Historical Overview
Bronchopulmonary dysplasia (BPD) or chronic lung disease of the premature comprises a heterogeneous group of respiratory diseases of infancy that usually evolve from an acute respiratory disorder experienced by a newborn. Chronic lung disease of the premature most commonly occurs in infants with birth weights less than 1500 g, and especially in infants with birth weights less than 1000 g and who are treated for respiratory distress syndrome (RDS). This entity was first described by Northway and colleagues in 1967 in premature infants who had severe RDS and required prolonged mechanical ventilation with high inflation pressures and inspired oxygen concentrations. The pathology was characterized by abnormalities of the terminal airways, the hallmark of which was an intense interstitial fibrosis and hyperplasia of the smooth muscle ( Fig. 1-1 ). Affected infants experienced chronic respiratory failure, with hypoxemia and hypercapnia, and many developed cor pulmonale.
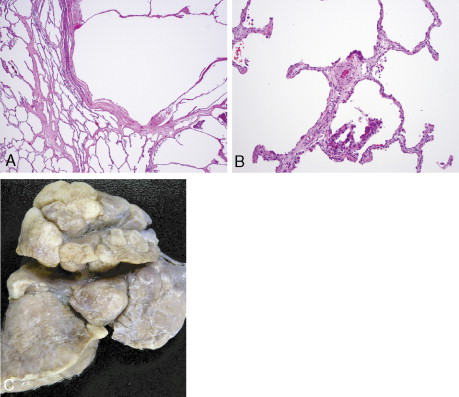
More recently, as the use of antenatal steroids and surfactant replacement therapy has resulted in increased survival of smaller preterm infants, the features of the so-called new BPD differ from the older descriptions and include milder respiratory distress that often requires minimal ventilatory support with low fractions of inspired oxygen (FiO 2 ) preceding the chronic condition. Pathologic examination typically shows an arrest in pulmonary development and alveolarization, with simplification of the terminal airways ( Fig. 1-2 ).
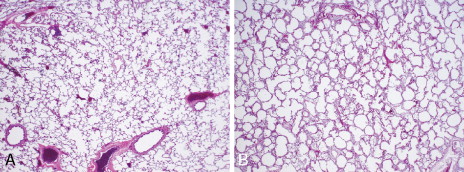
Definitions of Bronchopulmonary Dysplasia
The terminology used to describe chronic lung disease arising from neonatal insults is confusing. The terms “bronchopulmonary dysplasia” (BPD) and “chronic lung disease of infancy” (CLDI) are sometimes used interchangeably to describe chronic respiratory disease after treatment for RDS in preterm infants. A working definition of BPD is necessary because it is from BPD that most cases of CLDI arise. Three clinical definitions have been used to define BPD in neonates, as follows:
- •
Oxygen requirement at 28 days postnatal age
- •
Oxygen requirement at 36 weeks postmenstrual age (PMA)
- •
Diagnostic criteria proposed by a National Institute of Child Health and Human Development (NICHD) workshop based on gestational age and disease severity
In the 1990s, several studies showed that administration of supplemental oxygen at 36 weeks PMA rather than 28 days postnatal age more accurately predicted abnormal pulmonary outcome at 2 years of age. In one study, the positive predictive value for abnormal outcome in very-low-birth-weight (VLBW) infants (birth weight <1,500 g) (63% versus 38%) was better for supplemental oxygen administration at 36 weeks PMA than at 28 postnatal days. Outcome was normal in 90% of infants who did not receive oxygen at 36 weeks PMA. As a result, the definition of BPD as requiring administration of supplemental oxygen at 36 weeks PMA became widely used.
A precise definition of BPD is especially important when outcomes are compared among different centers or when new therapeutic interventions are tested. The increased survival of extremely-low-birth-weight (ELBW) infants (birth weight <1,000 g or gestational age <30 weeks) suggested that the definition of BPD could be refined to include infants with milder disease and account for developmental changes that occur with increasing gestational age. In 2001, the NICHD workshop proposed diagnostic criteria for BPD that included gestational age and disease severity ( Table 1-1 ). This scheme divides patients into the following two groups based on gestational age, which determines the timing of clinical assessment for BPD:
- •
Patients who are less than 32 weeks gestation are assessed at 36 weeks PMA or when discharged home, whichever comes first.
- •
Patients who are equal to or greater than 32 weeks gestation are assessed at 29 to 55 days of life or when discharged home, whichever comes first.
GESTATIONAL AGE <32 WEEKS PMA | GESTATIONAL AGE ≥32 WEEKS PMA | |
---|---|---|
Time of assessment | 36 wk PMA or discharge to home * | >28 days but <56 days postnatal age or discharge to home * |
Mild CLD | Treatment with oxygen >21% for at least 28 days plus | Breathing room air by 56 days postnatal age or discharge * |
Breathing room air at 36 wk PMA or discharge * | ||
Moderate CLD | Need † for FiO 2 <30% at 36 wk PMA or discharge * | Need † for FiO 2 <30% at 56 days postnatal age or discharge * |
Severe CLD | Need † for FiO 2 ≥30% and/or positive pressure (IPPV or NCPAP) at 36 wk PMA or discharge * | Need † for FiO 2 ≥30% and/or positive pressure (IPPV or NCPAP) at 56 days postnatal age or discharge * |
† At the time of the workshop, a physiologic test to confirm oxygen requirement had yet to be defined.
At the time of assessment, patients are evaluated for the severity of their disease. Infants who received treatment with supplemental oxygen for at least 28 postnatal days are classified as having mild, moderate, or severe BPD, depending on the extent of oxygen supplementation and other respiratory support.
In a study from the NICHD Neonatal Research Network of infants with BPD who were born at less than 32 weeks gestational age and with birth weight less than 1000 g, these criteria predicted pulmonary and neurodevelopmental outcomes at 18 to 22 months corrected age. The severity of BPD (mild, moderate, severe) was associated with use of pulmonary medications (30%, 41%, 47%) and rehospitalization for pulmonary disease (27%, 34%, 40%). The incidence of any neurodevelopmental impairment, including cerebral palsy, blindness, hearing deficit requiring amplification, and lower mental and psychomotor development index scores, also increased with the severity of BPD. The patients who had severe BPD often had substantial associated morbidity during their hospitalization at birth, such as severe intraventricular hemorrhage (25%), periventricular leukomalacia (10%), necrotizing enterocolitis (14%), late-onset infection (54%), and postdischarge deaths (5%), and were often treated with postnatal corticosteroids (78%). In this cohort, many infants who needed oxygen during the first 28 days of life no longer required oxygen at 36 weeks PMA and might not have been classified as having BPD. This study did not include an objective physiologic measure of oxygen requirement, however. These criteria, especially with the addition of a physiologic test, may improve the ability to compare therapeutic interventions in clinical trials and evaluate long-term outcomes.
