Diffusion magnetic resonance imaging is the best imaging tool for detecting acute ischemic brain injury. Studies have shown its high accuracy for delineating irreversible tissue damage within the first few hours after stroke onset; however, the true value of any diagnostic tool is whether it can be used to guide clinical management. This review discusses the role of diffusion imaging in the evaluation of the patient with acute ischemic stroke, and how this role is influenced by other important stroke-related variables, including the level of vessel occlusion and the clinical deficit. The review focuses on decision-making for intravenous and intra-arterial reperfusion therapies.
Acute ischemic stroke (AIS) is the third leading cause of death and the leading cause of severe disability in adults. There are approximately 700,000 ischemic strokes annually in the United States. Reperfusion therapy is the only proven treatment of AIS. Current treatment approaches include intravenous tissue plasminogen activator (IV tPA), thrombolytic and/or mechanical intra-arterial therapies (IATs), and combined intravenous/intra-arterial (IV/IA) bridging therapy.
The randomized controlled trials (RCTs) that have validated reperfusion therapy (and guide current clinical practice) used noncontrast computed tomography (NCCT) criteria for assessment of parenchymal injury. Patients were excluded from therapy if they had infarcts that involved greater than one-third of the middle cerebral artery (MCA) territory or that resulted in severe edema or mass effect. However, it is widely believed that advanced neuroimaging methods including diffusion magnetic resonance (MR) imaging provide a better means of patient selection than NCCT, and may allow the treatment window to be extended for patients with AIS.
This article reviews the evidence for using diffusion MR imaging in the evaluation of patients with AIS, with a focus on extended window reperfusion therapies.
Imaging the infarct core using diffusion MR imaging
Within the first several hours after the occlusion of a cerebral vessel, the hypoperfused territory is composed of tissue that is irreversibly injured (the infarct core) and a surrounding region of threatened but viable tissue (the ischemic penumbra). The infarct core grows into the penumbra to an extent determined largely by the collateral circulation. Within the infarct core, cell death occurs via a variety of mechanisms, including excitotoxicity, oxidative/nitrosative stress, inflammation, apoptosis, and peri-infarct depolarization. The loss of cellular energy metabolism causes a failure of membrane ionic pumps, resulting in cytotoxic edema and restricted diffusion of water, which is detectable on diffusion MR imaging ( Fig. 1 ). The biophysical basis of this restricted diffusion is not clearly known, but proposed mechanisms include movement of water into the intracellular compartment, cell swelling resulting in reduction of the extracellular space, and increased cytoplasmic viscosity.
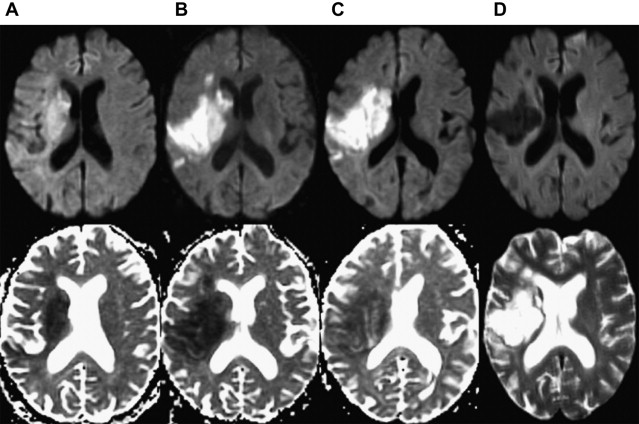
Of the available MR imaging and computed tomography (CT) measurements, abnormalities detected on diffusion MR imaging are considered the most reliable estimate of the infarct core, and are characterized by diffusion-weighted imaging (DWI) hyperintensity with associated reduction in apparent diffusion coefficient (ADC) signal. Diffusion imaging is highly sensitive (91%–100%) and specific (86%–100%) for the identification of early ischemic brain injury (within 6 hours of symptom onset), and has similar accuracy for the prediction of cortical infarction as [ 11 C]flumazenil positron emission tomography, which is a reliable marker of neuronal integrity. In comparison, NCCT and nondiffusion MR imaging suffer from low sensitivity (20%–50%; Fig. 2 ). Furthermore, because of its high image contrast, DWI shows superior interreader agreement compared with NCCT and conventional MR imaging for lesion detection and estimation of infarct size.
