Source of Funding
The authors received no outside funding for the work presented in this chapter.
Conflict of Interest
The authors have no conflicts of interest related to the subject matter presented in this chapter.
Introduction
Trauma to the upper cervical spine can result in a spectrum of pathology, ranging from benign to catastrophic. Upper cervical spine fractures account for nearly 70% of all cervical injuries in elderly adults and more than 35% of cervical injuries in young adults resulting in significant neurologic damage, chronic pain, and serious disability. Proper diagnosis, stabilization, and definitive management of upper cervical spine injuries are critical to avoid short- and long-term mortality and morbidity. Over the last several decades, numerous classification schemes have been developed to assist clinicians and surgeons in proper medical and surgical decision-making. This chapter aims (1) to present the various classification systems for describing upper cervical spine injuries, (2) to discuss the clinical correlates associated with each classification, specifically management of upper cervical spine injuries, and (3) to critique the classification based upon literature review.
Understanding the clinical implications of upper cervical spine injuries requires a robust understanding of the craniovertebral junction and upper cervical spine anatomy ( Fig. 1 ). The atlantooccipital joint is ellipsoid or kidney bean in shape and consists of the occipital condyles superiorly and the superior facet of C1 lateral mass inferiorly. The joint is supported by ligamentous craniocervical structures connecting the borders of the foramen magnum with the arches of the atlas. The ligamentous anatomy of this joint is critical in maintaining stability and can be divided into intrinsic and extrinsic structures.
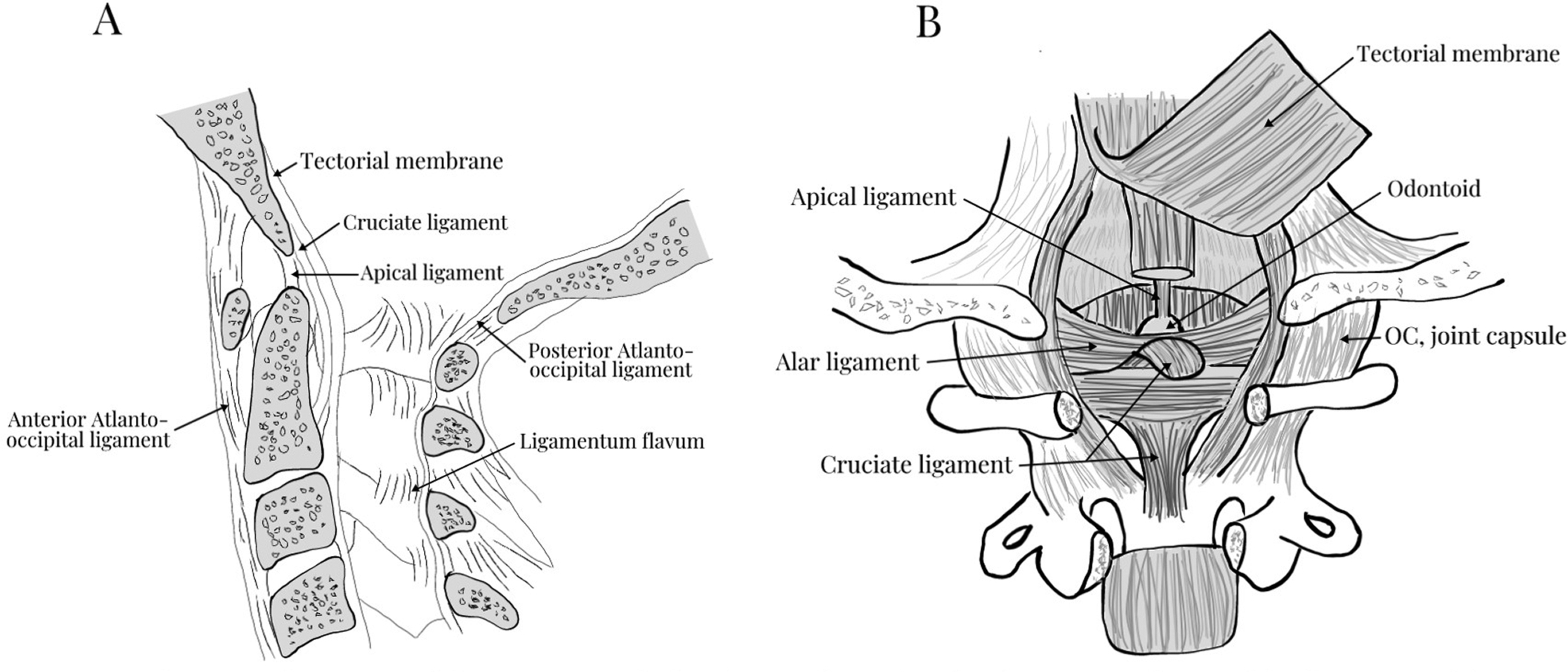
The extrinsic ligaments include the anterior atlantooccipital membrane, which is the broad and dense continuation of the anterior longitudinal ligament (ALL) and the posterior atlantooccipital ligament, which is a broad and thin continuation of the ligamentum flavum. The intrinsic ligaments originate from the posterior aspect of the anterior ring of C1 and the odontoid process with three layers of ligamentous structures providing the bulk of support to the craniovertebral articulation. The apical and alar ligaments, commonly referred to as the odontoid ligaments, form the anterior- most layer. The apical ligament is a relatively weak midline structure extending from the odontoid process to the anterior margin of the foramen magnum. The alar ligaments are a pair of thick cord-like structures extending anterolaterally from the odontoid process to the occipital condyles to limit extreme atlantoaxial rotation. In the middle lay the transverse ligament, which connects the posterior odontoid of C2 to the anterior arch of C1. Most posterior in this ligamentous bundle is the tectorial membrane, which is a broad, strong band-like continuation of the posterior longitudinal ligament (PLL) connecting the odontoid body to the anterior foramen magnum. These intrinsic ligaments provide the primary constraint to extreme flexion (i.e., bony atlantooccipital articulation), extension (i.e., tectorial membrane), rotation and lateral flexion (i.e., alar ligaments), and distraction (i.e., tectorial membrane and alar ligaments).