In most neonatal intensive care units (NICUs), supplemental oxygen is adjusted to maintain the infant’s oxygen saturation within a target range. As a result, the use of supplemental oxygen depends partly on the NICU policy for the target range, which varies among centers. To standardize the use of supplemental oxygen, the NICHD workshop also proposed that the need for oxygen less than or greater than 30% be confirmed by a physiologic test. Such a test was developed, based on oxygen administration and oxygen saturation, including a timed room air challenge in selected patients. It was found to be safe, feasible, and reliable. In a prospective multicenter study of VLBW infants who remained hospitalized at 36 weeks PMA, fewer infants had BPD when the physiologic definition was used (25% versus 35%) compared with the clinical definition (oxygen supplementation at 36 weeks PMA), and there was less variation among centers.
Epidemiology
The rate of BPD varies among institutions. In a report from the NICHD Neonatal Research Network (1995 to 1996), the rate of BPD ranged from 3% to 43% in the 14 participating centers. Variability in incidence among centers may reflect neonatal risk factors or care practices, such as target levels for acceptable oxygen saturation. The overall incidence of BPD was 23% of the VLBW infants, and increased with decreasing birth weight. Compared with the entire cohort, there were increased proportions of males with BPD and severe BPD (54% for entire cohort, 60% for males with BPD, and 67% for males with severe BPD).
In an 8-year (1994 to 2002) retrospective cohort study of six NICUs, the overall incidence of BPD remained constant at 12% for preterm infants born before 33 weeks gestation. Although there has been no change in the overall incidence of BPD, there has been a significant decline in the incidence of severe BPD, from 10% in 1994 to 4% in 2002, with severe BPD defined as requiring positive-pressure respiratory support (i.e., mechanical ventilation or continuous positive airway pressure [CPAP]) at 36 weeks PMA. The risk for any degree BPD increases with mechanical ventilation, as described in a case-cohort study conducted at two centers (two Boston hospitals and Babies’ and Children’s Hospital in New York), in which the higher rate of BPD in Boston (22% versus 4%) reflected the higher rate of mechanical ventilation (75% versus 29%) and surfactant administration (45% versus 10%) as part of the initial respiratory management of VLBW infants. The risk of BPD increases with decreasing birth weight. BPD is rare in infants older than 32 weeks gestation.
Pathogenesis
The etiology of BPD is multifactorial. Inflammation caused by mechanical ventilation, oxygen toxicity, or infection plays an important role. The lung seems to be most vulnerable before the saccular stage of development, which occurs at approximately 31 to 34 weeks gestation, and during which alveolar formation is initiated. The preterm lung is especially at risk of injury because of its structural and functional immaturity. Lungs in preterm infants have poorly developed airway supporting structures, surfactant deficiency, decreased compliance, underdeveloped antioxidant mechanisms, and inadequate fluid clearance compared with term infants.
Prenatal Events
Many events that occur before birth affect the development of the lung. In infants less than 27 weeks gestation—the period of highest risk for development of BPD—the human lung is in the saccular stage of development; alveolarization begins at approximately 32 weeks and proceeds through 40 weeks PMA. Any factor that has an adverse effect on this process leads to disruption of lung architecture and alveolar simplification, a hallmark of the “new BPD.”
Inflammation, as discussed later, is considered one of the central aspects in the pathogenesis of BPD. Several studies suggest that the timing, the type, and the intensity of the inflammatory response that determine the subsequent effects on the developing preterm lung. A series of studies in fetal rabbits and fetal sheep showed that intra-amniotic exposure to interleukin (IL)-1 or endotoxin accelerated lung maturation and the synthesis of surfactant proteins. In these studies, repeated exposure to proinflammatory agents did not cause progressive inflammation, but rather induced tolerance and suppression of fetal monocyte function. If IL-1 or endotoxin treatment was done with two doses, given 7 days apart, the fetal monocytes reacted in a manner similar to that seen in adult sheep. Concomitant treatment with corticosteroids and IL-1 or endotoxin initially suppressed the inflammatory response, but caused an exaggerated inflammatory response at 5 to 15 days post-treatment. Antenatal exposure to endotoxin and corticosteroids modulates lung injury and maturation in a time-dependent manner.
Hyperoxia and Oxidant Stress
High concentrations of inspired oxygen can damage the lungs, although the exact level or duration of exposure that is unsafe is unknown. In a study conducted in neonatal rats, exposing neonatal rat pups to moderate hyperoxia (FiO 2 0.50) for 15 days caused airway remodeling, hyperreactivity, and inflammatory alterations similar to that seen in BPD. Higher degrees of hyperoxia (FiO 2 0.95) for shorter durations (≤72 hours) result in inhibition of distal airway branching with simplification of architecture in fetal mouse lung explants.
The imbalance between pro-oxidant and antioxidant systems in a preterm neonate in favor of pro-oxidant processes also may contribute to the development of BPD. Preterm infants have few antioxidant defenses and are often exposed to supplemental oxygen to treat pulmonary insufficiency. They also are prone to infection, and many of the proinflammatory cytokines activate the production of reactive oxygen species (ROS), such as superoxide free radical, hydrogen peroxide, hydroxyl free radical, and singlet oxygen, which are produced as the result of oxidative stress. In addition, preterm infants have higher plasma and tissue-free iron concentrations compared with term infants, which can promote the propagation of ROS.
The toxicity of these ROS, particularly in response to exposure to hyperoxia, has been shown in experimental animals; when exposed to equal to or greater than 0.50 FiO 2 , they exhibited irreversible changes in lung growth and DNA synthesis. At the cellular level, ROS cause the uncoupling of respiration from adenosine triphosphate (ATP) synthesis and disruption of the outer mitochondrial membrane. Subsequently, this disruption allows release of pro-apoptotic factors, such as cytochrome- c , apoptosis-inducing factor, and pro-caspases, from the mitochondria into the cytosol, leading to cell death.
Various antioxidant defenses are available in mature human organisms to combat this oxidative stress. They include superoxide dismutase, catalase, glutathione- S -transferase (GST), and glutathione peroxidase, and nutrients such as vitamins A and E, iron, copper, zinc, and selenium, which help prevent oxygen toxicity. Preterm infants have inadequate antioxidant defenses and are at risk of oxygen free radical damage. In some species, antioxidant enzyme concentrations are lower in preterm than term animals, and are poorly induced in response to oxidative stress. Similarly, activities of catalase, glutathione peroxidase, and copper/zinc superoxide dismutase in human cord blood are lower in preterm than in term newborns. In a small study of preterm infants (gestational age 25 to 30 weeks), copper/zinc superoxide dismutase levels were greater in infants who subsequently developed BPD; these infants also had a higher cumulative oxygen exposure. These results suggest that in preterm infants with BPD, superoxide dismutase activity is upregulated and may be a marker for increased oxygen exposure and potentially increased reactive oxygen metabolites.
Mechanical Ventilation and Volutrauma
Lung injury associated with mechanical ventilation contributes to the development of BPD, and it is known that positive-pressure ventilation typically induces bronchiolar lesions. Pulmonary interstitial emphysema is a result of barotrauma and is associated with a high incidence of CLDI.