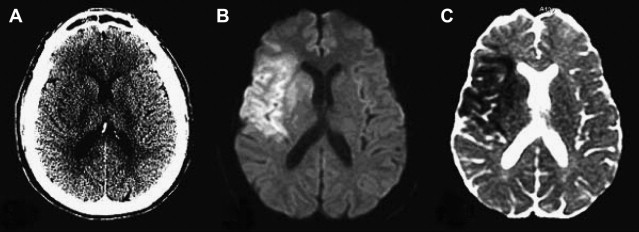
In the absence of therapy, ischemic diffusion abnormalities rarely reverse. With early reperfusion, partial reversal of diffusion abnormalities can be observed, with most rates ranging from 8% to 20% ( Fig. 3 ). Brain regions that undergo reversal typically have mild ADC depression, indicating that these areas have a mild reduction in blood flow, or that it is early in the course of ischemia. However, the volume of tissue with diffusion normalization is usually small (5.1–16 cm 3 ), and this normalization has not been shown to improve clinical outcomes. Any clinical benefit associated with diffusion imaging reversal is likely related to the effects of accompanying tissue reperfusion, notably penumbral salvage. Moreover, delayed regrowth into a previously observed diffusion abnormality frequently occurs, a phenomenon termed late secondary ischemic injury. Taken together, the evidence indicates that the signal abnormality observed on diffusion MR imaging is currently the best marker of the infarct core, an opinion supported by multiple expert panels (class I, level of evidence A).
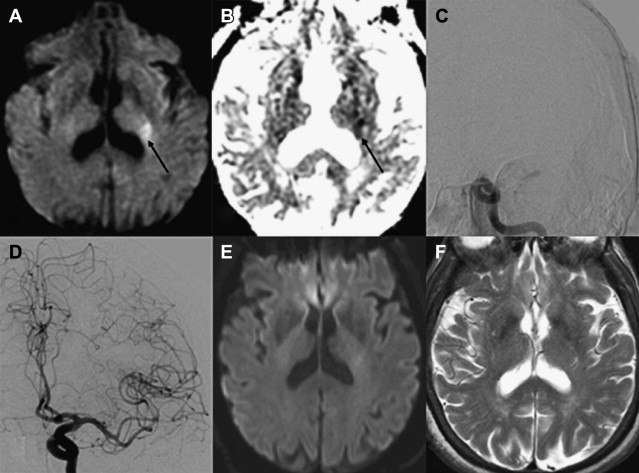
Using diffusion MR imaging to select patients for IV thrombolysis
Numerous studies have examined the usefulness of diffusion MR imaging for selecting patients for IV therapies outside the 3-hour window. The major selection criterion has been a volumetric mismatch between the perfusion and diffusion imaging lesions ( Fig. 4 ). This mismatch serves as an imaging surrogate of penumbral tissue.
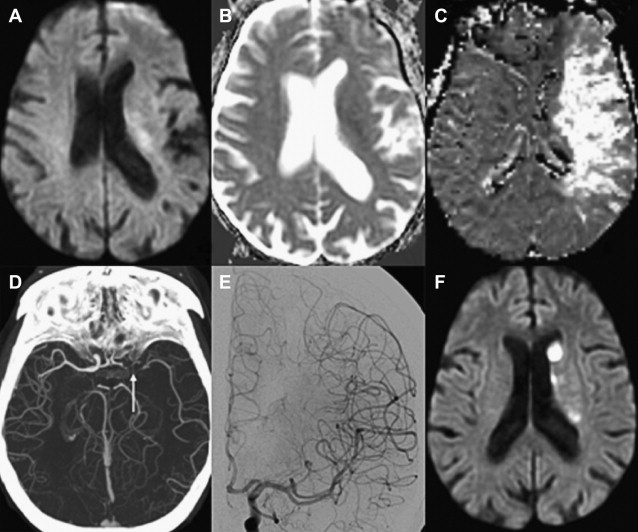
Evidence Supporting the Mismatch Hypothesis
Until the ECASS-3 (European Cooperative Acute Stroke Study 3) results, previous studies that used NCCT-based selection failed to extend the time window for IV tPA beyond 3 hours. ATLANTIS (Alteplase Thrombolysis for Acute Noninterventional Therapy in Ischemic Stroke), ECASS, and ECASS-2 could not show a clinical benefit that outweighed treatment risk in the 5-hour to 6-hour window. In comparison, multiple studies using MR imaging perfusion/diffusion mismatch have shown positive clinical results for extended window treatment with IV tPA, and provide the strongest evidence for this imaging approach.