Occipital Condyle Fracture
Introduction
In 1817, Sir Charles Bell provided the first scientific report of a patient with an occipital condyle fracture (OCF). Per his account, a man fell backward off a 5-ft wall, striking his head. The man recovered in the hospital, seeming to have experienced only a minor concussion. However, when he was preparing to leave the hospital, he turned his head in gathering his belongings and lost consciousness and died. The autopsy revealed a displaced occipital condyle fragment compressing the medulla oblongata. Historically, the presence of occipital condyle fractures has been difficult to diagnose owing to the low visibility on plain radiographic imaging of the skull and cervical spine. Now, however, with the increased availability of computed tomography (CT) and widespread use of CT in the standard trauma protocols for suspected craniocervical injury, we know that OCF injuries are more common than once thought. Although the exact prevalence of OCF is unknown, the incidence is estimated to be up to 16% among patients suffering severe craniocervical injury and between 0.1% and 0.4% of all trauma patients. Additionally, more than 20% of patients with OCF injuries have associated cervical spine injuries, primarily at C1 and C2. Despite the rarity of OCF injuries, prompt evaluation, identification, and management of these injuries are imperative, as delayed diagnosis and treatment may be catastrophic.
Clinical presentation of patients with occipital condyle fractures is variable and largely dependent upon severity and presence of concomitant vascular, cranial, or brain stem injury. Other than a history of trauma, general high cervical neck pain, or loss of consciousness, patients may suffer lower cranial nerve injury known as Collet-Sicard syndrome. Symptoms include difficulty swallowing and hoarseness (CN XI or glossopharyngeal nerve and CN X or vagus nerve), difficulty shrugging shoulders (CN XI or cranial nerve), or difficulty chewing or talking due to tongue weakness (CN XII or hypoglossal nerve). Occasionally, the presence of retropharyngeal hematoma, often seen on advanced imaging, may be the only indication that a craniovertebral insult has occurred. A number of classifications have been devised to describe and categorize occipital condyle fractures based upon fracture morphology, soft tissue injury, or pattern of instability.
