The frequency and importance of the evaluation of the posterior fossa have increased significantly over the past 20 years owing to advances in neuroimaging. Conventional and advanced neuroimaging techniques allow detailed evaluation of the complex anatomic structures within the posterior fossa. A wide spectrum of cerebellar and brainstem malformations has been shown. Familiarity with the spectrum of cerebellar and brainstem malformations and their well-defined diagnostic criteria is crucial for optimal therapy, an accurate prognosis, and correct genetic counseling. This article discusses cerebellar and brainstem malformations, with emphasis on neuroimaging findings (including diagnostic criteria), neurologic presentation, systemic involvement, prognosis, and recurrence.
Key points
- •
Progress in neuroimaging and genetics in the last decades has led to a significant improvement/refinement in the definition of cerebellar and brainstem malformations.
- •
Neuroimaging plays a key role in the diagnostic work-up of children with cerebellar and brainstem malformations.
- •
Diagnostic criteria of cerebellar and brainstem malformations are mostly based on neuroimaging findings.
- •
Neuroimaging findings may elucidate the role of the cerebellum for neurocognitive functions in children with cerebellar malformations and serve as predictive biomarkers for cognitive outcome.
- •
Advanced neuroimaging techniques such as diffusion tensor imaging may provide additional information that is helpful to better understand the pathogenesis of selected cerebellar and brainstem malformations.
Introduction
In the last few decades, progress in neuroimaging techniques, genetic analysis, and mouse model research has led to a significant improvement in the definition of cerebellar and brainstem malformations as well as in the recognition of novel disorders. Classifications based on neuroimaging, molecular genetic criteria, and developmental biological criteria have been proposed and include both inherited (developmental) and acquired (disruptive) anomalies.
Malformations are defined as nonprogressive, congenital morphologic anomalies of a single organ or body part caused by an alteration of the primary developmental program. Malformations result from intrinsic developmental processes, which refer to the cellular and molecular pathways involved in organogenesis. The molecules in these pathways can be altered by gene mutations, teratogens, or combined effects. The complex development of the cerebellum and brainstem and the high number of involved genes result in a high number of malformations.
Neuroimaging plays a key role in the diagnostic work-up of posterior fossa malformations. Diagnostic criteria for posterior fossa malformations are based on neuroimaging findings. For some posterior fossa malformations, the spectrum of neuroimaging findings has been shown to explain the cognitive outcome (eg, in Dandy-Walker malformation [DWM] and rhombencephalosynapsis). In addition, the spectrum of neuroimaging findings may suggest the underlying genotype (eg, in pontocerebellar hypoplasias [PCHs]). Conventional magnetic resonance (MR) imaging, including two-dimensional and three-dimensional T1-weighted, T2-weighted, and fluid attenuated inversion recovery (FLAIR) sequences, plays a key role in the evaluation/characterization of posterior fossa malformations and many diagnostic criteria have been based on conventional MR imaging findings. In the last decade, advanced MR imaging techniques became increasingly available in the clinical setting and have been applied to explore posterior fossa malformations in more detail. Diffusion tensor imaging (DTI) has been shown to provide noninvasive detailed qualitative and quantitative information on white matter tracts in children with brain malformations. In addition, the detailed internal neuroarchitectural exploration of the brain by DTI has allowed clinicians to elucidate certain aspects of the pathogenesis of selected posterior fossa malformations, such as Joubert syndrome (JS) and pontine tegmental cap dysplasia (PTCD).
This article discusses the normal anatomy of the posterior fossa followed by a discussion of the characteristic neuroimaging features of a variety of cerebellar and brainstem malformations. In this context, we classify posterior fossa malformations based on the neuroimaging pattern into (1) predominantly cerebellar, (2) cerebellar and brainstem, and (3) predominantly brainstem malformations as previously suggested.
Introduction
In the last few decades, progress in neuroimaging techniques, genetic analysis, and mouse model research has led to a significant improvement in the definition of cerebellar and brainstem malformations as well as in the recognition of novel disorders. Classifications based on neuroimaging, molecular genetic criteria, and developmental biological criteria have been proposed and include both inherited (developmental) and acquired (disruptive) anomalies.