In large multicenter studies, patient selection using the perfusion/diffusion mismatch was shown to safely and effectively extend the treatment window for IV tPA up to 6 hours. The most frequently used mismatch definition was a perfusion lesion at least 20% larger than the diffusion lesion. Furthermore, patients were excluded if they had extensive infarcts greater than one-third or one-half of the MCA territory. Patients with a perfusion/diffusion mismatch treated with IV tPA beyond 3 hours appeared to do just as well as patients treated within the 3-hour window using traditional NCCT criteria. In a multicenter study of 1210 patients, there were no significant differences in favorable 3-month clinical outcome (modified Rankin scale [mRS] score 0–1; 35.4% vs 40.0%), symptomatic intracerebral hemorrhage (sICH) (5.3% vs 4.4%), and mortality (13.7% vs 13.3%) between patients treated within 3 hours using NCCT versus those treated beyond 3 hours based on a 20% perfusion/diffusion mismatch. Onset-to-treatment time (OTT) was not a predictor of safety or efficacy in univariate or multivariate analysis. The perfusion imaging techniques were heterogeneous among the centers and included nonthresholded mean transit time (MTT) maps and time-to-peak (TTP) maps with a delay of more than 4 seconds and without thresholding. Other studies have found similar results, supporting the idea that a favorable cerebrovascular physiology may be more important than ischemic duration for extended window therapy.
Trials of IV Desmoteplase
Direct RCT evidence supporting the usefulness of mismatch-based selection was provided by trials of desmoteplase, a thrombolytic agent with high fibrin specificity and a long half-life. In the randomized, placebo-controlled DIAS (Desmoteplase in Acute Ischemic Stroke) trial, patients with OTT within 3 to 9 hours, a perfusion abnormality of 2 cm in diameter involving hemispheric gray matter, and a perfusion/diffusion mismatch of 20% or greater were included and randomized to 62.5 μg/kg, 90 μg/kg, 125 μg/kg, or placebo (part 2, n = 57 patients). Mismatch volumes were assessed by visual estimation. Compared with placebo (n = 27), the 125-μg/kg desmoteplase dose (n = 15) resulted in a higher reperfusion rate on MR imaging performed after 4 to 8 hours (71.4% vs 19.2%, P = .001) and higher rates of 90-day favorable clinical outcome (60% vs 22.2%, P = .009). As in other studies, OTT interval was not associated with reduced treatment effect. In a similar study design, the DEDAS (Dose Escalation of Desmoteplase for Acute Ischemic Stroke) trial confirmed the clinical benefit of the 125-μg/kg desmoteplase dose relative to placebo in patients who fulfilled all MR imaging selection criteria, without a difference in sICH rate or mortality.
The Clinical Response of Mismatch Patients to Reperfusion
The beneficial clinical response of mismatch patients to documented reperfusion was shown in DEFUSE (Diffusion and Perfusion Imaging Evaluation for Understanding Stroke Evolution), a phase II study examining 74 consecutive patients treated with IV tPA between 3 and 6 hours after stroke onset. Treatment selection was based on NCCT, not on an MR imaging mismatch. A total of 1020 patients were screened for 74 patients (7.2%). Patients with a baseline perfusion/diffusion mismatch (mismatch ratio ≥20%, and absolute mismatch ≥10 mL) who underwent early reperfusion (>30% reduction in perfusion lesion volume on MR imaging performed 3–6 hours after IV tPA treatment) had better outcomes than mismatch patients without reperfusion (odds ratio [OR] 5.4; 95% confidence interval [CI] 1.1–25.8). In addition, patients without a perfusion/diffusion mismatch did not seem to benefit from early reperfusion, although there were only 11 patients without a mismatch in the study. Penumbral imaging used T max with a 2-second delay.
The Limitations of the Mismatch Approach
Despite the favorable data in support of the mismatch hypothesis, there is no conclusive evidence that the mismatch alone identifies patients who respond to thrombolysis. This uncertainty was recently highlighted by the results of the phase III DIAS-2 study, which failed to confirm the DIAS and DEDAS findings. In this study, patients with stroke with a 20% visual mismatch on either MR imaging or CT perfusion were randomized in a 1:1:1 fashion to 90 μg/kg IV desmoteplase, 125 μg/kg IV desmoteplase, and placebo. Among the low-dose, high-dose, and placebo groups, respectively, the rates of favorable clinical outcome at 90 days were 47%, 36%, and 46%; the rates of sICH were 3.5%, 4.5%, and 0%; and the mortality was 11%, 21%, and 6%. One-third of enrolled patients were evaluated with CT perfusion.
In addition, EPITHET (the Echoplanar Imaging Thrombolytic Evaluation Trial), a phase II placebo-controlled, randomized trial of IV tPA administered 3 to 6 hours after stroke onset, failed to show any significant difference in the primary end point of infarct growth, or in reperfusion and clinical outcomes, between patients with a perfusion/diffusion mismatch (perfusion/diffusion ratio >1.2, and absolute mismatch volume ≥10 mL) and those without a mismatch, for both the IV tPA and placebo groups. As in DEFUSE, MR imaging findings were not used for patient selection or treatment allocation. These results are consistent with previous studies that have shown that the perfusion/diffusion mismatch does not predict infarct growth, and that lesion growth is equally common in patients with and without a mismatch.