Anderson-Montesano Classification
In 1988, Paul Anderson and Pasquale Montesano devised an OCF classification based upon fracture morphology, pertinent anatomy, and biomechanics of OCF injuries in a total of 26 patients ( Fig. 2 ). Their classification is as follows:
- •
Type I: Impacted occipital condyle fracture
- •
Results from axial loading of the skull onto C1 and compression of the condyle onto the superior facet of the C1 lateral mass
- •
Occipital condyle demonstrates comminution with little or no displacement
- •
Ipsilateral alar likely intact given minimal fragment displacement
- •
Generally a stable injury if intact contralateral alar ligament and tectorial membrane with minimal or no displacement of ipsilateral condyle fragments
- •
- •
Type II: Basilar skull fracture that exits through occipital condyle(s)
- •
Results from a direct blow to the skull
- •
Occipital condyle demonstrates fracture line exiting into the foramen magnum
- •
Fragment is generally nondisplaced or minimally displaced
- •
Alar ligament likely intact given minimal or no displacement of condyle fragment
- •
Generally a stable injury if intact contralateral alar ligament and tectorial membrane with minimal or no displacement of condyle fragment
- •
- •
Type III: Avulsion fracture of occipital condyle
- •
Results from excessive occipitocervical rotation or lateral bending
- •
Occipital condyle demonstrates fractured fragment displaced toward odontoid tip indicating occipitocervical dissociation
- •
Ipsilateral alar ligament is compromised, and contralateral alar ligament and tectorial membrane are loaded
- •
Generally an unstable injury given the incompetent ipsilateral alar ligament complex which leads to overloading of the contralateral alar ligament and tectorial membrane
- •
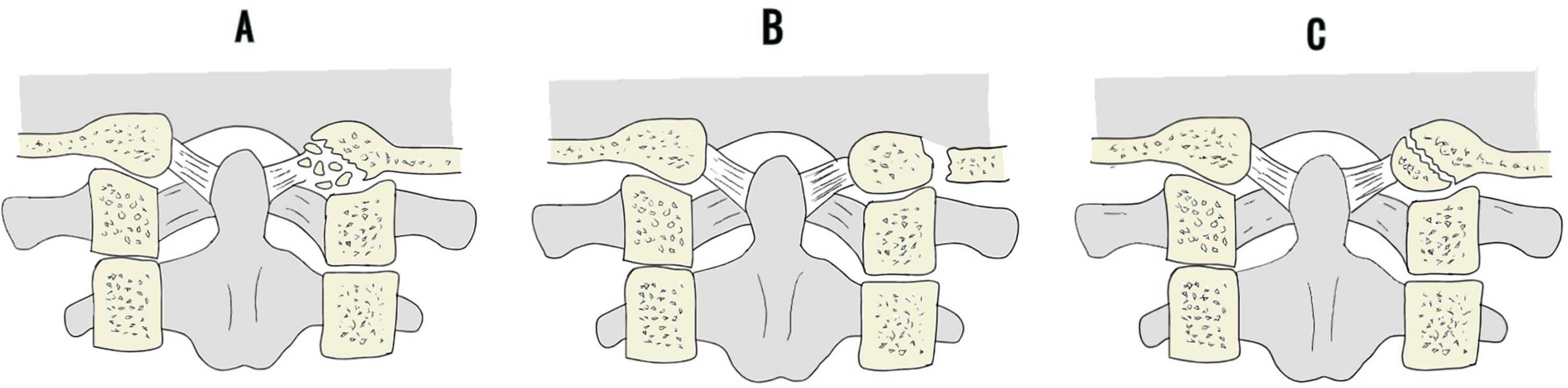
Tuli et al. Classification
Although the Anderson-Montesano classification of OCF injuries is touted as the first major classification that associates fracture pattern with instability, the extent to which it accurately predicted stability and directed treatment strategy is controversial. In 1997, Tuli et al. attempted to address these shortcomings by introducing a new classification scheme based upon the degree of ligamentous injury based upon CT and overall occipital-atlantoaxial instability as determined by X-ray and MRI. They retrospectively reviewed 3 cases from their home institution and 96 cases reported in the literature to devise three specific endpoints of their treatment algorithm. The Tuli et al. classification is as follows:
- •
Type I: Stable, nondisplaced occipital condyle fracture
- •
Diagnosed with CT only, no XR or MRI required
- •
No immobilization required
- •
- •
Type II: Displaced, stable occipital condyle fracture
- •
CT/XR without evidence of O-C1-C2 instability*
- •
Hard collar required
- •
- •
Type III: Displaced, unstable occipital condyle fracture
- •
CT/XR with evidence of O-C1-C2 instability*
- •
Halo vest or surgical fixation required
- •
- •
*Criteria of O-C1-C2 instability as derived from biomechanical analyses of spinal instability by White and Panjabi include any one of the following :
- •
CT/XR criteria of O-C1-C2 instability
- •
> 8-degree axial rotation of O-C1 to one side
- •
> 1 mm of O-C1 translation
- •
> 7 mm of overhang of C1 on C2
- •
> 45 degrees of axial rotation of C1-C2 to one side
- •
> 4 mm of C1-C2 translation
- •
< 13 mm distance between the posterior body of C2 to C1 posterior ring
- •
Avulsed transverse ligament
- •
- •
MR evidence of ligamentous disruption
- •
When comparing the Tuli et al. classification to the Anderson-Montesano classification, it is clear that additional imaging and more stringent criteria for ligamentous instability offer a more specific indication of ligamentous injury. In their retrospective review of 24 patients with OCF injuries, Byström et al. compared multiple OCF classification schemes. They noted certain difficulties with the Anderson-Montesano classification, specifically differentiating Type I versus Type III categorization for three of their patients. Additionally, of the seven patients who were determined to have unstable OCF injuries by Anderson-Montesano, only one of the seven was determined to have unstable OCF patterns by Tuli et al. Although both the Anderson-Montesano and Tuli et al. classifications provide a framework for understanding occipital condyle fractures and risk of ligamentous instability, they are not generally used by spine surgeons. There is disagreement in the literature regarding the definition of fracture displacement, criteria for instability, and management recommendations for stable nondisplaced OCF injuries and of bilateral OCF injuries.