Disruption of airways may occur early in the course of treatment and may be manifested by increased pulmonary resistance. In one study of ventilated preterm infants in the first 5 days after birth, mean pulmonary resistance was significantly greater in infants who subsequently developed BPD compared with infants who did not.
Animal studies suggest that volutrauma is more important than barotrauma in causing airway injury. Distention of the airways to near-maximum lung volume causes shear injury, capillary leak, and pulmonary edema. In studies in preterm lambs and rabbits and in adult rats, animals given large tidal volume breaths had significantly worse pulmonary mechanics and showed histologic evidence of widespread lung injury. In newborn lambs, large tidal volumes seem to impair the response to subsequent surfactant administration. In one study, preterm lambs ventilated with large tidal volumes (20 mL/kg) showed lower compliance, lower ventilatory efficiency, higher recovery of protein, and lower recovery of surfactant by 6 hours compared with animals ventilated with lower tidal volumes (5 mL/kg and 10 mL/kg).
In two retrospective cohort studies ( n = 235 and n =188), the authors found that increased ventilation resulting from large tidal volumes resulted in hypocarbia, a risk factor for subsequently developing BPD. In vitro cyclic cell stretch has been shown to upregulate the expression of proinflammatory cytokines by human alveolar epithelial cells without any structural cell damage. Tidal volumes large enough to cause similar cell stretch in vivo , without causing structural damage, may similarly initiate the cascade of proinflammatory cytokines, recruiting inflammatory cells and causing tissue damage. All of the above-described pulmonary insults occur at a time when most preterm infants have a relative adrenocortical insufficiency, which may potentiate the inflammatory effects.
Infection
Although the role of infection is incompletely understood, infants exposed to antenatal and postnatal infection seem to be at higher risk for developing BPD. Antenatal chorioamnionitis may play a key role in the production of a fetal pulmonary inflammatory response to the release of proinflammatory cytokines. This response can lead to aberrant wound healing and fibrosis, causing inhibition of alveolarization and vascular development, hallmarks of the new BPD. Similarly, infants who developed late-onset sepsis (>3 days of age) were more likely to need long-term mechanical ventilation, and were more likely to develop BPD. In a retrospective study, early tracheal colonization also predisposed to the subsequent development of BPD.
Nosocomial infection plays a role in some cases of CLDI, especially in association with a symptomatic patent ductus arteriosus PDA, particularly in ELBW infants. In a series of 119 ELBW infants who had mild or no initial RDS, BPD was significantly more likely to occur with patent ductus arteriosus (odds ratio [OR] 6.2) and sepsis (OR 4.4). The risk of BPD increased substantially in infants with both conditions (OR 48.3). Neither ductal ligation nor prophylactic use of low-dose indomethacin initiated in the first 24 hours has been shown to reduce significantly the incidence of CLDI, however.
Specific organisms also may play a role. In one report, development of BPD was associated with isolation of Ureaplasma urealyticum in tracheal aspirates performed on the first (before surfactant administration) and fourth days of mechanical ventilation in infants less than 28 weeks gestational age.
Inflammation
Macrophages, lymphocytes, and platelets in the lung release multiple inflammatory mediators, including cytokines, lipid mediators, and platelet factors, which interact with endothelial and epithelial cells. The airspaces of ventilated preterm infants contain many proinflammatory and chemotactic factors that are present in greater concentration in infants who subsequently develop BPD. The presence of these mediators is associated with complement activation, increased vascular permeability, protein leakage, and mobilization of neutrophils into the interstitial and alveolar compartments. Release of ROS, elastase, and collagenase by activated neutrophils can damage lung structures. Interaction between macrophages and other cell types may perpetuate the production of proinflammatory mediators and sustain the cycle of lung injury. Persistence of factors such as macrophage inflammatory protein-1 and IL-8 and decreases of counter-regulatory cytokines such as IL-10 may lead to unregulated and persistent inflammation.
Bombesin-like Peptides
Injury may be mediated partly by bombesin-like peptides (BLP), which are derived from pulmonary neuroendocrine cells and play an important role in normal lung growth and maturation. In one study, the number of BLP-positive cells was greater in infants who died with BPD than in controls. In a baboon model, urine BLP levels were increased soon after birth in animals who developed BPD, and administration of anti-BLP antibody attenuated the disorder. In infants ≤28 weeks gestational age, elevated urine BLP levels in the first 4 days after birth were associated with an increased risk of BPD.
Nutrition
Premature infants have very poor nutritional reserves and are at high risk for being malnourished and entering a catabolic state if not provided with adequate nutrition. The goal of postnatal nutrition is to provide adequate substrate to approximate the intrauterine rate of growth. In VLBW infants, this goal can be extremely difficult to achieve for the following reasons :
- •
Fluid restriction to prevent pulmonary edema
- •
Heart failure secondary to a patent ductus arteriosus
- •
Use of postnatal corticosteroids
- •
Decreased gastrointestinal absorption secondary to suspected or proven necrotizing enterocolitis
- •
Hypoxia and chronic respiratory acidosis
- •
Anemia of prematurity
- •
Medications that increase metabolic rate, such as methylxanthines and β-sympathomimetics
Malnutrition or undernutrition renders an infant more susceptible to injury resulting from hyperoxia, volutrauma/barotrauma, and infections, and impairs the infant’s ability to recover from this injury. In addition, infants with BPD are significantly more likely to have lower early protein and total energy intake.
Genetic Factors
The pathogenesis of BPD is complex, with an intricate interaction of preterm birth, the in utero environment, inflammation/infection, fluid management, vascular maldevelopment, surfactant deficiency, mechanical ventilation, the balance of oxidative stress and antioxidant systems, and nutrition. Not all factors are required to develop BPD, and severe BPD may develop in infants who have a benign perinatal course. It had been postulated that there might be a genetic predisposition to develop BPD. Several studies have looked at the development of BPD in singleton and multiple gestation VLBW infants. In the earliest study comparing the rate of BPD among 108 VLBW twins, it was reported that BPD in one twin significantly predicted BPD in the other twin (adjusted OR 12.3, P < .001), even after adjusting for birth weight, gestational age, gender, RDS, pneumothorax, and patent ductus arteriosus. More recently, in a multicenter retrospective study of 450 twin pairs born at 32 weeks, the concordance of BPD was higher in monozygotic twins than predicted, after controlling for all covariates.
In addition to twin studies, many other investigators have attempted to look for candidate genes that may predispose to developing BPD. Genes involved in the differential regulation of lung development and the response to lung injury have been probed to determine whether they participate in the pathogenesis of BPD. Two separate studies looking at polymorphisms of angiotensin-converting enzyme (ACE) hypothesized that increased ACE activity, leading to increased aldosterone production and increased water retention, would increase the incidence of BPD. Kazzi and Quasney found that the polymorphism that conferred increased ACE activity showed increased incidence of BPD, whereas Yanamandra and colleagues did not find an association between ACE activity and incidence of BPD.
