Since the report published in 1968 by Favaloro about the use of saphenous veins to restore coronary artery blood flow in 171 patients, a large number of coronary artery bypass grafting (CABG) procedures have been performed worldwide. In the United States alone, 219,000 patients underwent a total of 397,000 CABG procedures in 2010.
The left internal mammary artery (IMA) is frequently used as an arterial conduit to the left anterior descending (LAD) and its diagonal branches. Other arterial conduits include the right IMA placed to the right coronary artery (RCA) or LAD, the right gastroepiploic artery to the RCA, or the use of free radial artery grafts. Reversed saphenous veins are generally used for grafting to distal branches of the RCA and circumflex coronary artery (LCX) or to diagonal branches of the LAD.
The long-term results of aortocoronary bypass surgery depend largely on the maintenance of graft patency. About 25% of venous grafts occlude within 1 year of surgery, whereas during the following 5 years there is a 2% annual occlusion rate, which increases to 5% yearly thereafter. Thus 50% to 60% of venous grafts are occluded after 10 years and only half of the remaining patent ones have no evidence of atherosclerosis. The mechanisms responsible for venous graft occlusion are believed to be thrombosis in the early weeks after surgery, followed by intimal hyperplasia during the first year and accelerated atherosclerosis in the later stages. Atherosclerotic changes develop comparatively in a smaller percentage of patients with IMA grafts. As a result, in situ arterial grafts occlude less frequently, up to 5% in the first year and 20% to 30% after 10 years, leading to an improved long-term survival.
These graft attrition statistics result in the need for accurate evaluation of bypass graft patency and function, which often is required several times during the lifetime of any given patient.
Imaging Modalities Capable of Evaluating Grafts
Selective x-ray coronary angiography is the gold standard for assessment of graft anatomy and has the added advantage of simultaneous visualization of the native coronary arteries. Use of a Doppler-tipped guidewire during angiography provides hemodynamic information about graft function by assessing flow pattern at rest and after pharmacologically induced hyperemia.
Selective coronary angiography is, however, invasive, uses ionizing radiation, iodine contrast, and has a small risk of complications such as coronary artery dissection, arrhythmia or stroke.
Two-dimensional (2D) Doppler echocardiography is restricted to evaluation of grafts placed on the LAD artery.
Near infrared fluorescence complex angiography and perfusion analysis is a novel real-time imaging technology used to assess the physiologic response to grafting during the intraoperative phase of CABG and which may be useful in predicting subsequent graft failure.
Cardiovascular magnetic resonance (CMR) and coronary computed tomography (CCT) are techniques which allow the direct evaluation of bypass grafts patency. A unique feature of CMR is that in addition to standard anatomic imaging, blood flow velocity and volume can be quantified within the grafts. Thus the true physiologic status of the functional unit represented by a graft and its recipient vessel can be determined noninvasively.
Cardiovascular Magnetic Resonance of Bypass Grafts
Over the years, several CMR techniques have been introduced to evaluate aortocoronary bypass grafts ( Table 26.1 ). The assessment strategy may include either anatomic (angiographic) or hemodynamic (flow volume, velocities, flow reserve) evaluation or a combination of these modalities. This is usually combined in clinical practice with evaluation of the myocardial function (cine CMR), tissue characterization (late gadolinium enhancement imaging for presence of myocardial fibrosis/infarction), and ischemia detection (pharmacologic first pass gadolinium contrast myocardial perfusion).