Some suggest that the Anderson-Montesano and Tuli et al. classification schemes are more useful for academic purposes and less important for determining treatment. In their retrospective review of 106 OCFs, Maserati et al. concluded that detailed classification of OCF injuries, beyond the identification of craniocervical misalignment on CT (i.e., O-C1 interval > 2.0 mm ), does not affect the clinical decision-making process. They suggest that OCF injuries showing disruption of the O-C1 joint or neural compression should be treated with fusion surgery, while all others could be treated with a rigid cervical orthosis.
Occipitocervical Instability and Dislocation
Introduction
Instability at the occipitocervical junction portends a serious risk of severe neurologic morbidity and mortality if left undiagnosed and untreated. Although commonly associated with traumatic atlantooccipital dislocation (AOD), more indolent conditions and disorders such as inflammatory arthropathy, rheumatoid arthritis, neoplasm, and infection may lead to instability at the occipitocervical junction. Patients may present with acute neurologic changes, following trauma, or with more progressive neurologic changes and craniocervical deformity, seen in atraumatic cases of instability. Following traumatic injury, AOD often results in an incongruent association between one or both occipital condyles and the paired C1 lateral mass. The most sensitive method for diagnosing traumatic AOD has traditionally been the measurement of the basion axial-basion dens interval on computed tomography (CT). In addition to O-C1 joint dislocation, more than half (~ 55%) of the cases of AOD are associated with atlantoaxial injury, instability, or frank dislocation. MRI may be indicated for the identification of ligamentous disruption and rotational instability of the upper cervical spine. For atraumatic conditions, such as rheumatoid arthritis, CT and MRI may be equally as important for diagnosing O-C1 instability, as RA patients with occipitocervical instability who are treated conservatively generally suffer a poor outcome. With respect to classification schemes, occipitocervical instability has primarily been described in association with trauma.
Traynelis Classification
In 1986, Traynelis et al. published a simple classification for AOD based upon a review of 17 patients who survived more than 48 hours after AOD injury. Type of injury is based primarily upon radiographic and CT findings of displacement or dislocation of the occiput relative to the atlas ( Fig. 3 ). Thresholds for defining the degree of displacement were previously published. Powers et al. discuss the relationship among four bony landmarks of the occipitocervical spine, specifically the ratio of the