The Need for Improved Breast Cancer Screening Methods Irrespective of tumor biology, it is true that there is a close correlation between the stage of breast cancer at the time of diagnosis and cancer survival. Breast cancer is a highly curable disease if diagnosed at a stage where the disease is localized, that is, confined to the breast, with 10-year survival rates well above 95%. The prognosis is still good, with 10-year survival around 80%, if the disease is regional, that is, confined to the axillary lymph nodes. Yet once the disease has spread, that is, once distant metastases are present, survival rates will be greatly reduced, and the patient will usually die of the disease. 1 The aim of screening is therefore to detect breast cancer in an early localized or regional stage. Mammographic screening has been found to be effective for this purpose, and helps reduce mortality from breast cancer. 2 Some important reasons to search for breast cancer screening methods beyond mammography are overdiagnosis of biologically inert disease, 3 as well as underdiagnosis of prognostically important cancers that are observed with mammographic screening. Overdiagnosis may be particularly problematic for structural (radiographic) breast imaging techniques, that is, mammography or tomosynthesis. Radiographic breast imaging detects cancer by depicting regressive changes such as tumor necrosis, apoptosis, or hypoxia—hallmarks of cancer that lead to the calcifications, fibrosis, and architectural distortions then picked up by a mammogram. It is well established that a screen-detected, that is, mammographically visible, breast cancer is associated with a better prognosis than a cancer of the same size that was not screen detected, that is, mammographically occult, but detected as so-called interval cancer in between mammographic screening rounds. This effect is referred to “length time bias.” It implies that the cancer yield of mammographic screening may overestimate its power to reduce mortality because it detects prognostically less aggressive types of breast cancer. Overdiagnosis—that is, the diagnosis of nonprogressive, self-limiting breast cancer—is a length time bias put to an extreme. The flipside to this is that mammographic (or radiographic) imaging performs relatively poor in detecting rapidly growing, prognostically important breast cancers. Such cancers are usually not necessarily hypoxic, but well fed with nutrients and oxygen through active angiogenic activity; accordingly, they may not develop calcifications and do not induce fibrosis. Moreover, these cancers are usually hypercellular and exhibit pushing margins, which makes them mammographically occult if embedded in breast tissue, and even if they are mammographically detectable, they will be indistinguishable from cysts or fibroadenomas. In summary, there is a propensity of radiographic imaging to detect slowly growing cancers, and a lack of sensitivity for prognostically important, rapidly growing, cancers. The limited sensitivity of screening mammography has also been observed in DMIST (Digital Mammography Screening Trials). This trial was designed to compare the clinical performance of film-screen with that of digital mammography and recruited over 50,000 women. The DMIST found an overall sensitivity of screening mammography to range between 36% and 38% for women with dense breast tissue (ACR 3 and 4), suggesting that over half of cancers will not be picked up by screening mammography. 4 Another piece of evidence on “underdiagnosis” is available through the performance reports on organized mammographic screening programs. European quality assurance guidelines accept an interval cancer rate (referenced to the background incidence of breast cancer) of up to 50% for 2-year screening periods. This implies that half of cancers that occur in a screening cohort will not be picked up by mammography. Last, and most important, breast cancer continues to represent the most or second most important cause of cancer death, and is the main reason for “life years lost” in the female population. Despite several decades of publicly organized screening programs, breast cancer mortality remains high. This, together with the well-established correlation between early diagnosis and ultimate prognosis of women with breast cancer, suggests that there is room, and need, for further improvement of screening methods even for women at average risk. Current candidate methods for complementary breast cancer screening are digital breast tomosynthesis (DBT), contrast-enhanced spectral mammography (CESM), ultrasound, and magnetic resonance imaging (MRI). Several large clinical studies have been published on the use of DBT for supplementary breast cancer screening, that is, the use of DBT in addition to screening mammography. The added cancer yield achievable by DBT ranges between 1.1 and 6.7 per 1,000 women, with an average of 1.25 per 1,000. The main impact of using DBT in addition to mammography is to avoid recall and improve the Positive Predictive Value (PPV) of biopsy recommendations from 23% to 29%. Accordingly, DBT will modestly improve cancer detection rates / sensitivity, as well as specificity / PPVs. 11, 12, 13 However, information on the biologic profile of the breast cancers that were found by DBT in addition to mammographic screening is scarce. Technically, DBT mainly improves the detection of architectural distortions and stellate lesions—cancers that, based on