Reference | Technique | No. of Grafts | Sensitivity (%) | Specificity (%) | Accuracy (%) |
---|---|---|---|---|---|
White et al. | SE CMR | 65 | 91 | 72 | 86 |
Rubinstein et al. | SE CMR | 44 | 92 | 85 | 89 |
Jenkins et al. | SE CMR | 60 | 90 | 90 | 90 |
Frija et al. | SE CMR | 52 | 98 | 78 | 94 |
White et al. | Cine CMR | 28 | 93 | 86 | 89 |
Aurigemma et al. | Cine CMR | 45 | 88 | 100 | 91 |
Galjee et al. | SE CMR | 98 | 98 | 85 | 96 |
Cine CMR | 98 | 88 | 96 | ||
Combined | 98 | 76 | 94 | ||
Kessler et al. | 3D navigator | 19 | 87 | 100 | 89 |
Vrachliotis et al. | 3D CE MRA, ECG-triggered | 44 | 93 | 97 | 95 |
Wintersperger et al. | 3D CE MRA Non–ECG triggered | 76 | 95 | 81 | 92 |
Kalden et al. | HASTE | 59 | 95 | 93 | 95 |
3D CE MRA ECG-triggered | 93 | 93 | 93 | ||
Bunce et al. | SSFP | 79 | 84 | 45 | 78 |
3D CE MRA ECG-triggered | 85 | 73 | 84 | ||
Langerak et al. | 3D navigator Two observers | 56 | 65–83 | 80–100 | ~80 |
Whereas pulse sequences developed for imaging native coronary arteries are also applied for imaging bypass grafts, CABG imaging is associated with specific problems (different vessel anatomy and flow patterns and the presence of metallic vascular clips, ostial markers and sternal wires).
A majority of published clinical CMR studies addressed imaging the proximal portion of vein grafts. Proximal segments are less affected by bulk cardiac motion compared with distal graft segments or native coronary arteries, resulting in fewer motion artifacts, whereas the lack of direct contact with epicardial fat or myocardium results in higher contrast to surrounding tissues. Unfortunately, graft stenosis often occurs at the site of anastomosis with the native vessel where CMR encounters artifacts and resolution problems similar to those in imaging the native coronary arteries. Arterial grafts imaging poses additional problems because of vessel tortuosity and smaller caliber as well as presence of metallic artifacts from hemostatic clips and sternal wires.
Bypass Graft Anatomic Imaging Techniques
Conventional Spin Echo and Gradient Echo Imaging
The assessment of saphenous vein aortocoronary bypass graft patency has been one of the early indications for CMR. More than two decades ago several groups reported the feasibility of assessing graft patency using conventional electrocardiogram (ECG)-triggered multislice spin-echo techniques. On spin-echo images, patent grafts with good blood flow appear in consecutive imaging planes as conduits with a signal void. In contrast, stenotic grafts with slow flow or occluded grafts display intermediate signal intensity ( Fig. 26.1 ). With selective x-ray angiography as the method of reference, the sensitivity of spin-echo CMR in predicting graft patency ranged from 90% to 98% with a specificity of 72% to 90%.
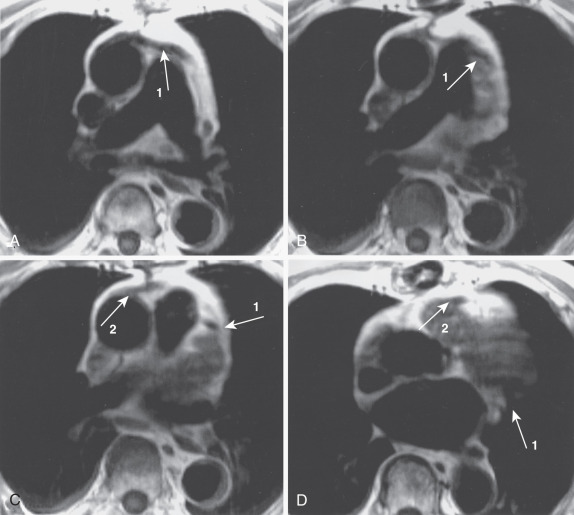
Using conventional gradient echo nonbreath-hold CMR, with a relatively long echo time (TE) and repetition time (TR), the sensitivity was in the same order of magnitude (88%–98%), with a somewhat higher specificity (86%–100%). On gradient echo images blood flow within patent grafts appears bright ( Fig. 26.2 ). On spin-echo images, signal voids from metal clips, stents or calcifications, can falsely mimic graft patency. These artifacts are accentuated, thus easier to detect, on gradient echo compared with spin-echo images, which decreases the number of false positive patent grafts (increases specificity). On the other hand, the number of false-positive occlusions might be expected to increase (decreased sensitivity).