Other groups have examined the role of polymorphisms in the gene encoding for GST. GST is an innate defense mechanism against ROS and is found in various human tissues. A polymorphism of the GST-P1 gene produces two isoforms, one of which is significantly more efficient in eliminating oxidative toxins. In a small pilot study of 35 infants with BPD, it was shown that infants who developed BPD were more likely to have the less efficient form of the enzyme. Groups that have attempted to determine the role of various polymorphisms of the anti-inflammatory cytokines IL-4 and IL-10, the anti-inflammatory transforming growth factor-β (TGF-β), and the proinflammatory chemokine monocyte chemoattractant protein-1 have found no association between allelic variants and the development of BPD.
Finally, the role of allelic variants of various surfactant proteins in the development of RDS and BPD has been explored. Specifically, the surfactant protein A (SP-A) allele 6A-6 is more common in infants with BPD. Many loss-of-function mutations of the surfactant protein B (SP-B) gene have been reported. The clinical phenotype can vary, ranging from chronic respiratory failure to refractory hypoxic respiratory failure in the neonatal period. One group looked at polymorphisms in intron 4 of the SP-B gene. They found that BPD was more common in infants who had the polymorphisms in intron 4. The definition of BPD that they used was that of oxygen requirement at 28 postnatal days, however; if the definition of oxygen requirement at 36 weeks PMA was used, there were no differences in the wild-type versus the intron 4 variants.
Pathology
In surfactant-treated ELBW infants, the characteristic pathologic finding of BPD is disruption of lung development. Decreased septation and alveolar hypoplasia lead to fewer and larger alveoli. Reduced microvascular development also may occur. These changes have been observed in infants who died of BPD, biopsy specimens from severely affected infants, and a baboon model of the disorder. These findings are in contrast to BPD seen in infants before the availability of surfactant replacement therapy. The prominent pathologic findings in those cases were airway injury, inflammation, and parenchymal fibrosis. Similar changes may be seen in surfactant-treated infants who develop severe BPD. In severely affected infants, fibrosis, bronchial smooth muscle hypertrophy, and interstitial edema may be superimposed on the characteristic reduced numbers of alveoli and capillaries. Pulmonary vascular changes, such as abnormal arterial muscularization and obliteration of vessels, may occur.
Lung injury also is associated with increased elastic tissue formation and thickening of the interstitium. These tissue deformations may compromise septation and capillary development. Disturbed elastic tissue maturation was shown in a study of 44 infants, 23 to 30 weeks gestational age, who died at 5 to 59 days of age. In infants with high respiratory scores computed from supplemental oxygen concentration and mean airway pressure, the volume, density, and absolute quantity of elastic tissue were significantly greater than those of infants with low scores or control infants. Alveolar and duct diameters and septal thickness also were greater. With increased elastic tissue content and thickened interstitium, the lungs tend to collapse at end expiration and have a low functional residual capacity (FRC).
Clinical Features
BPD predominantly affects ELBW infants, and most affected infants are ventilator-dependent from birth with severe RDS requiring surfactant therapy. The evolution to BPD typically is recognized at approximately 2 weeks of age as pulmonary function deteriorates rather than improves. The infant remains ventilator-dependent, and the concentration of supplemental oxygen must be increased to maintain adequate oxygen saturation. Wide swings in oxygenation may occur, likely caused by intermittent atelectasis.
Physical Examination
The physical examination is variable. Infants usually are tachypneic, and depending on the extent of pulmonary edema or atelectasis or both, they may have mild to severe retractions, and crackles may be audible. Intermittent expiratory wheezing may be present in infants with increased airway reactivity.
Chest Radiograph
As BPD evolves, the chest radiograph becomes diffusely hazy, reflecting inflammation or pulmonary edema or both ( Fig. 1-3A ), with low to normal lung volumes. There may be areas of atelectasis that alternate with areas of gas trapping, related to airway obstruction from secretions or other debris. The chest radiograph in infants who develop severe BPD shows hyperinflation ( Fig. 1-3B ). Streaky densities or cystic areas may be prominent, corresponding to fibrotic changes. During acute exacerbations, pulmonary edema may be apparent ( Fig. 1-3C ).
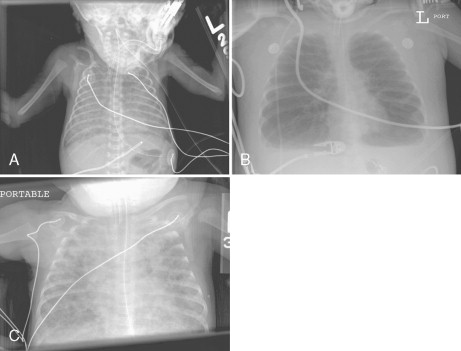
Clinical Course
Most infants improve gradually during the next 3 to 4 months. As pulmonary function improves, infants can be weaned to CPAP, then supplemental oxygen alone, until they can maintain adequate oxygenation breathing room air. Some infants develop severe BPD that leads to prolonged ventilator dependence. The clinical course during the first few weeks after birth includes marked instability with frequent changes in oxygenation and intermittent episodes of acute deterioration requiring increased ventilator support. The marked instability typically improves after 4 to 6 weeks.
Severely affected infants may develop pulmonary hypertension and cor pulmonale. Elevated pulmonary vascular resistance may impair pulmonary lymphatic drainage and exacerbate interstitial edema. In some cases, anastomoses develop between pulmonary and systemic vessels that may worsen the pulmonary hypertension.
Cardiopulmonary Function
Abnormalities of pulmonary function in severe BPD include decreased tidal volume, increased airway resistance, and low dynamic lung compliance, which become frequency dependent. Uneven airway obstruction leads to air trapping and hyperinflation with abnormal distribution of ventilation. Bronchomalacia can result in airway collapse during expiration, and severely affected infants can have hypoxemia and hypercapnia.
Pulmonary vascular resistance is increased because of reduced cross-sectional area of pulmonary vessels ( Fig. 1-4 ). In addition, alveolar hypoxia in underventilated areas of the lung induces local vasoconstriction. Intact vessels in well-ventilated areas of the lung accept a disproportionate amount of pulmonary blood flow. Because these vessels are already fully recruited and dilated, the additional flow results in elevated pressure and increased right ventricular afterload. The high microvascular pressure promotes increased fluid filtration into the perivascular interstitium. Elevated right atrial pressure inhibits pulmonary lymphatic drainage, further promoting pulmonary edema.
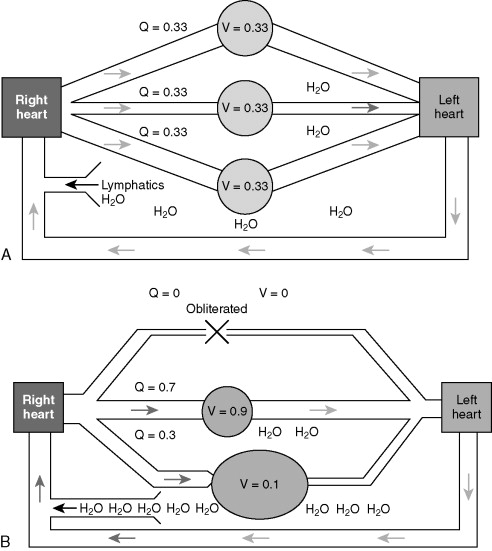
Management
The management of infants with BPD begins with prevention, attempting to avoid or minimize contributory factors.
