Significant progress has been made recently in the recognition, screening, diagnosis, and treatment of blunt cerebrovascular vascular injury (BCVI). Although controversy still exists as to optimal screening algorithms and best diagnostic modality, the vital and growing role of noninvasive imaging in identifying patients at high risk for BCVI and in characterizing the injury itself has been clearly established. There has been promising early work in stratifying BCVI patients into risk categories by initially evaluating them with high-resolution head, maxillofacial, and cervical computed tomographic examinations with the ultimate goal of maximizing diagnostic yield and enabling prompt initiation of therapy.
Key points
- •
Significant recent progress has been made in the recognition, screening, diagnosis, and treatment of blunt cerebrovascular injury (BCVI).
- •
Although controversy still exists as to optimal screening algorithms and best diagnostic modality, the vital and growing role of noninvasive imaging in identifying patients at high risk for BCVI and in characterizing the injury itself has been clearly established.
- •
There has been promising early work in stratifying BCVI patients into risk categories by initially evaluating them with high-resolution head, maxillofacial, and cervical computed tomographic (CT) examinations with the ultimate goal of maximizing diagnostic yield and enabling prompt initiation of therapy.
- •
Further work is needed to delineate the mechanistic relationship between craniofacial fractures and BCVI.
- •
Recent studies indicate the incidence of BCVI may be much higher (1%–3%) than initially reported (0.1%), due to the wider utilization of aggressive screening algorithms and noninvasive imaging.
- •
A high index of suspicion is necessary to identify BCVI, since many patients exhibit a latent, asymptomatic period.
- •
Untreated BCVI is associated with high morbidity and mortality. Identification and treatment of patients while they are asymptomatic has been shown to improve outcomes.
- •
CT angiography is the study of choice for initial imaging of traumatic CVI, although magnetic resonance imaging/magnetic resonance angiography demonstrates considerable value in characterizing vessel injury as well as associated ischemic complications.
- •
Current screening algorithms reinforce the importance of high-resolution head, maxillofacial, and cervical spine CT in identifying patients at high risk for BCVI.
Introduction
Historical Perspective and Significance of Traumatic Blunt Cerebrovascular Injury
The recognition of blunt cerebrovascular injury (BCVI) as an important diagnostic entity has occurred only in the past 2 decades, with continued current debate as to best practices in regards to screening, diagnosis, treatment, and follow-up.
The true incidence of BCVI in the setting of trauma is still not known but has been greatly underestimated in the past, largely because of a lack of routine imaging of asymptomatic patients. Before 1990, less than 200 total blunt carotid artery injury (BCAI) cases had been described in the literature. Regionalization of trauma care caused these “uncommon” injuries to be funneled into fewer referral centers, generating greater interest in improving diagnosis. Many studies before the mid 1990s reported a 0.1% overall incidence of blunt injury to the carotid artery in trauma victims. With subsequent utilization of aggressive screening criteria, however, the incidence of documented cerebrovascular injury in blunt trauma patients increased 10-fold to 1%, and even higher (2.7%) when applied to patients with Injury Severity Scores of greater than or equal to 16. Although initial emphasis was placed on carotid arterial injury (CAI), the incidence of vertebral artery injuries (VAI) from blunt trauma was found to range from 0.53% to 0.73%.
Despite the relative infrequency of BCVI, devastating complications are very common in patients with documented injuries ( Fig. 1 ). A 1998 review of the literature reported BCAI mortalities of 23% to 28%, with even higher rates of permanent neurologic deficit (48%–58%). Similarly, a mortality of 8% and permanent morbidity of 14% to 24% have been reported in untreated patients with blunt VAI. Over the past decade, a growing body of evidence has revealed that a significant percentage of BCVI patients present in a delayed fashion, with ischemic events following a latent asymptomatic period. Antithrombotic medical therapy has been recently shown to decrease the incidence of posttraumatic stroke significantly and improve final neurologic outcome, emphasizing the importance of early diagnosis. As a result, aggressive screening protocols have been instituted, with an emphasis on utilization of noninvasive imaging modalities, such as computed tomographic angiography (CTA) and magnetic resonance angiography (MRA).