Malformations are defined as nonprogressive, congenital morphologic anomalies of a single organ or body part caused by an alteration of the primary developmental program. Malformations result from intrinsic developmental processes, which refer to the cellular and molecular pathways involved in organogenesis. The molecules in these pathways can be altered by gene mutations, teratogens, or combined effects. The complex development of the cerebellum and brainstem and the high number of involved genes result in a high number of malformations.
Neuroimaging plays a key role in the diagnostic work-up of posterior fossa malformations. Diagnostic criteria for posterior fossa malformations are based on neuroimaging findings. For some posterior fossa malformations, the spectrum of neuroimaging findings has been shown to explain the cognitive outcome (eg, in Dandy-Walker malformation [DWM] and rhombencephalosynapsis). In addition, the spectrum of neuroimaging findings may suggest the underlying genotype (eg, in pontocerebellar hypoplasias [PCHs]). Conventional magnetic resonance (MR) imaging, including two-dimensional and three-dimensional T1-weighted, T2-weighted, and fluid attenuated inversion recovery (FLAIR) sequences, plays a key role in the evaluation/characterization of posterior fossa malformations and many diagnostic criteria have been based on conventional MR imaging findings. In the last decade, advanced MR imaging techniques became increasingly available in the clinical setting and have been applied to explore posterior fossa malformations in more detail. Diffusion tensor imaging (DTI) has been shown to provide noninvasive detailed qualitative and quantitative information on white matter tracts in children with brain malformations. In addition, the detailed internal neuroarchitectural exploration of the brain by DTI has allowed clinicians to elucidate certain aspects of the pathogenesis of selected posterior fossa malformations, such as Joubert syndrome (JS) and pontine tegmental cap dysplasia (PTCD).
This article discusses the normal anatomy of the posterior fossa followed by a discussion of the characteristic neuroimaging features of a variety of cerebellar and brainstem malformations. In this context, we classify posterior fossa malformations based on the neuroimaging pattern into (1) predominantly cerebellar, (2) cerebellar and brainstem, and (3) predominantly brainstem malformations as previously suggested.
Normal anatomy of the posterior fossa
Conventional MR imaging sequences allow detailed evaluation of the anatomy of the posterior fossa and its contents. A midline sagittal T1-weighted or T2-weighted sequence is ideal for showing the size of the posterior fossa, the shape and size of the vermis, and the size and morphology of the fourth ventricle and brainstem ( Fig. 1 ). The vermis is divided into 3 parts by the primary and prepyramidal fissures. The rostrocaudal length of the ventral pons should be approximately twice that of the midbrain from the isthmus (ventral midbrain-pons junction) to the third ventricle, whereas the rostrocaudal length of the midbrain should be roughly the same as that of the medulla (from the level of the obex to the level of the ventral pontomedullary junction). The posterior margin of the brainstem extending from the caudal sylvian aqueduct to the obex should be a straight line. The fastigium, or summit of the fourth ventricle, should lie just below the midpoint of the ventral pons on sagittal images. The cerebellar hemispheres and peduncles can be well assessed on parasagittal images, whereas the size and morphology of the vermis, cerebellar hemispheres, dentate nuclei, and superior and middle cerebellar peduncles can be best evaluated on axial images. In addition, the cerebellar folia run parallel to the calvaria (onionlike configuration) (see Fig. 1 ). Coronal images show fissures radiating toward the cerebellar nuclei. In addition, the superior, middle, and inferior cerebellar peduncles can be assessed in terms of size, symmetry, contour, and location.
Predominantly cerebellar malformations
Dandy-Walker Malformation
DWM is the most common posterior fossa malformation, and occurs mainly sporadically, with a low overall risk of recurrence (1%–5%). DWM may be isolated or may be part of numerous chromosomal anomalies or well-defined mendelian disorders. Recently, genetic studies suggested that mutations resulting in dysgenesis of both the cerebellum and its overlying mesenchyme are likely to be needed to cause a DWM.
Most children with DWM present before 1 year of age with signs and symptoms of increased intracranial pressure. Macrocephaly is the most common manifestation, affecting 90% to 100% of children during the first months of life. Nowadays the diagnosis of DWM is made by fetal MR imaging in an increasing number of children.