Respiratory Care
Respiratory care of an infant with developing or established BPD is supportive and should aim to minimize additional lung injury by judicious use of mechanical ventilation and supplemental oxygen. Early use of continuous distending pressure in at-risk infants reduces the need for subsequent positive-pressure ventilation. The COIN trial compared CPAP with mechanical ventilation in infants born at 25 to 28 weeks gestation. In a preliminary report, the authors found that there were no significant differences in the incidence of BPD (oxygen treatment at 36 weeks PMA), days of respiratory support, oxygen treatment, hospital stay, incidence of grade III or IV intraventricular hemorrhage, cystic periventricular leukomalacia, or home oxygen use. There was an increased rate of pneumothorax in the CPAP group.
If mechanical ventilation is needed, the lowest peak airway pressure necessary to ventilate adequately should be used, and large tidal volumes should be avoided. Although this strategy is supported by few data in human neonates, the use of lower than routine tidal volumes decreased mortality and increased the number of ventilator-free days in adults with acute respiratory distress syndrome. In addition, in a multicenter trial conducted by the NICHD Neonatal Research Network, the need for ventilator support at 36 weeks PMA was significantly lower in the minimal ventilation group, although this strategy did not change the relative risk of death or BPD at 36 weeks PMA, the primary outcome. Although the very low tidal volumes associated with high-frequency oscillatory ventilation (HFOV) might be expected to reduce the rate of BPD, only one of two large trials comparing HFOV and conventional ventilation in patients at the highest risk showed an effect. This finding suggests that routine initial use of HFOV generally is not warranted.
The most appropriate range of arterial oxygenation in preterm infants with acute or chronic respiratory failure is unknown. High levels of oxygen saturation seem to offer no advantages, however, and pulmonary injury may result from the increased concentration of supplemental oxygen required to maintain higher saturations, as shown by two studies. In the STOP-ROP trial, providing supplemental oxygen to maintain higher compared with routine oxygen saturation (96% to 99% versus 89% to 94%) in infants with prethreshold retinopathy of prematurity did not reduce progression to threshold retinopathy of prematurity or the need for peripheral retinal ablation. The incidence of pulmonary events, including pneumonia/exacerbation of BPD, and the need for oxygen, diuretics, and hospitalization at 3 months corrected age was higher, however, in the higher saturation group. Similarly, in the BOOST trial, in infants less than 30 weeks gestation who were oxygen dependent at 32 weeks PMA, no differences were detected in growth and neurodevelopment at 12 months corrected age—the primary outcomes—between the groups maintained at high (95% to 98%) or standard (91% to 94%) oxygen saturation ranges. Infants in the high saturation group received oxygen for a longer time, however, and had higher rates of oxygen dependence at 36 weeks PMA and home oxygen therapy.
Respiratory management in infants with developing or established BPD should ensure adequate tissue oxygenation to promote growth and prevent pulmonary arterial hypertension that can result from chronic hypoxemia, and should minimize lung injury from excessive oxygen levels or mechanical ventilation. Although the safest levels are unknown, oxygen saturation is generally maintained in the 85% to 95% range, with PaO 2 greater than 50 mm Hg, and PaCO 2 should be allowed to increase to 50 to 60 mm Hg or possibly even higher in infants with the most severe disease as long as the pH is normal. When the infant is able to maintain adequate PaCO 2 and PaO 2 on the lowest ventilator settings, weaning from assisted ventilation should be attempted. Episodes of hypoxemia should be avoided because these are associated with increased airway resistance. Supplemental oxygen may be needed for several months or longer in the most severe cases.
Nutrition and Fluid Management
Nutrition is a key component of the management of infants with BPD. Nutrition, supplied enterally or parenterally or both, must be sufficient to promote somatic growth and the development of new alveoli; this should facilitate weaning from mechanical ventilation and decrease vulnerability to infection that is associated with malnutrition. Energy also must be sufficient to meet the demands of increased work of breathing. Plans for dietary support of infants with BPD who fail to thrive should consider that an excessive intake of carbohydrate might be associated with increased CO 2 production and impair respiratory function further. Insufficient caloric intake may potentiate oxygen toxicity and impair cell multiplication and lung growth. Deficiencies in sulfur-containing amino acids may reduce lung glutathione, an important antioxidant. The adequacy of nutrition should be monitored closely, and growth charts for weight, head circumference, and length should be maintained.
Vitamin deficiency may occur and interfere with lung healing. Vitamin A is an essential micronutrient for the normal growth and differentiation of epithelial cells. Vitamin A levels are lower in infants with severe BPD than in infants without BPD. In animal models, low vitamin A levels contribute to airway abnormalities, such as loss of ciliated epithelium or squamous metaplasia, similar to histologic changes seen in BPD, and these changes are reversed by normalization of vitamin A levels. Vitamin E is another important micronutrient and is a ubiquitous antioxidant. A small study has shown a strong correlation between low vitamin E levels in the cord blood and on the third day of life and the subsequent development of BPD, but further studies are needed before the routine use of vitamin E supplementation can be recommended. Another key antioxidant that is deficient in preterm infants is vitamin C. In a pilot study with premature baboons, high-dose vitamin C treatment did not prevent pulmonary oxygen toxicity. Of concern is that vitamin C in high doses can induce oxidative stress and have adverse effects on the developing lung. At this time, it is recommended that specific vitamin deficiencies should be avoided in infants with BPD by providing adequate supplementation.
Inflammatory changes in the lungs of infants with BPD promote water retention. As a result, these infants tolerate excess fluid administration poorly, and fluids should be modestly restricted (140 to 150 mL/kg/day) to avoid pulmonary edema. Further restriction may be needed in severely affected infants. Additional supplementation of human milk or formula with calories or protein or both may be required to ensure adequate nutrition in these infants.
Diuretics
Administration of the loop diuretic furosemide, hydrochlorothiazide, or spironolactone, which acts on the distal tubule, results in acute, nonsustained improvements in pulmonary mechanics. The improvement in pulmonary mechanics seen with furosemide administration may be independent of its diuretic effect, as venous capacitance increases, and pulmonary blood flow decreases in response to furosemide administration. In a Cochrane systematic review and meta-analysis, however, diuretic administration did not reduce the need for ventilator support, reduce the duration of hospital stay, or improve long-term outcomes. Long-term administration of a diuretic is often complicated by metabolic abnormalities, such as a hypokalemic, hypochloremic metabolic alkalosis, hypercalciuria leading to nephrocalcinosis, osteopenia, impaired growth, and hearing loss (with furosemide administration).