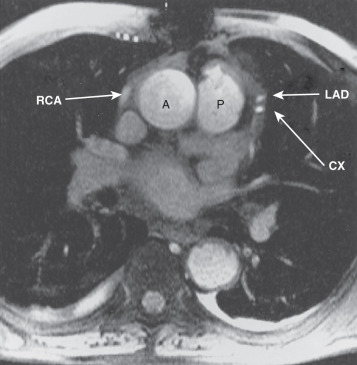
Two-Dimensional Breath-Hold Cardiovascular Magnetic Resonance Angiography
This technique, first described for imaging coronary arteries, can also be applied for visualization of bypass grafts. During a breath-hold lasting for 15 to 25 seconds, a segmented gradient echo image is acquired with 4 to 5 mm slice thickness and in-plane resolution of approximately 1 × 1.4 mm. The entire course of a bypass graft is covered by repeating the sequence multiple times. The procedure may become rather time consuming when multiple grafts have to be evaluated. Also, a high susceptibility to metallic materials used during surgery has been reported, with significant effects on diagnostic accuracy. This technique has been used for evaluation of sequential grafts ( Fig. 26.3 ). High sensitivity and specificity were found for predicting patency of grafts to branches of the RCA, but the accuracy decreased in distal graft segments to branches of the LAD and was poor in distal segments to the LCX.
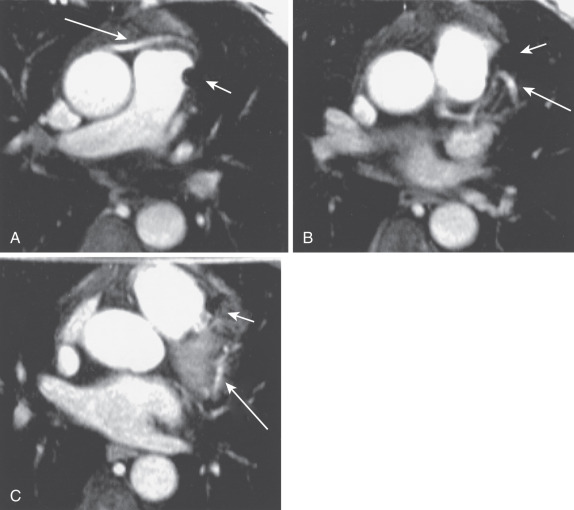
Another 2D breath-hold approach uses a multislice half-Fourier acquisition single shot turbo spin echo (HASTE) sequence. Seven T2-weighted images are generated within a breath-hold of approximately 15 seconds, with a 1.3 × 1.4 mm in-plane resolution and 5 mm slice thickness. Using this technique, Kalden and colleagues reported a sensitivity and specificity of 95% and 93%, respectively, in predicting graft patency and good visualization of 83% of distal graft segments. Because the HASTE sequence is less susceptible to metallic artifacts compared with gradient echo sequences, a remarkably high accuracy was found in detecting arterial graft patency (sensitivity of 90% and specificity of 100%). However, these figures must be interpreted with caution because the pretest probability of occluded arterial grafts is generally low. In that study, it is unclear how many of the 14 reported IMA grafts were occluded. In our personal experience, the HASTE sequence provides excellent visualization of coronary arteries and bypass grafts but rather poor detection of disease ( Fig. 26.4 ). Accordingly, Kalden and colleagues noted that only two out of eight hemodynamically significant graft stenoses were detected using HASTE sequence.