basion (B) to C1 posterior arch distance (C) and the opisthion (O) to the C1 anterior arch distance (A) ( Fig. 3 A1). A “Powers ratio” greater than 1.0 is indicative of AOD and is most sensitive for detecting anterior dislocations. In rare instances, such as patients with congenital anomalies, a posterior dislocation injury, or a pure distraction injury, the Powers ratio may be less than 1.0 despite the presence of AOD. The Traynelis classification accounts for horizontal displacement as measured by the distance between the mandible to the anterior arch of the atlas and the mandible to the anterior aspect of the odontoid. The standard measurements for each relationship are 2 mm and 10 mm, respectively ( Fig. 3 A2). Lastly, Traynelis et al. included an assessment of longitudinal distraction measured from the occipital condyle to the superior facet of C1 (occipital-C1 interval or CCI). A gap greater than 5 mm in pediatric patients and greater than 2 mm in adult patients indicated AOD. Using these parameters, Traynelis classified AOD as follows ( Fig. 3 B):
- •
Type I: Anterior displacement of the occiput relative to C1
- •
Type II: Longitudinal distraction of the occiput relative to C1
- •
Type III: Posterior displacement of the occiput relative to C1
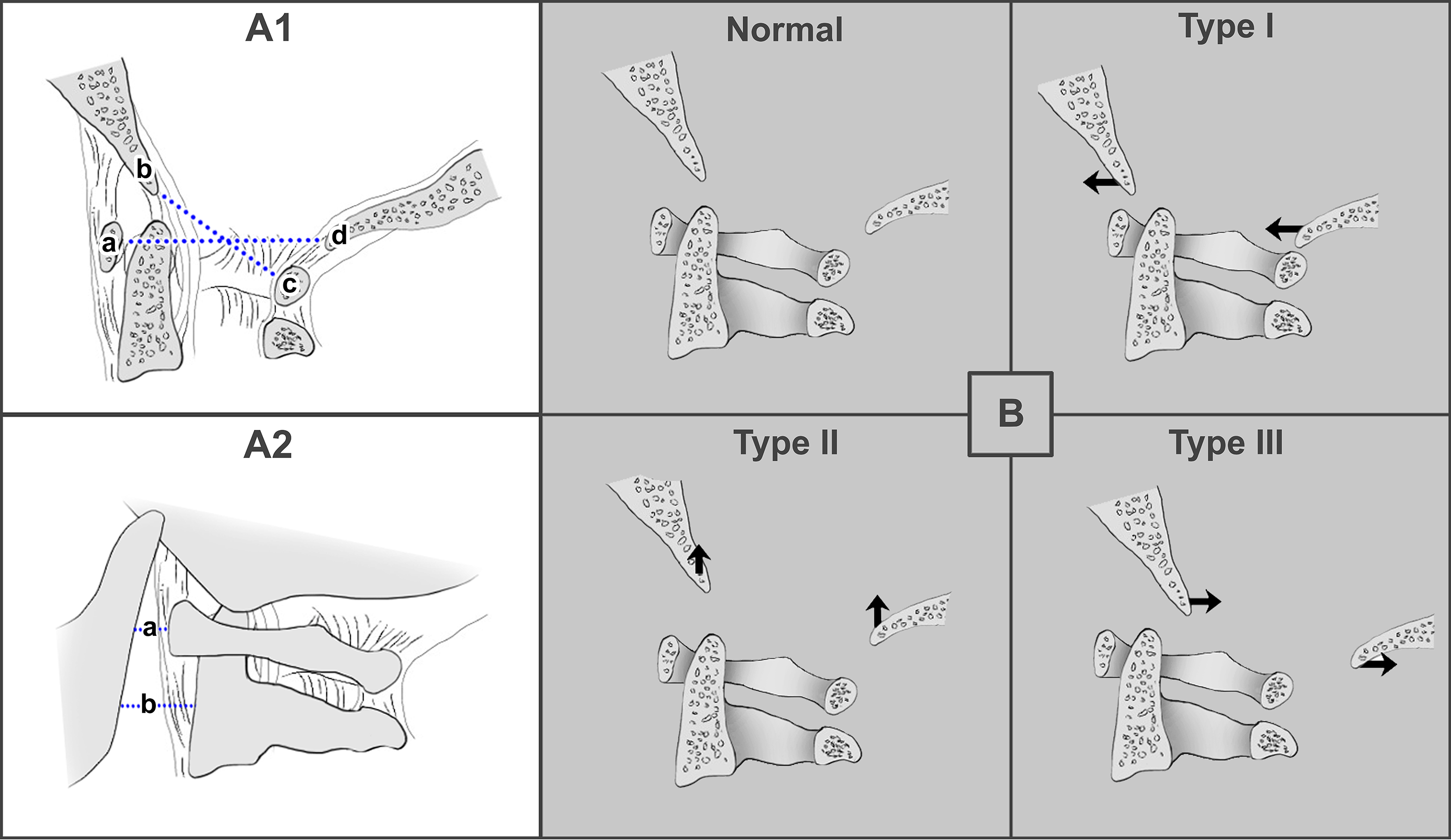
Historically, the purpose of this classification served to describe the type of AOD and to suggest which types of injury may improve with axial traction. Traynelis et al. proposed that longitudinal traction in a Type II AOD may not be indicated, as it would only exacerbate, rather than reduce, the original injury. Instead, the group suggested that longitudinal traction is best used in cases of Type I or Type III AOD injuries. At the time, case reports suggested that cervical traction safely reduced bony structures, restored alignment, and decompressed the cord, resolving major neurologic deficits in Type I and Type III injuries—injuries in which the primary displacement was not longitudinal. More recent literature, however, recommends against cervical traction and instead suggests immediate immobilization, followed by halo fixation and/or occipitocervical fusion. Cervical traction has been shown to increase the risk of neurologic deterioration by nearly 10% in cases of AOD. Aside from the questionable indications for cervical traction, Traynelis’ classification has additionally been critiqued for not accounting for coronal or rotational occipitocervical malalignment. Additionally, without a detailed examination of the ligamentous structures, head positioning during imaging could result in “normal” radiographic parameters despite ligamentous compromise. Lastly, the classification system does not address the severity of the injury.