A recent meta-analysis of the DEDAS, DIAS, DIAS II, DEFUSE, and EPITHET trials analyzed the data from 502 mismatch patients who were treated with IV thrombolysis or placebo beyond 3 hours. Although patients with an imaging mismatch showed higher rates of reperfusion with thrombolysis (adjusted OR [a-OR] 3.0; 95% CI 1.6–5.8), there was no significant improvement in favorable clinical outcome by thrombolytic therapy (a-OR 1.3; 95% CI 0.8–2.0). Furthermore, there was a significant increase in mortality and sICH after treatment, but these end points did not remain significant after exclusion of abandoned desmoteplase doses. These negative results suggest that the clinical and tissue response to reperfusion therapy may not be dependent strictly on the presence of a mismatch as it is currently defined.
Using diffusion MR imaging to select patients for IV thrombolysis
Numerous studies have examined the usefulness of diffusion MR imaging for selecting patients for IV therapies outside the 3-hour window. The major selection criterion has been a volumetric mismatch between the perfusion and diffusion imaging lesions ( Fig. 4 ). This mismatch serves as an imaging surrogate of penumbral tissue.
Evidence Supporting the Mismatch Hypothesis
Until the ECASS-3 (European Cooperative Acute Stroke Study 3) results, previous studies that used NCCT-based selection failed to extend the time window for IV tPA beyond 3 hours. ATLANTIS (Alteplase Thrombolysis for Acute Noninterventional Therapy in Ischemic Stroke), ECASS, and ECASS-2 could not show a clinical benefit that outweighed treatment risk in the 5-hour to 6-hour window. In comparison, multiple studies using MR imaging perfusion/diffusion mismatch have shown positive clinical results for extended window treatment with IV tPA, and provide the strongest evidence for this imaging approach.
In large multicenter studies, patient selection using the perfusion/diffusion mismatch was shown to safely and effectively extend the treatment window for IV tPA up to 6 hours. The most frequently used mismatch definition was a perfusion lesion at least 20% larger than the diffusion lesion. Furthermore, patients were excluded if they had extensive infarcts greater than one-third or one-half of the MCA territory. Patients with a perfusion/diffusion mismatch treated with IV tPA beyond 3 hours appeared to do just as well as patients treated within the 3-hour window using traditional NCCT criteria. In a multicenter study of 1210 patients, there were no significant differences in favorable 3-month clinical outcome (modified Rankin scale [mRS] score 0–1; 35.4% vs 40.0%), symptomatic intracerebral hemorrhage (sICH) (5.3% vs 4.4%), and mortality (13.7% vs 13.3%) between patients treated within 3 hours using NCCT versus those treated beyond 3 hours based on a 20% perfusion/diffusion mismatch. Onset-to-treatment time (OTT) was not a predictor of safety or efficacy in univariate or multivariate analysis. The perfusion imaging techniques were heterogeneous among the centers and included nonthresholded mean transit time (MTT) maps and time-to-peak (TTP) maps with a delay of more than 4 seconds and without thresholding. Other studies have found similar results, supporting the idea that a favorable cerebrovascular physiology may be more important than ischemic duration for extended window therapy.
Trials of IV Desmoteplase
Direct RCT evidence supporting the usefulness of mismatch-based selection was provided by trials of desmoteplase, a thrombolytic agent with high fibrin specificity and a long half-life. In the randomized, placebo-controlled DIAS (Desmoteplase in Acute Ischemic Stroke) trial, patients with OTT within 3 to 9 hours, a perfusion abnormality of 2 cm in diameter involving hemispheric gray matter, and a perfusion/diffusion mismatch of 20% or greater were included and randomized to 62.5 μg/kg, 90 μg/kg, 125 μg/kg, or placebo (part 2, n = 57 patients). Mismatch volumes were assessed by visual estimation. Compared with placebo (n = 27), the 125-μg/kg desmoteplase dose (n = 15) resulted in a higher reperfusion rate on MR imaging performed after 4 to 8 hours (71.4% vs 19.2%, P = .001) and higher rates of 90-day favorable clinical outcome (60% vs 22.2%, P = .009). As in other studies, OTT interval was not associated with reduced treatment effect. In a similar study design, the DEDAS (Dose Escalation of Desmoteplase for Acute Ischemic Stroke) trial confirmed the clinical benefit of the 125-μg/kg desmoteplase dose relative to placebo in patients who fulfilled all MR imaging selection criteria, without a difference in sICH rate or mortality.