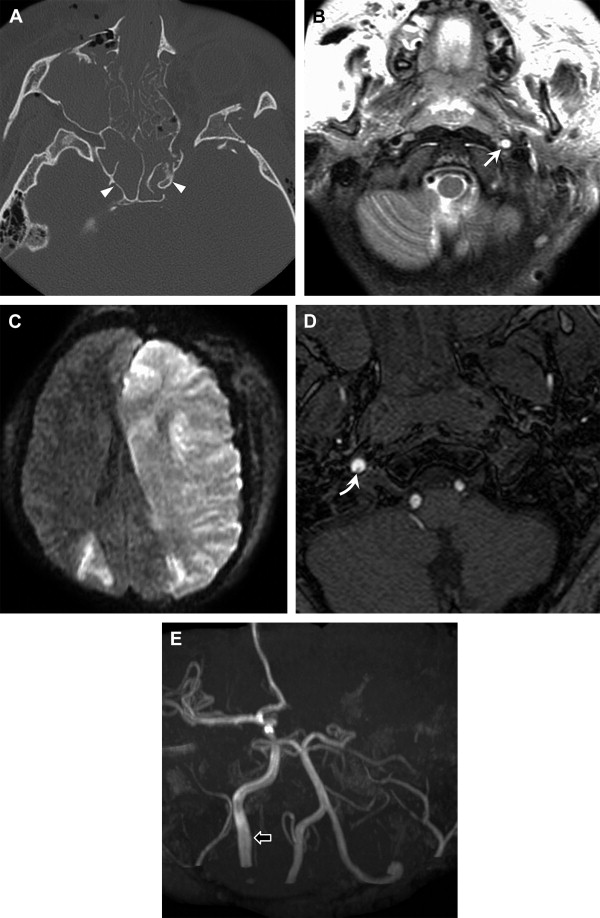
Introduction
Historical Perspective and Significance of Traumatic Blunt Cerebrovascular Injury
The recognition of blunt cerebrovascular injury (BCVI) as an important diagnostic entity has occurred only in the past 2 decades, with continued current debate as to best practices in regards to screening, diagnosis, treatment, and follow-up.
The true incidence of BCVI in the setting of trauma is still not known but has been greatly underestimated in the past, largely because of a lack of routine imaging of asymptomatic patients. Before 1990, less than 200 total blunt carotid artery injury (BCAI) cases had been described in the literature. Regionalization of trauma care caused these “uncommon” injuries to be funneled into fewer referral centers, generating greater interest in improving diagnosis. Many studies before the mid 1990s reported a 0.1% overall incidence of blunt injury to the carotid artery in trauma victims. With subsequent utilization of aggressive screening criteria, however, the incidence of documented cerebrovascular injury in blunt trauma patients increased 10-fold to 1%, and even higher (2.7%) when applied to patients with Injury Severity Scores of greater than or equal to 16. Although initial emphasis was placed on carotid arterial injury (CAI), the incidence of vertebral artery injuries (VAI) from blunt trauma was found to range from 0.53% to 0.73%.
Despite the relative infrequency of BCVI, devastating complications are very common in patients with documented injuries ( Fig. 1 ). A 1998 review of the literature reported BCAI mortalities of 23% to 28%, with even higher rates of permanent neurologic deficit (48%–58%). Similarly, a mortality of 8% and permanent morbidity of 14% to 24% have been reported in untreated patients with blunt VAI. Over the past decade, a growing body of evidence has revealed that a significant percentage of BCVI patients present in a delayed fashion, with ischemic events following a latent asymptomatic period. Antithrombotic medical therapy has been recently shown to decrease the incidence of posttraumatic stroke significantly and improve final neurologic outcome, emphasizing the importance of early diagnosis. As a result, aggressive screening protocols have been instituted, with an emphasis on utilization of noninvasive imaging modalities, such as computed tomographic angiography (CTA) and magnetic resonance angiography (MRA).
Anatomy and pathology
Mechanisms and Patterns of Cerebrovascular Injury
Commonly accepted physiologic mechanisms of traumatic cerebrovascular injury include extreme cervical hyperextension/rotation, direct blunt vascular trauma, intraoral trauma, and direct laceration from bony fracture fragments. Traumatic cerebrovascular dissections typically result from rapid deceleration of the body and resultant stretching of the involved vessel. This mechanism can be seen in patients following motor vehicle accidents, assault, pedestrian accidents, falls, and with hanging accidents. Although consistently implicated as a risk factor for BCVI, the mechanisms associated with craniofacial fractures are not as well delineated.