Harborview Classification of Craniocervical Injury
In 2006, Bellabarba et al. published a new, three-stage classification system that accounts for potential upper cervical ligamentous instability and injury severity. Their group retrospectively analyzed 17 consecutive cases of patients surviving AOD between 1994 and 2002 and found a significant delay between time of presentation and time of proper diagnosis of AOD. This lag time led was associated with a delay to surgical fixation and worse neurologic outcomes compared to those treated acutely. The classification leverages MRI data to determine ligamentous strain or disruption and anatomic measurement, such as the BAI and BDI to determine displacement. Harborview classification of craniocervical injury is as follows:
- •
Stage 1: Stable, minimally or nondisplaced
- •
MRI evidence of injury to craniocervical osseoligamentous stabilizers
- •
Craniocervical alignment within 2 mm of normal
- •
Distraction of ≤ 2 mm on provocative traction radiography
- •
For example, unilateral alar ligament injuries, partial ligamentous strains
- •
- •
Stage 2: Unstable, partially or complete spontaneously reduced
- •
MRI evidence of injury to craniocervical osseoligamentous stabilizers
- •
Craniocervical alignment within 2 mm of normal
- •
Distraction of > 2 mm on provocative traction radiography
- •
- •
Stage 3: Unstable, displaced, or unreduced
- •
Craniocervical malalignment of > 2 mm on static radiography
- •
Stage 1 injuries typically can be treated nonoperatively, while Stage 2 and 3 injuries require rigid internal fixation. One criticism of the classification is that no patients in the author’ series suffered a Stage 1 injury. Therefore, a direct comparison of these diagnostic parameters is not feasible. Another criticism of the Harborview classification is the inclusion of dynamic traction radiography in the diagnostic criteria, particularly for distinguishing between Stage 1 and Stage 2 injury. Data in support of using dynamic traction radiography come primarily from biomechanical studies using cadaver models. Child et al. found that craniocervical traction reliability evaluates instability and requires only 5–10 pounds of traction. Although dynamic traction may be considered the gold standard for diagnosing craniocervical instability, the safety and utility of dynamic traction radiography have not been well studied clinically in the context of acute spine trauma. Additionally, several authors recommend against acute traction given the possibility of further neurologic deterioration.
C1 Fractures
Introduction
Fractures of the first cervical vertebra (C1) or atlas are of low incidence and relatively low risk of neurologic injury when occurring in isolation, comprising 2%–13% of all cervical spine fractures and up to 25% of C1-C2 complex injuries. The distinct osseous and ligamentous anatomy of the atlas renders it a highly mobile, articular ring of the craniovertebral junction (CVJ), facilitating approximately half of the flexion-extension and rotational motion of the cervical axis. This motion occurs at the atlantooccipital and atlantoaxial complexes, respectively. As an integral component to CVJ stability, unique consideration is required in the context of injury and fracture. Atlas fractures most often are the result of an axial load—impact of occipital condyle on atlas ring—with variable secondary vectors of forward flexion, extension, and lateral bending. Albeit risk of neurologic deficit is rare in isolated atlas injuries, appraisal of fracture stability determines the risk of unacceptable cervical dysfunction, delayed deformity, and contributes to treatment selection.
Jefferson, Landells and Van Peteghem, Gehweiler, Levine and Edwards Classification
No single accepted classification of atlas fractures exists. Sir Geoffrey Jefferson, as published in 1919, provided the first complied description of atlas fractures and theorized mechanisms of failure. In a review of 46 atlas fractures—derived from 2 personal cases, 2 dry museum specimens, and 42 published case-reports—Jefferson deduced an anatomical description of atlas fracture patterns, “sites of fracture of the atlas ring.” Later to be attributed as the first atlas fracture classification scheme, Jefferson described the following patterns:
- •
Type I: Posterior arch in isolation
- •
Type II: Anterior arch in isolation
- •
Type III: One or more fractures including both arches
- •
Type IV: Lateral mass or masses in isolation
- •
Type V: Lateral mass or masses and posterior arch
Jeffersońs classification is as notated in Table IV of his original publication, and various iterations of his classification exist in the literature. What is most commonly referred to as a “Jeffersońs fracture” is a Jefferson Type III, also known as an atlas burst fracture given the predominant axial mechanism and pattern of fragment dispersion ( Fig. 4 ). Classifications since Jeffersońs initial description are numerous and include those of Landells and Van Peteghem, Gehweiler et al., and Levine and Edwards. Each system restructures Jeffersońs descriptions with the variable addition of transverse process fractures and primary pattern modifications. Regional preference of classifications is prevalent with Landells and Van Peteghem’s reaching proclivity in the United States and Gehweiler’s being most commonly reported in European literature. In a review of 35 atlas fractures, Landells and Van Peteghem proposed their three-part classification consolidating Jeffersońs descriptions:
- •
Type I: Confined to a single arch, either anterior or posterior.
- •
Type II: One or more fractures including both arches.
- •
Type III: Lateral mass with fracture extension, if present, limited to one arch.