Although no evidence exists for long-term benefit, diuretics frequently are used in the management of infants with BPD to improve pulmonary function acutely. This use of diuretics may be beneficial in infants with a pulmonary exacerbation thought to be caused by pulmonary edema or to reduce the effects of circulatory overload after a packed red blood cell transfusion. Whether diuretic therapy facilitates optimal nutrition by reducing the need for fluid restriction requires further study. If diuretics are used, close monitoring of serum electrolytes is needed, and supplementation may be required to compensate for urinary losses.
Bronchodilator Therapy
Infants with severe BPD have increased baseline airway resistance that increases further with periods of hypoxemia, leading to respiratory decompensation owing to bronchoconstriction. This condition is sometimes treated with inhaled bronchodilators, a practice that has been extrapolated from the treatment of asthma. Infants with BPD treated with β-agonists respond with a short-term increase in compliance and tidal volume and decrease in airway resistance. This treatment has not been shown to affect long-term outcome, however. In one trial in which 173 ventilator-dependent infants less than 31 weeks gestation were randomly assigned to inhaled salbutamol (albuterol), beclomethasone, combination salbutamol and beclomethasone, or placebo, treatment resulted in no difference in duration of mechanical ventilation or oxygen supplementation, diagnosis or severity of BPD at 28 days, or survival. The efficacy of anticholinergic agents in the treatment of BPD has not been studied in randomized trials. Individual infants can be treated with bronchodilators to achieve short-term improvement in pulmonary mechanics. Continued use should depend on clinical response assessed by improvement in gas exchange and respiratory effort.
Corticosteroids
Administration of systemic corticosteroids to infants with evolving or established BPD reduces inflammation and improves lung mechanics, facilitating extubation. Systemic corticosteroid use is associated with short-term adverse effects, however, such as hyperglycemia, glucosuria, and hypertension, and mortality is not reduced. In addition, outcome studies have suggested that corticosteroid treatment, especially dexamethasone, may contribute to poor neurodevelopmental outcome and cerebral palsy. These concerns led the American Academy of Pediatrics Committee on Fetus and Newborn to recommend that the routine use of dexamethasone should be avoided and its use limited to extreme circumstances. That the likelihood of dexamethasone treatment leading to adverse outcomes is influenced by the baseline risk of developing BPD was suggested by a meta-regression analysis. Whether infants at extremely high baseline risk for developing BPD would benefit from dexamethasone remains to be established in clinical trials.
Data on the use of inhaled steroids are insufficient to recommend their routine use. Corticosteroids given via a metered dose inhaler and holding chamber or nebulized budesonide have been used successfully, however, even in patients younger than 1 year. The inhaled route is the preferred route for preventing side effects of systemic corticosteroids. Infants with CLDI treated with inhaled corticosteroids should be monitored for potential side effects, including delayed growth, increased blood pressure, osteoporosis, adrenal suppression, and cataracts.
Prevention
Antenatal Corticosteroids
Antenatal corticosteroids given to women at risk for preterm delivery decreases the risk of RDS, intraventricular hemorrhage, and mortality. They should be given to any pregnant woman 24 to 34 weeks gestation with intact membranes at high risk for preterm delivery within 7 days of administration. Treatment does not decrease the incidence of BPD, however, partly because increased survival has resulted in more infants at risk for the condition.
Fluid Restriction
Higher fluid intake associated with a lack of postnatal weight loss during the immediate postnatal period has been proposed as a predisposing risk factor for BPD. This hypothesis is supported by a retrospective report of premature infants (birth weight 401 to 1,000 g) from the Neonatal Research Center study that found infants who either died or developed BPD had a higher fluid intake and a lower weight loss during the first 10 days of life compared with infants who survived without BPD. Small trials of fluid restriction have not shown a consistent effect on the development of BPD, although this strategy may minimize pulmonary edema. In one study, 168 ventilated infants, gestational age 23 to 33 weeks, were randomly assigned to receive routine fluid volumes (60 mL/kg on the first day, progressing to 150 mL/kg on the seventh day) or 80% of routine volume. Similar proportions in each group had BPD (26% versus 25%) and survived without oxygen dependency at 36 weeks PMA (58% versus 52%). Significantly fewer restricted infants received postnatal corticosteroid treatment (19% versus 43%), however, suggesting that their clinicians may have considered them less ill than were controls. In addition, the duration of oxygen requirement was significantly associated with colloid infusion.
Minimal Ventilation
Ventilation with high tidal volumes results in mechanical injury to the lung. Mechanical ventilation with small tidal volumes and target goals of modest permissive hypercapnia (PCO 2 50 to 55 mm Hg) may protect the lung from mechanical injury. The benefit of permissive hypercapnia has not been shown definitively in newborns at high risk for BPD, although additional studies are needed. In one trial, infants with birth weight 501 to 1,000 g requiring mechanical ventilation before 12 hours of age were randomly assigned to minimal ventilation (target PCO 2 >52 mm Hg) or routine ventilation (PCO 2 <48 mm Hg) and a course of dexamethasone or placebo. The trial was stopped after enrollment of 220 infants because of excess adverse events in infants treated with dexamethasone. There was no difference between ventilator groups in the combined primary outcome of death or BPD. At 36 weeks PMA, the proportion of infants requiring mechanical ventilation was significantly less in the minimal group (16% versus 1%).
The lack of difference in lung injury between the minimal and routine ventilation groups may be due partly to an insufficient sample size. Another reason may be that target levels of PCO 2 rather than tidal volume distinguished the groups because the former usually is not measured continuously, and the difference in PCO 2 between groups was small. Because ventilated infants continued to breathe spontaneously, the measured PCO 2 reflected the selected airway pressure (which determines the tidal volume) and rate and the infant’s own ventilation. Spontaneous breathing may have resulted in the modest increase in PCO 2 in the minimal ventilation group and minimized the difference between groups.
High-Frequency Oscillatory Ventilation
HFOV, a technique of rapid ventilation with very small tidal volumes, reduces lung injury in animal models compared with conventional ventilation. Nearly all trials comparing HFOV with conventional ventilation performed in preterm infants at risk for the disorder since surfactant replacement therapy has been available show no effect on the rate of BPD.
In one trial in 500 preterm infants, 601 to 1,200 g birth weight, the proportion of infants who survived without BPD was slightly greater with HFOV (56% versus 47%). This study was performed in centers experienced in the use of HFOV and followed strict protocols for management. Similar results may not be achieved at centers with less experience or without strict protocols. It is recommended that conventional ventilation with low tidal volumes and modest hypercapnia, rather than HFOV, be employed as the initial mode of mechanical ventilation for most preterm infants.
Vitamin A
Extremely preterm infants may have vitamin A deficiency, which may promote the development of BPD. Possible mechanisms include impaired lung healing, increased squamous cell metaplasia, reduced alveolar number, increased susceptibility to infection, and increased loss of cilia. Supplementation with vitamin A reduces the risk of BPD in susceptible infants.