The extracranial segments of the carotid and vertebral arteries are more vulnerable to traumatic injury than the intracranial segments because of their close relationship to surrounding osseous structures and relative greater mobility. Extracranial carotid artery injuries most commonly occur in the distal cervical internal carotid artery (ICA) ( Figs. 2 and 3 ). Injury is thought to result from stretching over the lateral masses of the cervical vertebrae (particularly C1-3) in the setting of head hyperextension and contralateral hyperrotation, and from impingement on the styloid process during head rotation. The ICA may also be compressed between the mandible or hyoid bone and the cervical spine during neck hyperflexion. Prior studies have reported that superior displacement of the pterygoid plates (as in the case of Le Fort type fractures) poses a risk to the ICA inferior to the foramen lacerum. Displaced bony fragments from skull base fractures may also lead to direct injury of the ICA.
Extracranial VAI most commonly involves the V2 and V3 segments, because the vessel travels through the bony transverse foramina and around C1, respectively ( Figs. 4 and 5 ). Displaced fracture fragments of the cervical spine may directly lacerate these segments of the vertebral arteries. Injuries to the V3 and V4 segments occur more commonly without associated cervical spine fracture/dislocation than injuries to the V2 segment.
Although there is a relative dearth of data on incidence, it is generally accepted that intracranial cerebrovascular injury is less common than extracranial BCVI. Basilar skull fracture, certain patterns of facial fracture, and fractures extending through the carotid canal have been reported as risk factors for intracranial arterial injury ( Figs. 6 and 7 ). Manifestation of injury includes vascular compression, dissection, dissecting aneurysm, occlusion, arterial rupture, and arteriovenous fistula (carotid-cavernous).
Pathophysiology of BCVI
Different mechanisms of carotid and VAI contribute to a varied appearance on imaging. Blunt cerebrovascular dissection usually begins with a trauma-induced intimal tear or primary intramural hematoma. With intimal injury, exposed subendothelial collagen initiates platelet aggregation to form thrombus ( Figs. 8 and 9 ), which may produce vessel stenosis or occlusion or result in distal embolization (see Fig. 8 ). A dissecting hematoma within the media may propagate cranially to narrow or occlude the vessel ( Figs. 10 and 11 ), or focally expand the adventitia to form a traumatic dissecting aneurysm (also referred to as “pseudoaneurysm”).
Imaging
Imaging Findings of BCVI
It is important to be familiar with the spectrum of findings and imaging pitfalls associated with the diagnosis of vascular injury on CTA, magnetic resonance (MR) imaging, MRA, and conventional angiography. Ultrasound imaging plays a limited role in diagnosis of cerebrovascular injury (see Fig. 8 ), because of its poor detection of specific signs of BCVI lesions in the depth of the neck, where many ICA and VAI occur.
In those patients at risk for BCVI, imaging of the entire cerebrovascular system should be performed from the aortic arch through the circle of Willis, as vessel injury may be remote from other signs of trauma. Another important imaging principle in the assessment for BCVI is to be aware of the high rate of multiple lesions. Several series have reported that up to 22% to 43% of injuries are bilateral ( Figs. 12 and 13 ).
Digital subtraction angiography (DSA) has been considered the gold standard for diagnostic evaluation of BCVI for many years (see Figs. 2 and 13 ). It is limited, however, by an inability to characterize the thickness and configuration of the arterial wall, the requirement to transport the patient outside the emergency department, its invasive nature, and risk of procedural complications. The classic angiographic finding of dissection is an eccentric, long segment, tapered stenosis (“string sign”) often associated with intimal irregularity (see Fig. 13 ). Focal narrowing with a more distal site of dilatation (“string-and-pearl sign”) (see Fig. 13 ) can also be present. Tapered stenosis with a concomitant dissecting aneurysm, occlusion, and isolated dissecting aneurysm are the most common imaging findings, in that order. Pathognomonic imaging signs on DSA, such as intimal flap or a double lumen, are seen less commonly.