The Clinical Response of Mismatch Patients to Reperfusion
The beneficial clinical response of mismatch patients to documented reperfusion was shown in DEFUSE (Diffusion and Perfusion Imaging Evaluation for Understanding Stroke Evolution), a phase II study examining 74 consecutive patients treated with IV tPA between 3 and 6 hours after stroke onset. Treatment selection was based on NCCT, not on an MR imaging mismatch. A total of 1020 patients were screened for 74 patients (7.2%). Patients with a baseline perfusion/diffusion mismatch (mismatch ratio ≥20%, and absolute mismatch ≥10 mL) who underwent early reperfusion (>30% reduction in perfusion lesion volume on MR imaging performed 3–6 hours after IV tPA treatment) had better outcomes than mismatch patients without reperfusion (odds ratio [OR] 5.4; 95% confidence interval [CI] 1.1–25.8). In addition, patients without a perfusion/diffusion mismatch did not seem to benefit from early reperfusion, although there were only 11 patients without a mismatch in the study. Penumbral imaging used T max with a 2-second delay.
The Limitations of the Mismatch Approach
Despite the favorable data in support of the mismatch hypothesis, there is no conclusive evidence that the mismatch alone identifies patients who respond to thrombolysis. This uncertainty was recently highlighted by the results of the phase III DIAS-2 study, which failed to confirm the DIAS and DEDAS findings. In this study, patients with stroke with a 20% visual mismatch on either MR imaging or CT perfusion were randomized in a 1:1:1 fashion to 90 μg/kg IV desmoteplase, 125 μg/kg IV desmoteplase, and placebo. Among the low-dose, high-dose, and placebo groups, respectively, the rates of favorable clinical outcome at 90 days were 47%, 36%, and 46%; the rates of sICH were 3.5%, 4.5%, and 0%; and the mortality was 11%, 21%, and 6%. One-third of enrolled patients were evaluated with CT perfusion.
In addition, EPITHET (the Echoplanar Imaging Thrombolytic Evaluation Trial), a phase II placebo-controlled, randomized trial of IV tPA administered 3 to 6 hours after stroke onset, failed to show any significant difference in the primary end point of infarct growth, or in reperfusion and clinical outcomes, between patients with a perfusion/diffusion mismatch (perfusion/diffusion ratio >1.2, and absolute mismatch volume ≥10 mL) and those without a mismatch, for both the IV tPA and placebo groups. As in DEFUSE, MR imaging findings were not used for patient selection or treatment allocation. These results are consistent with previous studies that have shown that the perfusion/diffusion mismatch does not predict infarct growth, and that lesion growth is equally common in patients with and without a mismatch.
A recent meta-analysis of the DEDAS, DIAS, DIAS II, DEFUSE, and EPITHET trials analyzed the data from 502 mismatch patients who were treated with IV thrombolysis or placebo beyond 3 hours. Although patients with an imaging mismatch showed higher rates of reperfusion with thrombolysis (adjusted OR [a-OR] 3.0; 95% CI 1.6–5.8), there was no significant improvement in favorable clinical outcome by thrombolytic therapy (a-OR 1.3; 95% CI 0.8–2.0). Furthermore, there was a significant increase in mortality and sICH after treatment, but these end points did not remain significant after exclusion of abandoned desmoteplase doses. These negative results suggest that the clinical and tissue response to reperfusion therapy may not be dependent strictly on the presence of a mismatch as it is currently defined.
Alternative approaches to imaging-based selection
There are several challenges to the mismatch approach. First, the 20% mismatch threshold is an arbitrary value. Post hoc analyses of DEFUSE and EPITHET have found more optimal definitions, but these require prospective validation. Second, perfusion imaging methods and parameters are heterogeneous among different centers, a factor that has been shown to affect the size of the mismatch. Third, mismatch assessment is based on visual estimation, which suffers from a high degree of error. However, the most fundamental problem with the mismatch approach is that it provides a nonspecific assessment of stroke physiology.