In the largest trial, 807 infants with birth weight 401 to 1,000 g who received mechanical ventilation or supplemental oxygen at 24 hours of age were randomly assigned to receive 5000 IU vitamin A intramuscularly three times per week for 4 weeks or a sham injection. The combined outcome of death or BPD (oxygen requirement at 36 weeks PMA) occurred significantly less often in the vitamin A group compared with control group (55% versus 62%). No clinical or biochemical evidence of vitamin A toxicity was detected. In a subsequent study of the original cohort, vitamin A supplementation did not differ from placebo in reducing hospitalizations or pulmonary problems after discharge from the nursery. In addition, at a corrected age of 18 to 22 months, there were no differences in mortality or neurodevelopmental impairment between the treated and nontreated infants. Some clinicians provide supplemental vitamin A (5000 IU intramuscularly three times per week for 4 weeks) to infants ≤1,000 g birth weight who require ventilatory support within 24 hours after birth. The decision to treat may depend on a balance of factors, such as the local incidence, the value of a modest decrease in BPD, and the need for repeated intramuscular injections.
Systemic Corticosteroids
Administration of systemic corticosteroids reduces the risk of BPD. Routine use of corticosteroid therapy is not recommended, however, because there are concerns about significant short-term and long-term adverse effects. In systematic reviews by the Cochrane database, treatment with corticosteroids (usually dexamethasone) before 96 hours of age or at 7 to 14 days of age reduced the development of BPD at 36 weeks PMA and the need for oxygen supplementation at 28 postnatal days, and promoted earlier extubation, compared with control. Survival did not improve with treatment, however. In addition, systemic corticosteroid use was associated with short-term adverse effects, including hypertension, hyperglycemia, poor growth, and gastrointestinal bleeding and perforation.
Systematic reviews of long-term outcome (≤4 years of age) suggest that postnatal dexamethasone use increases neurodevelopmental delay and cerebral palsy. A review that included nine randomized controlled trials of postnatal corticosteroids administered within the first week of life found that the risk of cerebral palsy was greater among surviving infants who were treated compared with controls (24% versus 14%, relative risk 1.75, 95% confidence interval 1.25 to 2.44). A potential problem in the interpretation of these results is that many infants enrolled in trials of corticosteroids were treated with dexamethasone by their clinicians in addition to the study drug (open-label treatment). Children who participated in one of the trials included in the above-mentioned systematic reviews were evaluated at school age. Among the original cohort of 262 infants, 159 survived to school age, and 146 were included in follow-up (evenly distributed between treatment and control groups). Clinically significant disabilities (motor skills, coordination, visual motor integration, and IQ) were more common among children from the treatment group (39% versus 16%). Differing rates of disability persisted when the children who received open-label dexamethasone treatment were excluded from the analysis (41% versus 21%).
Even when administered in low doses or when other agents aside from dexamethasone are used, systemic corticosteroids are associated with serious adverse effects. In a multicenter trial, mechanically ventilated ELBW infants were randomly assigned to hydrocortisone therapy or placebo to determine whether prophylaxis of early adrenal insufficiency affected clinical stability and incidence of BPD. Infants receiving hydrocortisone were treated with 1 mg/kg/day for 12 days and then 0.5 mg/kg/day for 3 days. Enrollment was discontinued early because of an increase of spontaneous gastrointestinal perforation in the hydrocortisone group (9% versus 2%). Overall survival without BPD did not differ between groups.
Although most of the data show the negative impact of corticosteroids, with risk of short-term and long-term adverse effects including impaired neurodevelopmental outcomes, it remains unclear whether there is a potential role for corticosteroids in selected populations. In the meta-analysis described earlier, there was a significant negative correlation between the corticosteroid effect on combined outcome of death and cerebral palsy and the rate for BPD in the control groups. The rate of BPD was used as a marker for the risk for BPD and was not a variable available at trial entry. This relationship suggests that in a population of preterm infants at high risk for BPD, corticosteroid therapy may decrease the risk of death or cerebral palsy. At present, the American Academy of Pediatrics and the Canadian Pediatric Society recommend that dexamethasone should not be used routinely in VLBW infants to treat or prevent chronic lung disease because of its limited short-term benefits, no apparent long-term benefits, and substantial risk of short-term and long-term complications. They further recommend that dexamethasone use should be limited to controlled trials. Outside of a trial, corticosteroids should be used only in exceptional clinical circumstances, such as an infant who requires maximal ventilatory and oxygen support. In this case, the parents should be made aware of potential risks and agree to treatment.
Inhaled Corticosteroids
In a systematic review and meta-analysis by the Cochrane database, early postnatal administration of inhaled corticosteroids did not prevent BPD, but was associated with lower rates of systemic corticosteroid treatment. In the largest trial, 253 infants with gestational age less than 33 weeks and birth weight ≤1,250 g who were mechanically ventilated at 3 to 14 days of age were randomly assigned to a 4-week course of beclomethasone (tapering dose of 40 μg/kg/day to 5 μg/kg/day) or placebo. The need for supplemental oxygen at 28 days (43% versus 45%) and 36 weeks PMA (18% versus 20%) was similar in the beclomethasone and placebo groups. Beclomethasone significantly reduced the rate of systemic corticosteroid use (relative risk 0.8) and mechanical ventilation (relative risk 0.8) at 28 days of age. No adverse effects were observed. In a separate report from the same trial, beclomethasone therapy was associated with slightly lower median basal cortisol levels (5 μg/dL versus 6 μg/dL) compared with placebo, but similar response to cosyntropin stimulation.
Inhaled Nitric Oxide
Inhaled nitric oxide (iNO) is used in the management of term neonates with hypoxic respiratory failure, but little is known about the effects of iNO in the preterm population. Multiple physiologic effects of nitric oxide are known. Exposure to chronic hypoxemia leads to remodeling that includes increased proliferation of pulmonary vascular smooth muscle, leading to increased pulmonary vascular pressures, and eventual right ventricular hypertrophy and cor pulmonale. Several animal studies have shown that iNO prevents or ameliorates this remodeling of the pulmonary vascular bed. Of particular concern is the effect of iNO on coagulation; iNO is known to increase bleeding time in adult patients, presumably via a cyclic guanosine monophosphate–dependent mechanism causing platelet dysfunction. In a preterm infant already at risk for intraventricular hemorrhage, this complication would add significant long-term morbidity.
Several studies of the use of iNO in preterm neonates have been conducted, and several included BPD as an outcome. A Cochrane systematic review and meta-analysis has shown that the use of iNO in infants less than 35 weeks of age does not show any benefit in terms of survival without BPD; there also is a trend toward an increase in the combined outcome of severe intraventricular hemorrhage or periventricular leukomalacia.
Caffeine
Caffeine has been shown to reduce the frequency of apnea of prematurity and the need for mechanical ventilation. A study of 1917 infants with birth weight 500 to 1,250 g showed that the rate of BPD (a secondary outcome) in infants treated with caffeine was significantly lower than in the placebo group (36% versus 47%). The rates of adverse outcomes—specifically death, brain abnormalities by head ultrasonography, and necrotizing enterocolitis—were not different between the two groups. This cohort has been followed to assess the primary outcome measure, which is the combined rate of mortality and neurodevelopmental disability in survivors at a corrected age of 18 to 21 months, and the authors more recently reported improved survival without neurodevelopmental disability in the caffeine-treated group. Neurodevelopmental outcomes in this cohort also will be followed up at 5 years of age.