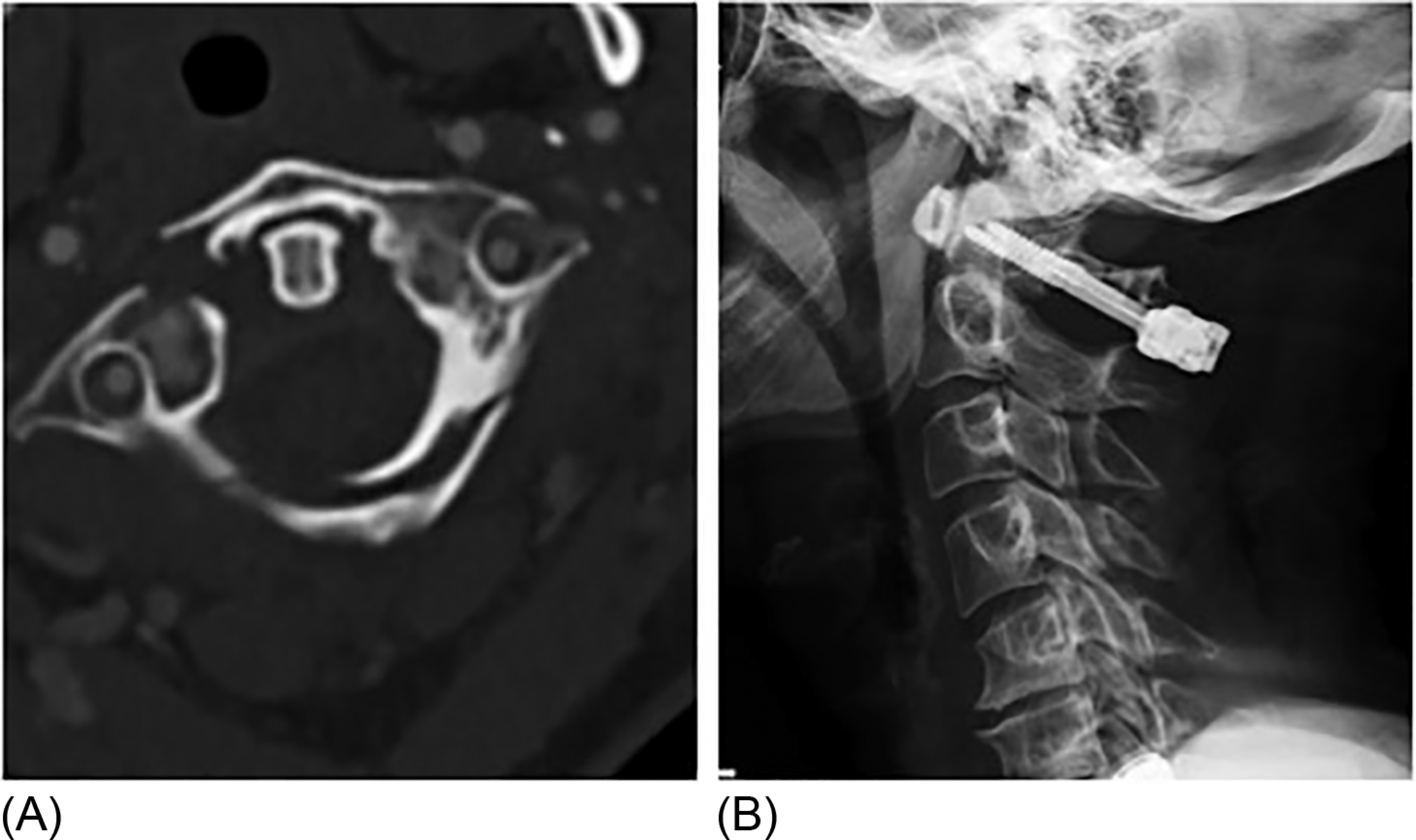
Lateral mass fractures with the involvement of both arches would satisfy the criterion of Landells and Van Peteghem Type II. Landells and Van Peteghem reported an overall C2 fracture incidence of 4.7% among 750 cervical spine injuries comprised of 45.7% Type I, 37.1% Type II, and 17.1% Type III variants. Overall, the variability of prevalence exists among atlas fracture literature with burst fractures, posterior arch, and lateral mass variants accounting for 20%–30% of all fracture descriptions. The Jefferson and Landells and Van Peteghem classifications are simultaneously exhibited within Fig. 5 . Gehweiler’s five-part classification is more analogous to Jeffersońs descriptions with the supplement of transverse process fractures and transverse atlantal ligament assessment:
- •
Type I: Anterior arch in isolation
- •
Type II: Posterior arch in isolation
- •
Type IIIa: Anterior and posterior arch with the integrity of the transverse atlantal ligament
- •
Type IIIb: Anterior and posterior arch with the disruption of the transverse atlantal ligament
- •
Type IV: Lateral mass in isolation
- •
Type V: Transverse process in isolation
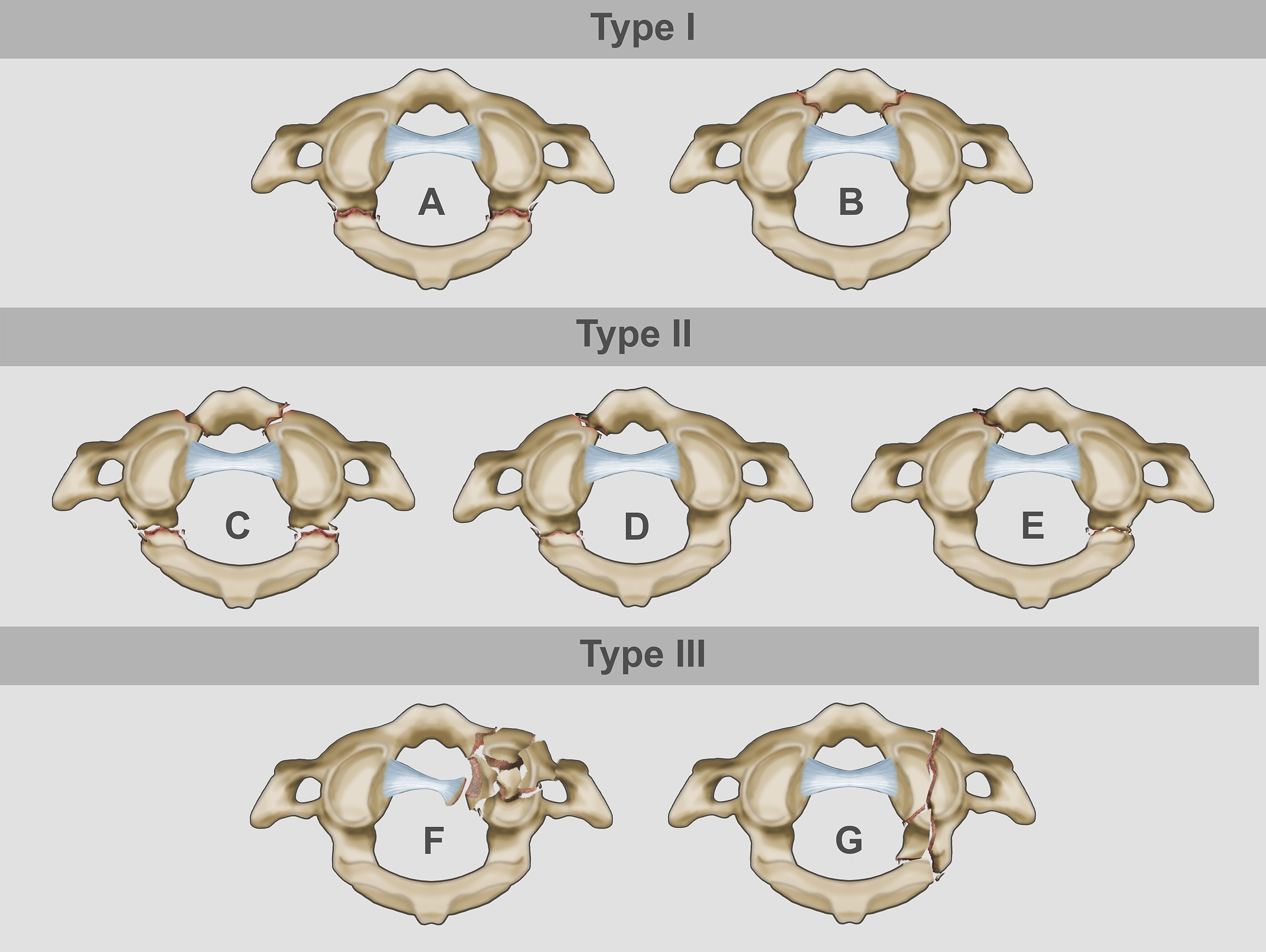
Description of transverse atlantal ligament (TAL) integrity provides management implications contingent on the nature of the ligamentous disruption. TAL assessment, classification, and management considerations will be discussed in the following sections. Although better known for their descriptive contributions to C2 “Hangman” fractures, Levine and Edwards provide the final atlas fracture classification to be discussed. Their proposed primary classification types are as follows:
- •
Type I: Posterior arch in isolation
- •
Type II: Lateral mass in isolation, unless Type VII
- •
Type III: Anterior and posterior arch fractures
- •
Type IV: Avulsion fracture from the anterior arch
- •
Type V: Transverse process fracture
- •
Type VI: Anterior arch fracture, not an avulsion etiology
- •
Type VII: Comminuted unilateral intra-articular lateral mass fracture
Levine and Edwards “Type IV” injuries in contrast to “Type VI” are generally of horizontal morphology and may have incomplete involvement of the anterior arch from longus coli avulsion. In aggregation, these taxonomic arrangements describe most atlas fracture patterns or variants thereof aside from behavioral distinct sagittal lateral mass fractures as described by Bransford et al. Aside from Gehweiler “Type IIIb” injuries, the discussed classifications provide limited practicality for distinction in management. Validation studies are suitably deficient, and their use is limited to descriptive purposes. Table 1 displays each classification organized by anatomic location of fracture for ease of comparison. The AOSpine Upper Cervical Spine Trauma classification details fractures and ligamentous injuries of the entire occipito-atlantoaxial complex (O-C2), and its application and utility will be discussed in the coming sections. In the absence of a ubiquitously accepted fracture classification system, the determination of atlas fracture stability using imaging-based standards guides evaluation and treatment considerations.