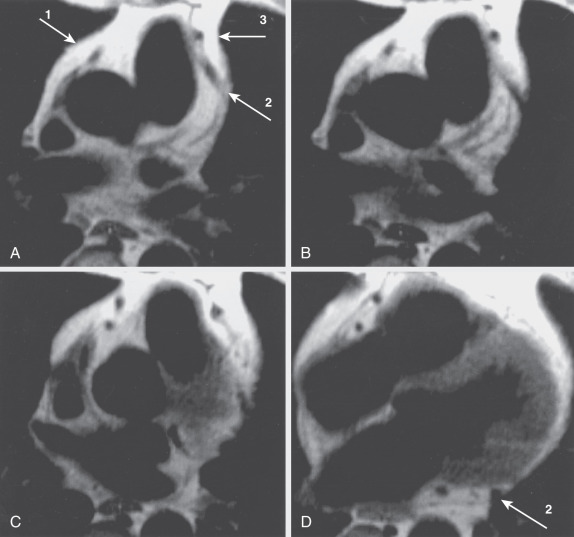
Three-Dimensional Respiratory-Gated Cardiovascular Magnetic Resonance Angiography
A three-dimensional (3D) dataset of truly contiguous slices may be obtained with respiratory-gated gradient echo techniques. Gating to the respiratory cycle is achieved by using navigators that monitor the diaphragm position. Magnetic resonance (MR) data acquired within a preset acceptance window of the respiration-induced diaphragm excursion are used for image reconstruction. The patient is allowed to breathe freely, at the expense of an increase in total imaging time. Several refinements of this gating procedure have been developed for imaging of the coronary arteries.
Kessler and colleagues used respiratory-gated 3D MR angiography (MRA) with navigator guiding and retrospective data processing for imaging of bypass grafts. In a relatively small number of 19 grafts, 13 out of 15 patent grafts and 4 out of 4 occluded grafts were correctly classified. Another study by Molinari and colleagues including 18 patients (51 grafts) which compared navigator guided 3D MRA with coronary angiography showed a sensitivity of 91%, the specificity of 97% for CMR in detecting occlusion versus patency of grafts.
A study by Langerak and colleagues including 38 post-CABG patients (56 venous grafts) who underwent conventional angiography for recurrent angina, assessed the accuracy of contemporary high-resolution respiratory-gated 3D MRA in detecting vein graft disease. The sequence used in the study included a T2-preparation prepulse (for muscle suppression), a fat suppression prepulse and had an acquisition window of 71 ms placed in mid diastole and at end expiration. A total of 50 out of 56 grafts were adequately visualized with a spatial resolution of 0.7 × 1 × 1.5 mm. The study reported sensitivities of 73% and 83%, with specificities of 80% to 87% and 98% to 100% in detecting grafts with ≥70% diameter stenosis and complete occlusion, respectively.
Three-Dimensional Contrast-Enhanced Breath-Hold Cardiovascular Magnetic Resonance Angiography
Breath-hold contrast-enhanced MRA is another technique which was first applied for aorta imaging. The T1-shortening effect of the gadolinium-contrast agent on blood signal allows the obtaining of high vascular contrast when using short TR/TE gradient echo sequences. After intravenous injection of an interstitial gadolinium-contrast agent, a 3D spoiled gradient echo sequence with short TR/TE (4.4/1.4 ms or even shorter) is applied. Within a breath-hold of approximately 30 seconds, a 3D volume slab composed of 24 to 32 contiguous slices with a total thickness of approximately 9 cm is imaged. Before acquiring the 3D volume slab, a single-slice 2D turbo gradient echo sequence is used to time the arrival of a contrast agent test bolus in the aorta. To maximize the contrast-enhancing effect, acquisition of the central k -space lines of the 3D imaging data is set to coincide with peak contrast arrival. This is achieved by introducing a time delay between contrast injection and start of the imaging sequence (delay = arrival time − or
of acquisition time). Depending on the field of view, matrix size, number of partitions and slab thickness a spatial in-plane resolution of 1 × 1.5 mm with 2- to 3-mm section thickness is achieved. Each partition of the 3D acquisition yields a “source” image. Studies are evaluated by reviewing the source images and after postprocessing techniques such as maximum intensity projection and planar or curved reformatting ( Figs. 26.5 and 26.6 ).