The key neuroimaging features of DWM are (1) hypoplasia (or, rarely, agenesis) of the cerebellar vermis (of which the inferior portion is typically affected, possibly in combination with its superior portion), which is elevated and counterclockwise rotated; and (2) dilatation of the cystic-appearing fourth ventricle, which may fill most of the posterior fossa ( Fig. 2 ). The cerebellar hemispheres are typically hypoplastic and displaced anterolaterally. The posterior fossa is usually enlarged, and the tentorium, the torcular Herophili, and transverse sinuses are elevated. Additional malformations, including callosal dysgenesis or agenesis, occipital encephalocele, polymicrogyria, and heterotopia, are present in 30% to 50% of patients. Hydrocephalus is associated with DWM in about 90% of children.
Neuroimaging allows the differentiation of DWM from other cystic posterior fossa malformations, such as Blake pouch cyst (BPC), posterior fossa arachnoid cyst (PFAC), and mega cisterna magna (MCM) ( Fig. 3 , Table 1 ). BPC is characterized by a retrocerebellar or infraretrocerebellar cyst that directly communicates with the fourth ventricle, likely secondary to a deficient opening of the foramen of Magendie during development. The choroid plexus is displaced inferior to the vermis, and is best visualized in a sagittal T1-weighted postcontrast image. The absence of communication between the fourth ventricle and subarachnoid space results in a tetraventricular hydrocephalus. Mild mass effect may cause indentation of the inferior vermis and cerebellar hemispheres, but these are globally normal. PFAC may be located inferior or posterior to the vermis (retrocerebellar), cranial to the vermis (supravermian), anterior or lateral to the cerebellar hemispheres, or anterior to the brainstem. Neuroimaging shows a well-circumscribed extra-axial fluid collection, which is isointense to cerebrospinal fluid (CSF) on T2-weighted imaging, may have a slightly higher signal on FLAIR imaging or apparent diffusion coefficient maps because of a higher internal protein content, and does not communicate with the fourth ventricle or subarachnoid space. PFAC may enlarge and produce mass effect on the cerebellum causing hydrocephalus. In addition, remodeling, scalloping, or thinning of the overlying occipital bone may be observed. MCM is an enlarged cisterna magna (≥10-mm diameter on midsagittal images) with an intact vermis and normal fourth ventricle. MCM freely communicates with the fourth ventricle and cervical subarachnoid space, and consequently hydrocephalus is consistently absent. MCM is mostly an incidental finding without clinical relevance.
Disease | Vermis Size | IV. Ventricle Size | Posterior Fossa Size | Hydrocephalus |
---|---|---|---|---|
DWM | Hypoplastic | Enlarged | Enlarged | Yes (most patients) |
BPC | Normal | Enlarged | Normal | Yes |
MCM | Normal | Normal | Inconsistently enlarged | No |
PFAC | Normal | Normal or reduced | Normal | Possible |
Rhombencephalosynapsis
Rhombencephalosynapsis is most likely caused by a dorsoventral patterning defect in the rostral dorsal midline regions of rhombomere 1. The genetic basis is still unknown. The sporadic nature of rhombencephalosynapsis contributes to its low recurrence risk.
Most patients are nonsyndromic and present with truncal and/or limb ataxia, abnormal eye movements, head stereotypies, and delayed motor development. In some patients, rhombencephalosynapsis is a key feature of Gómez-López-Hernández syndrome (parietal alopecia, trigeminal anesthesia, and craniofacial dysmorphic signs) or may be seen in VACTERL (Vertebral anomalies, Anal atresia, Cardiac defects, Tracheoesophageal fistula and/or Esophageal atresia, Renal anomalies, and Limb defects) association. Long-term cognitive outcome varies from severe impairment to normal cognition. Severe rhombencephalosynapsis, associated holoprosencephaly, or VACTERL features are poor prognostic factors. Systemic involvement is uncommon.