Because a mismatch ratio is a relative measure, the same ratio can equate to different absolute tissue volumes and hence represent varying stroke severities. Despite identical imaging selection criteria for DIAS, DEDAS, and DIAS-2, baseline strokes in DIAS-2 were less severe across all study groups (average National Institutes of Health Stroke Scale [NIHSS] score of 9 vs 12 in DIAS and DEDAS). Similarly, diffusion lesion volumes and absolute mismatch volumes were significantly smaller in DIAS-2, despite the higher relative mismatch volume, and the rate of vessel occlusion was lower. The less severe stroke population in DIAS-2 helps to explain the high placebo response rate that contributed to the failure of this study. Likewise, despite similar imaging criteria between DEFUSE and EPITHET (eg, T max threshold of 2 seconds), the DEFUSE cohort had lower baseline NIHSS scores (11.5 vs 13) and smaller baseline diffusion (10 vs 21 mL) and perfusion (48 vs 192 mL) lesion volumes.
To address this problem, approaches based on absolute lesion volume have been proposed. In support of this concept, a post hoc analysis of EPITHET recently showed that diffusion and perfusion lesion volumes influence clinical response to IV tPA, whereas mismatch ratios do not. This finding is reasonable when one considers that a small territory at risk likely results in a favorable clinical outcome even if the perfusion abnormality is 20% larger than the core infarct defined by diffusion imaging. Similarly, a significant neurologic deficit caused by a large core infarct is not influenced by improved perfusion in the surrounding tissue. It is the size and eloquence of the infarct and the territory at risk that are critical to outcome. Diffusion MR imaging must be analyzed in the context of other important stroke-related variables, including the level of vessel occlusion and the clinical deficit.
The Importance of Vessel Imaging: Is There a Proximal Artery Occlusion Causing a Significant Stroke Syndrome?
When a patient presents with a significant clinical stroke syndrome reflected in an NIHSS score of 10 or greater, vessel imaging represents a critical early step in patient management because it quickly identifies patients who harbor a proximal artery occlusion (PAO) ( Box 1 ). Using clinical stroke severity alone to triage patients to vascular imaging is limited by a high degree of overlap in NIHSS scores between patients with and without PAO. Therefore, it is recommended that noninvasive vessel imaging with CT or MR angiography should be performed on all patients with suspected stroke or transient ischemic attack.
Rationale for vessel imaging:
- •
There is significant overlap in NIHSS scores between patients with and without a proximal occlusion.
- •
Vessel imaging is a screening test for endovascular therapy: terminal internal carotid artery (ICA), MCA M1 or proximal M2 segment, and vertebrobasilar occlusions are the targets for IAT.
- •
Vessel status provides important prognostic information: more proximally located occlusions, particularly terminal ICA occlusions, have the worst outcomes following reperfusion therapy.
- •
Imaging Approach:
- •
CT angiography (CTA) is the best noninvasive test, and has high accuracy for detecting proximal intracranial artery occlusions. Maximum intensity projection images facilitate lesion detection.
- •
MR angiography performs reasonably well in detecting major vessel occlusion, but is prone to patient motion and flow-related artifact. It is less sensitive for detecting M2 occlusions.
- •
Recommendations:
- •
Noninvasive vessel imaging should be performed in all patients with suspected ischemic stroke.
- •
CTA is the test of choice.
- •
Imaging of the cervical vessels is helpful to detect steno-occlusive disease that may be the embolic source and that may need to be addressed during the endovascular procedure (eg, carotid stent placement).
- •
The presence of a proximal occlusion is associated with significantly worse clinical outcomes. In a large prospective study of CTA in 735 patients with stroke or TIA at 2 major academic centers (the STOPStroke study ), patients with PAO, particularly involving the ICA, basilar artery, or MCA M1 and M2 segments had worse 6-month functional outcomes (OR 0.33; 95% CI 0.24–0.45) and higher mortality (OR 4.5; 95% CI 2.7–7.3) than those with normal CTA findings ( Fig. 5 ). ICA or basilar artery occlusion was an independent predictor of functional outcomes (OR 0.44, P = .04). Another study of 480 patients showed that patients with major strokes secondary to PAO classified according to the Boston Acute Stroke Imaging Scale (BASIS) accounted for all the deaths, had longer ICU and hospital stays, and were more likely to be discharged to a rehabilitation facility (all outcomes, P <.0001). Although major strokes comprised less than one-third of the cohort, they accounted for 60% of the total in-hospital costs.
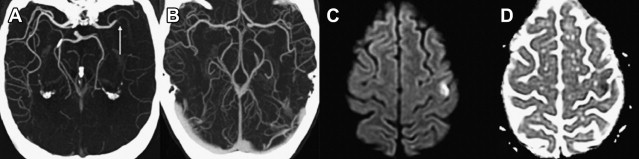
On the other hand, if no arterial occlusion is seen, the prognosis is much improved. In a study of 35 patients with acute stroke who underwent MR angiography in the first 24 hours, the average amount of infarct growth at 2 to 4 days in patients without a visible occlusion was only 1.7 cm 3 . Another study examined 283 consecutive patients with stroke who underwent catheter angiography for intended IAT. The investigators found 28 patients without a visible occlusion, of whom 21 (75%) had a good 3-month outcome (mRS score ≤ 2). Twenty-seven patients had follow-up imaging: 5 normal scans, 8 lacunar strokes, 2 striatocapsular strokes, and 12 small/medium infarcts.
