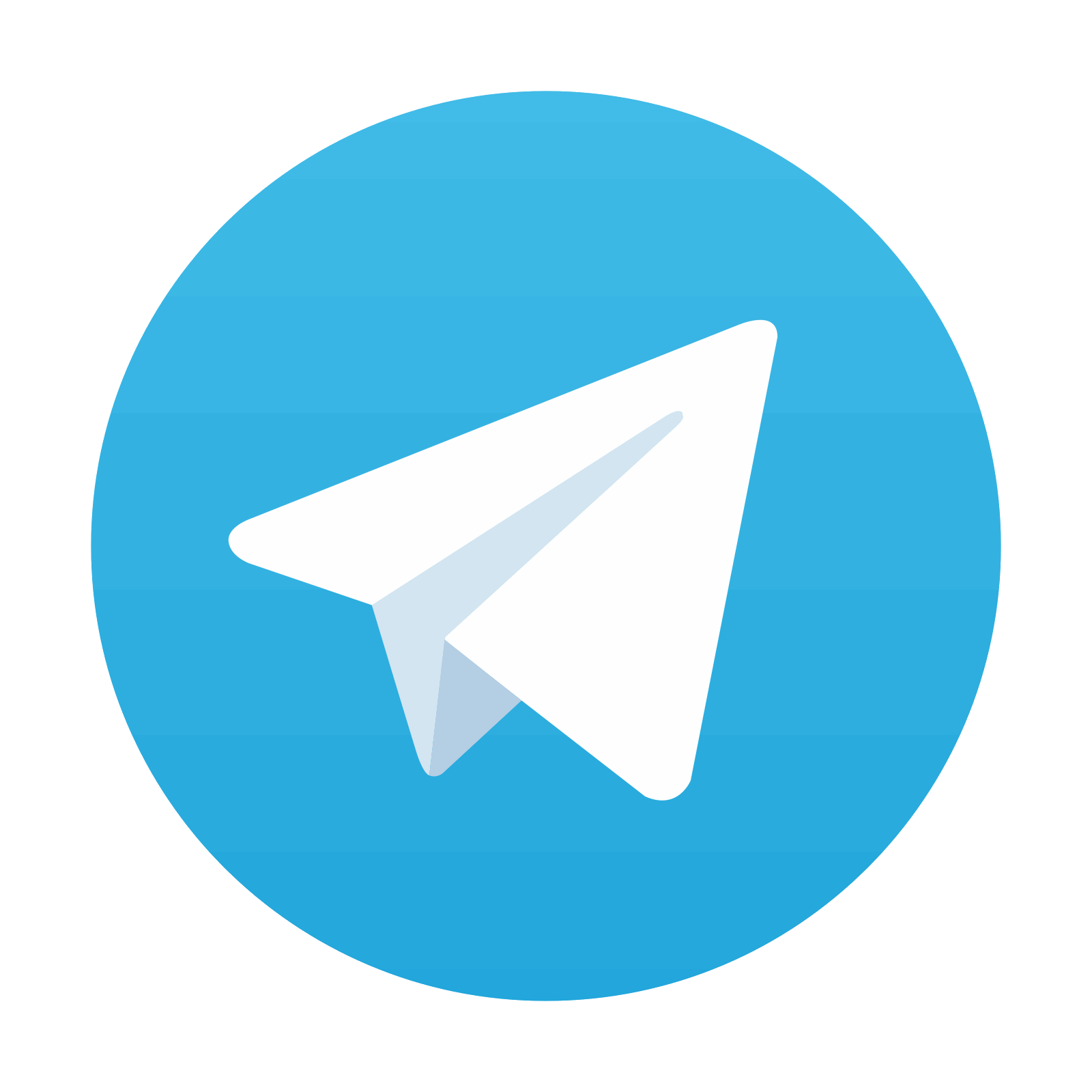
Stay updated, free articles. Join our Telegram channel

Full access? Get Clinical Tree
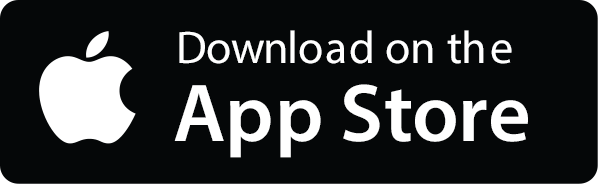
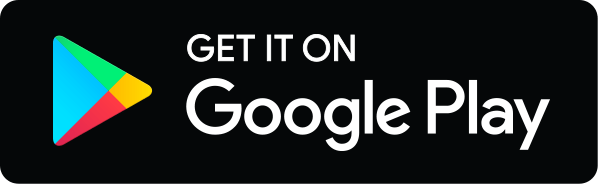