The key neuroimaging findings in rhombencephalosynapsis are agenesis or hypogenesis of the vermis and continuity of the cerebellar hemispheres, dentate nuclei, and often superior cerebellar peduncles (SCP) ( Fig. 4 ). The horizontal folial pattern of the cerebellar hemisphere is best seen on posterior coronal T2-weighted images, whereas axial and sagittal images show the dentate nuclei in the midline (in normal anatomy, the dentate nuclei are separated by the vermis and are consequently off midline). In addition, on midsagittal images the primary fissure is not identified. Rhombencephalosynapsis may be associated with other central nervous system anomalies such as hydrocephalus, mostly because of stenosis of the sylvian aqueduct and forebrain abnormalities, including absent olfactory bulbs, callosal dysgenesis, absent septum pellucidum, and, in rare cases, holoprosencephaly.
Cerebellar Dysplasia and Cerebellar Cysts
Cerebellar dysplasia is characterized by abnormal cerebellar foliation and fissuration, white matter arborization, and an abnormal gray-white matter junction. It is a nonspecific feature associated with several different conditions ( Table 2 ) including Chudley-McCullough syndrome, bilateral frontoparietal polymicrogyria caused by GPR56 mutations, Poretti-Boltshauser syndrome caused by LAMA1 mutations, and tubulinopathies. In most patients, however, the cause remains unknown. Multiple mechanisms underlie cerebellar dysplasia, including abnormal precursor cell division and neuronal migration. In particular, alpha-dystroglycanopathies, Poretti-Boltshauser syndrome ( LAMA1 mutations), and GPR56 -associated cerebellar dysplasia are thought to be caused by basement membrane defects that result in abnormal neuronal migration. Chudley-McCullough syndrome is caused by recessive mutations in GPSM2 , which encodes a GTPase regulator needed for correct orientation of stem-cell division. In some patients, cerebellar dysplasia may be focal and located only in 1 cerebellar hemisphere of reduced volume. A prenatal acquired (disruptive) origin is assumed in view of the focal nature and hemorrhages on fetal MR imaging studies.
Disease | Genes | Dysplasia Pattern | Distinguishing Features |
---|---|---|---|
Chudley-McCullough syndrome | GPSM2 | Dysplastic inferior hemispheres | Hearing loss, frontal PMG, heterotopia, partial callosal agenesis |
Alpha-dystroglycanopathies | >15 genes | Global cerebellar dysplasia with multiple cysts | Cobblestone brain, brainstem kinking, muscle and eye involvement, increased creatine kinase level |
GPR56 -related PMG and cerebellar dysplasia | GPR56 | Global cerebellar dysplasia with multiple cysts | Bifrontal PMG, T2/FLAIR hyperintense signal of the supratentorial white matter |
Poretti-Boltshauser syndrome | LAMA1 | Global cerebellar dysplasia with multiple cysts | High myopia, retinal abnormality |
Tubulinopathies | TUBA1A , TUBA8 , TUBB2B , TUBB3 , TUBB5 | Diagonal folia across vermis in axial view | Dysmorphic basal ganglia, cortical malformation (PMG to lissencephaly), callosal dysgenesis, asymmetric brainstem |
JS | >30 genes | Dysplastic superior vermis and hemispheres | Molar tooth sign |
The clinical presentation is highly variable and mostly depends on the underlying disease. Isolated cerebellar dysplasia may be an incidental finding or cause severe neurologic impairment.
The key neuroimaging findings of cerebellar dysplasia are abnormal foliation, fissuration (including defective, enlarged, or vertical fissures), white matter arborization, and an obscured gray matter–white matter junction ( Fig. 5 ). Cerebellar dysplasia may be associated with a variety of other cerebellar (eg, cerebellar cysts) and supratentorial (eg, polymicrogyria, partial callosal agenesis, and dysmorphic basal ganglia) findings that are usually helpful to differentiate between the causative diseases.
Cerebellar cysts ( Fig. 6 ) are uncommon findings in pediatric neuroimaging and may be seen in selected disorders with both malformative and disruptive causes ( Table 3 ).