Superoxide Dismutase
Preterm infants may have inadequate antioxidant defense because of nutrient deficiencies or immature enzyme development. Postnatal administration of antioxidants such as superoxide dismutase may protect against oxidant injury, although additional evidence is needed. In one study performed after replacement surfactant became available, 33 infants with birth weight 700 to 1,300 g who were mechanically ventilated for RDS were randomly assigned to intratracheal administration of recombinant human copper/zinc superoxide dismutase (rhSOD) (2.5 mg/kg or 5 mg/kg) or saline every 48 hours while they remained intubated, for up to seven doses. Clinical outcomes did not differ between groups. Tracheal aspirate inflammatory markers (neutrophil chemotactic activity, albumin concentration) were lower, however, in the rhSOD groups than in the control group. In a larger multicenter trial, no difference was found in the rate of oxygen dependence at 28 postnatal days or 36 weeks PMA. At 1 year of age, infants who received rhSOD had less respiratory illness, however. Growth and neurodevelopmental status were similar.
Summary
Multiple strategies are needed to prevent BPD in high-risk patients.
- •
Although antenatal corticosteroids do not affect the incidence of BPD, they reduce the risk of RDS and intraventricular hemorrhage, and improve survival in preterm infants. Antenatal corticosteroids should be given to pregnant women at high risk for preterm delivery.
- •
Excessive fluid administration should be avoided in ELBW infants, and fluids should be restricted to minimize the development of pulmonary edema. Use of colloid infusions should be avoided.
- •
Conventional ventilation with low tidal volumes and reasonable ventilation goals (initially, PaCO 2 50 to 55 mm Hg) should be used in preterm infants with respiratory failure in most cases. In centers experienced with the technique, HFOV may be an appropriate alternative.
- •
Optimal nutrition should be provided to promote somatic and lung growth. Administration of supplemental vitamin A (5000 IU intramuscularly three times per week for 4 weeks) to ELBW infants who require early respiratory support should be considered.
- •
Routine use of postnatal dexamethasone should be avoided.
- •
Use of iNO to prevent BPD should await publication of follow-up studies.
- •
Caffeine decreases the rate of BPD in infants 500 to 1,250 g, without neurodevelopmental sequelae at 18 to 21 months of age.
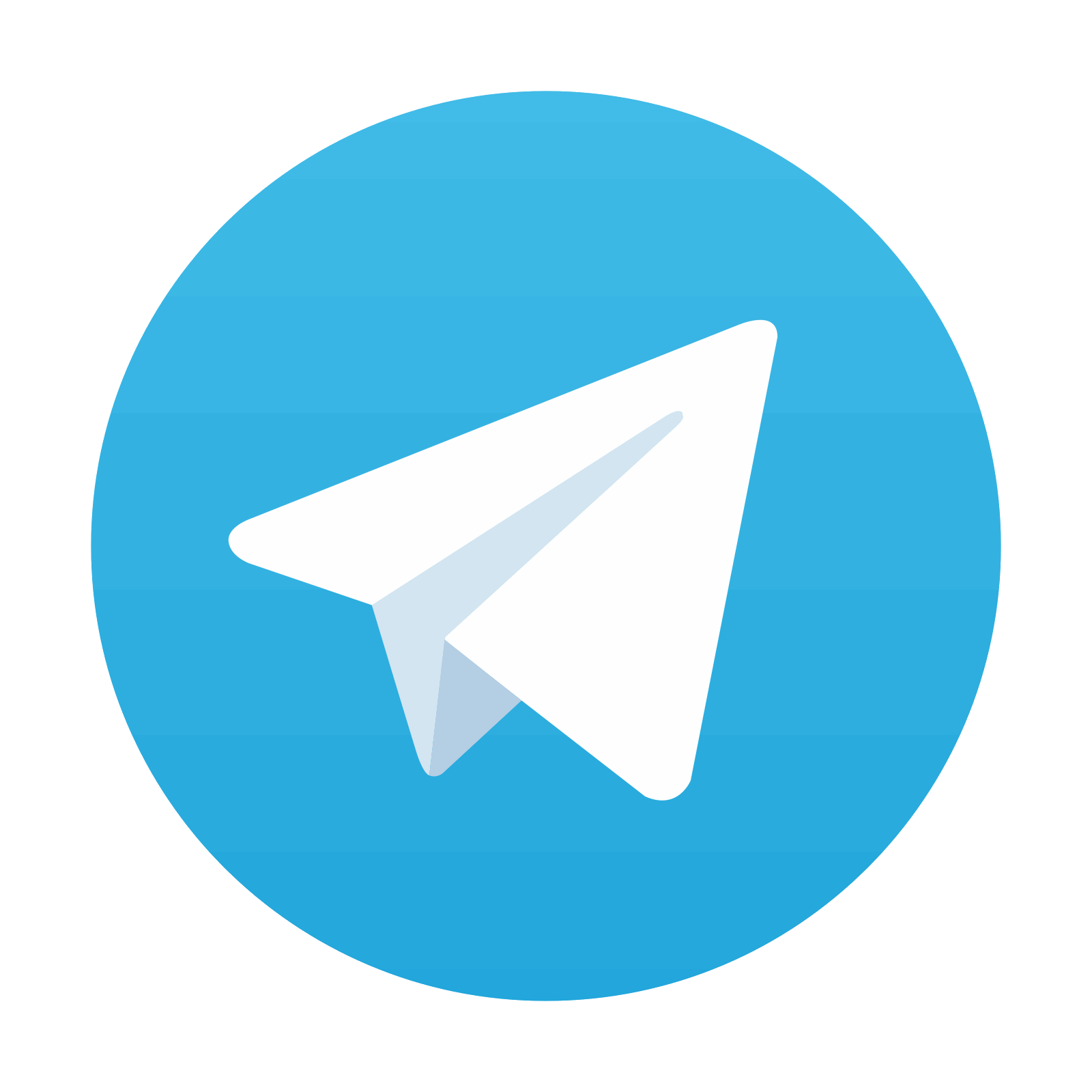
Stay updated, free articles. Join our Telegram channel

Full access? Get Clinical Tree
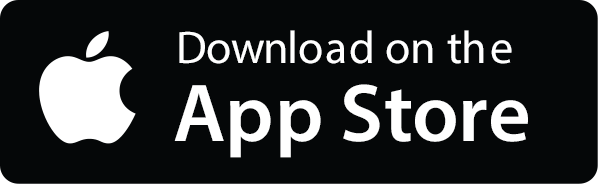
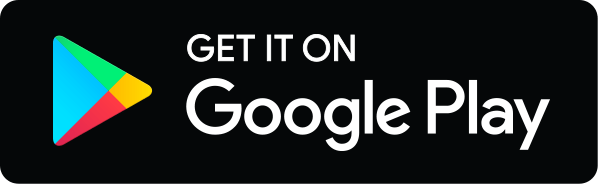