CTA provides the advantage of both high-spatial and high-contrast resolution of the arterial wall and lumen (see Figs. 2–9 ). In contradistinction to the 2 projections typically obtained with conventional angiography, CTA allows profiling of the entire 360° circumference of the arterial lumen, increasing sensitivity for detection of minor vessel injury. Inclusion of an unenhanced head CT is an essential component of a CTA protocol, to evaluate for associated intracranial hemorrhage and/or ischemia. These nonenhanced images may occasionally demonstrate injuries of the distal segments of injured carotid and vertebral arteries. Dissecting intramural hematomas can manifest on unenhanced CT as a hyperdense crescent-shaped mural lesion, often visualized near the skull base. On CTA, the same pathologic abnormality will be seen as luminal narrowing caused by crescentic intramural hematoma, which is usually isodense to muscle. Because this may be difficult to distinguish from atherosclerotic disease, recognizing that dissection will typically spare the carotid bulb is essential to making the correct diagnosis. Often the intramural hematoma causes overall enlargement of the external vessel diameter, despite narrowing of the lumen (see Figs. 2 and 3 ). Other reliable signs of dissection on CTA include the identification of an intimal flap or dissecting aneurysm (see Figs. 2 , 6 , and 9 ). Multiplanar 2-dimensional (2D), curved planar 2D, and 3-dimensional (3D) reformations can be obtained to create images that are comparable to those seen with conventional angiography. Although these reformations are complementary, it is absolutely essential to evaluate the thin-section axial CT source images systematically for signs of vascular injury, as it may be obscured on 2D reconstructed images.
The MR imaging appearance of dissection is highly dependent on the age of the intramural hematoma, the surrounding tissues, and MR imaging sequences used for evaluation. The MR imaging appearance of the hematoma will follow the known age-dependent signal intensity of paramagnetic iron (see Figs. 1 , 3 , 10 , and 11 ). The intramural hematoma is usually most apparent in the subacute stage. Subacute hematomas (containing methemoglobin) demonstrate characteristic findings on fat-suppressed T1-weighted images (see Fig. 10 ). The intramural hematoma will be seen as a high-intensity crescentic lesion adjacent to an eccentric flow void, which represents the residual lumen. The subacute intramural hematoma, with its short T1 values, will also be evident on noncontrast time-of-flight (TOF) MRA and can be mistaken for flow on these images (see Fig. 10 ). Phase-contrast and contrast-enhanced MRA will more clearly differentiate flow from the adjacent intramural hematoma. The intramural hematoma often causes overall enlargement of the external vessel diameter (see Figs. 3 and 10 ). Important pitfalls of MR imaging include the relative isointense appearance of acute (<7 days) and chronic (>2 months) hematoma on T1-weighted imaging, which blends in with surrounding tissues with fat suppression. Dephasing and signal dropout on TOF images caused by turbulent flow in the horizontal petrous segment of the ICA can mimic intraluminal thrombus or dissection. Signal loss from in-plane flow or slab artifact on TOF imaging can also result in poor signal in horizontal segments of carotid or vertebral artery branches. Systematic evaluation of source images on TOF or contrast-enhanced MRA images is essential, to avoid missing subtle injury that may not be as apparent on reformations.
Color Duplex ultrasound has a limited role in the evaluation of BCVI patients, as 90% of traumatic lesions occur in acoustically nonassessable segments of the carotid and vertebral arteries. The cephalad parts of the extracranial ICA and VA are difficult to image, requiring use of low-frequency sector transducers and reliance mainly on hemodynamic abnormalities for diagnosis of dissection. Most dissecting aneurysms are missed. That said, specific signs of dissection may be detected in the more accessible proximal ICA, with mural hematoma manifesting as a thickened hypoechoic vessel wall on B-mode or high-frequency sector transducers. Intimal flaps and double lumens are occasionally depicted (see Fig. 8 ).
BCVI Classification
Traumatic cerebrovascular injuries can be classified by the location of injury (intracranial vs extracranial) and/or the extent of vessel wall involvement. The mildest form of injury is merely extrinsic compression of the lumen by extramural hematoma, ( Fig. 14 ) without a true tear of the vessel wall. True vascular tears may affect just the intima, both the intima and the media, or may extend through the entire thickness of the vessel wall. The greater the extent of wall disruption, the more abnormal the vessel configuration will appear on imaging studies ( Figs. 15–20 ).