The precise location of the occlusion provides further information regarding prognosis and response to therapy. In general, arterial occlusions that arise more proximally are associated with worse outcomes, with terminal ICA occlusions having the poorest outcomes among anterior circulation strokes. A major reason is that such occlusions are associated with greater clot burden, which in turn hinders the degree and speed of recanalization for both IV and IA therapies. In 1 study of 135 patients with AIS who underwent IAT, patients with large thrombus (>2 vessel diameters) had lower rates of recanalization (50% vs 61%, P = .22) with significantly longer times to recanalization (median, 113 vs 74 minutes, P <.001). Large thrombus was an independent predictor of poor outcome (OR 2.4; 95% CI 1.06–5.57) and mortality (OR 4.0; 95% CI 1.2–13.2).
Furthermore, the territory at risk expands with occlusion of more proximal branch points. As one would expect, a larger amount of lesion growth is observed with M1 (MCA stem) versus M2 (post-MCA bifurcation) occlusions, despite similar recanalization rates. In addition, within the M1 segment itself, a proximal clot occludes the lenticulostriate arteries, leading to early infarct extension into the basal ganglia. For terminal ICA occlusions, there are 2 factors that promote worse outcomes: involvement of both the anterior cerebral artery (ACA) and MCA territories, and a marked impairment of the collateral circulation. Occlusion of the ACA A1 (precommunicating) segment results in a variable reduction