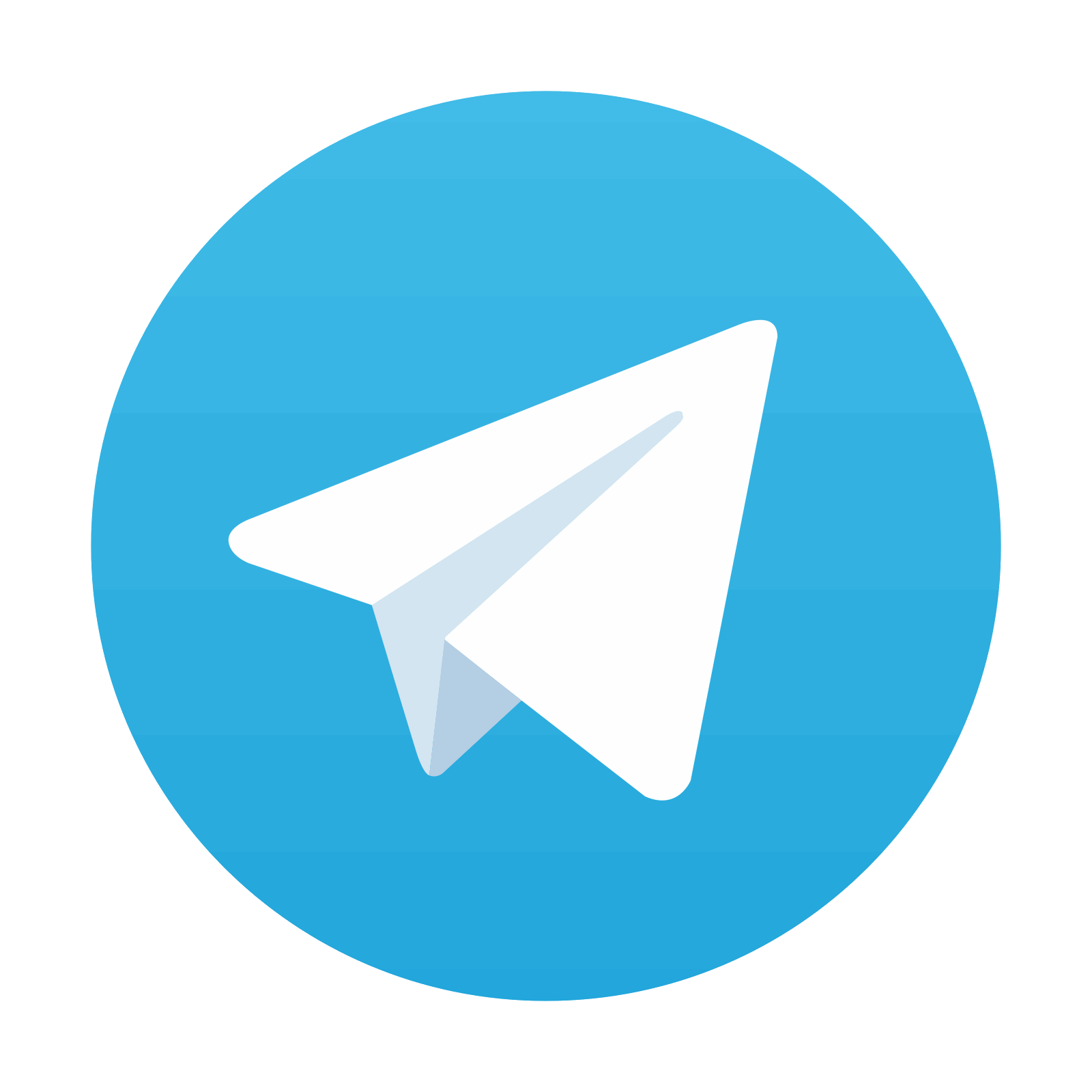
Stay updated, free articles. Join our Telegram channel

Full access? Get Clinical Tree
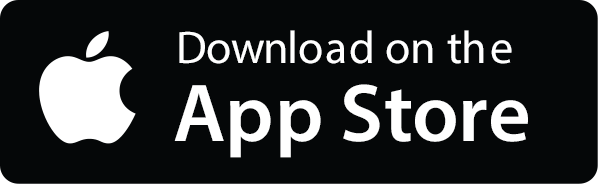
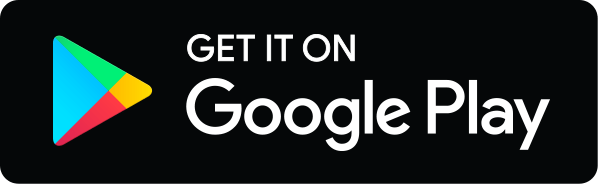