of flow into the distal ACA territory, which is dependent on the caliber of the anterior communicating artery (ACOM) and the contralateral A1 segment. An isolated hemisphere is rare, and leads to rapid infarction of the ACA and MCA territories ( Fig. 6 ). However, even with the presence of an ACOM, in many cases the occlusion of antegrade flow through the ipsilateral A1 segment diminishes the perfusion pressure through the pial collaterals, resulting in greater and more rapid infarct growth in the MCA territory. This finding was reported in 1 study of 49 patients with AIS who underwent MR imaging/MR angiography within 6 hours of stroke onset and before IV tPA treatment. Of the 47 patients with documented vessel occlusions, patients with ICA occlusions (n = 12) had significantly larger mean pretreatment diffusion lesion volumes (84.8 ± 75.2 cm 3 vs 32 ± 41.6 cm 3 ) than those with M1 occlusions (n = 19). In addition, patients with ICA occlusions had significantly larger perfusion defects on pretreatment TTP maps (161.2 ± 38.9 cm 3 vs 127.5 ± 40.9 cm 3 ). Moreover, it has been shown that relative infarct growth is greater for terminal ICA occlusions than for M1 occlusions after normalizing for the absolute volume of hypoperfusion. Numerous imaging studies have confirmed these findings.
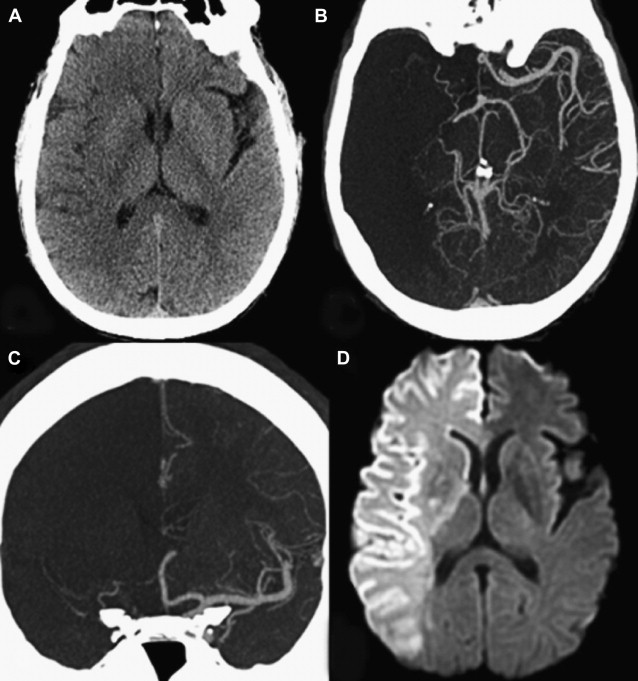
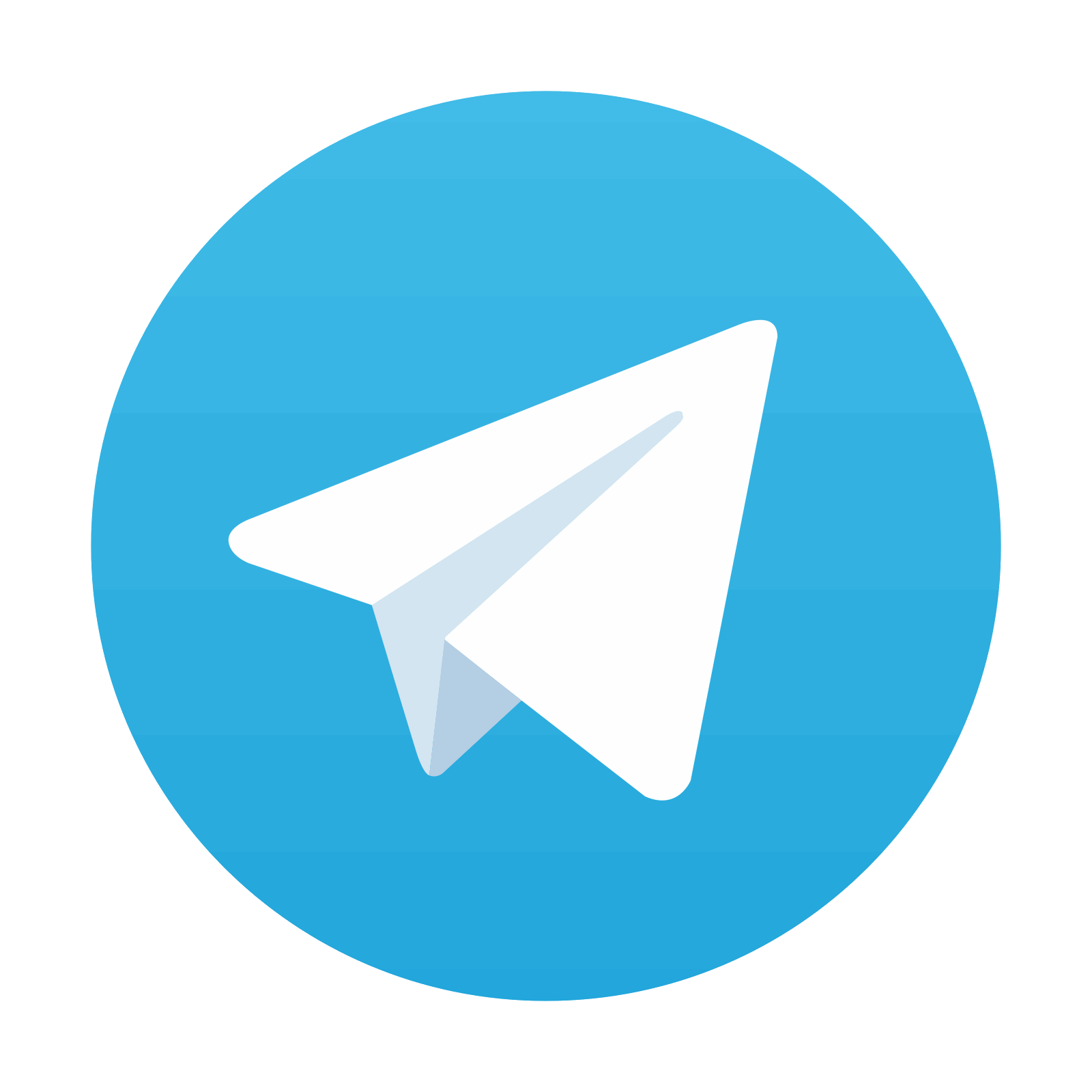
Stay updated, free articles. Join our Telegram channel

Full access? Get Clinical Tree
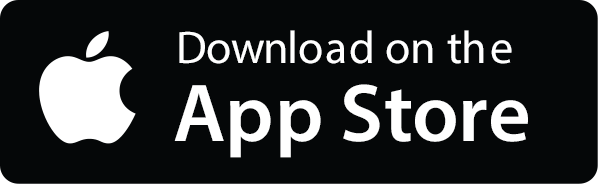
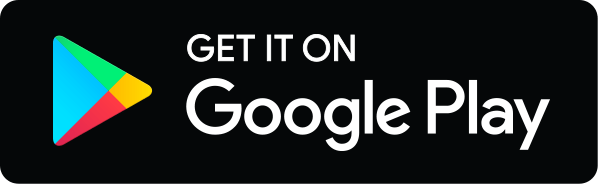