their imaging phenotype, may more likely represent well-differentiated, low-grade, disease. As such, although DBT may avoid some “underdiagnosis” of breast cancer, that is, will improve the detection of breast cancer missed by regular full-field digital mammography, it is relatively unlikely that it will help reduce overdiagnosis. Indeed, for reasons mentioned earlier, it is possible that it will even contribute to additional overdiagnosis of biologically unimportant disease ( ▶ Table 3.1). CESM has so far been used for diagnostic purposes only, not for screening. However, the emerging results suggest that contrast injection may greatly increase the sensitivity of digital mammography, to levels that may be similar to those obtained with contrast-enhanced breast MRI. Although sensitivity levels are still a matter under investigation, it is likely that CESM could serve as surrogate for diagnostic breast MRI. If one considers using CESM for screening, it is important to keep in mind that the safety profile of any type of iodinated contrast agent used for CESM is far less advantageous compared to that observed for any type of MRI contrast agent used for breast MRI. With modern, macrocyclic contrast agents recommended for breast MRI, the volume of injection ranges between 5 and 9 mL, whereas the injection volume for iodinated contrast agents for CESM ranges between 70 and 150 mL. Although nephrogenic systemic fibrosis (NFS) screening may be recommendable in some women prior to injection of a macrocyclic MRI contrast agent, it will always be important to test for renal as well as thyroid function in women undergoing injection of iodinated contrast agents. Accordingly, patient preparation will be more complex for CESM, especially if this method was used in a screening situation. Ultrasound has been proposed to help improve the detection of breast cancer in women with dense radiographic tissue ( ▶ Table 3.2). Several prospective screening studies showed that screening ultrasound is indeed useful to increase the cancer detection rate by an average of 4.1 per 1,000 compared with mammographic screening in the same individuals. The increased cancer detection rate associated with supplemental ultrasound screening does reduce the interval cancer rate. In their American College of Radiology Imaging Network (ACRIN)–sponsored 6666 trial, 21 Berg and colleagues reported an interval cancer rate as low as 8%. A limitation of screening ultrasound is the relatively broad overlap of ultrasound imaging features of benign and malignant lesions. Published rates of PPV for screening ultrasound range between 8 and just over 20%. 22 This may lead to substantial additional downstream costs and possibly morbidity. Another limitation is the radiologist’s time it takes to complete a screening ultrasound study. In the 6666 trial by Berg and colleagues, 21 the average time for a bilateral ultrasound screening study was around 19 minutes. Automated breast ultrasound may help reduce the physician time substantially; however, at the time this article was written, the supplemental cancer yield and PPV rates of screening by automated breast ultrasound was unknown. 23 After completion of their ACRIN 6666 ultrasound screening study, Berg and coworkers were able to offer their screening cohort—women who had undergone several years of annual mammography plus ultrasound—a single round of screening MRI. This yielded an additional supplemental cancer detection rate of 14.6 per 1,000, which compares favorably to the rate of 4.1 per 1,000 in the same cohort based on mammography plus ultrasound screening. 24 This evidence is in good agreement with a series of studies that had been published on the use of MRI, mammography, and ultrasound for screening women at increased risk of breast cancer, and which consistently found about doubled sensitivity of MRI compared with the combined use of mammography and ultrasound. 25, 26, 27, 28, 29, 30, 31, 32, 33, 34, 35, 36 Beyond the comparison of mere numbers of “cancers detected” by different breast screening methods, it is more and more evident that it is at least as important to investigate what type of cancers are diagnosed by different screening methods. The major difference between conventional breast imaging or ultrasound and breast MRI is the fact that in the latter case, cancer is detected due to its local contrast enhancement. Breast MRI is not only a diagnostic tool but also an in vivo imaging biomarker for disease activity or tumor biology. Enhancement of a ductal carcinoma in situ (DCIS) or of an invasive cancer requires a locally increased vessel density, an increased vessel permeability and—in the case of DCIS—an increased permeability of the ductal basal membrane. Breast cancer detection in MRI is therefore based on pathophysiological changes that are indicative of cancer proliferation, infiltrative growth, and metastasis. 37, 38 In good agreement with these pathophysiological considerations, it has been shown that screening MRI is associated with something one could call a “reverse length time bias,” that is, cancer detection in MRI is biased toward prognostically important disease. Cancers detected only by MRI tend to exhibit high nuclear grade and high Ki-67 values, hallmarks of rapid growth. In turn, malignant lesions that went undetected by MRI screening, and picked up by mammography alone, typically consist of low-grade DCIS. Trials that compared the added value of mammography in women undergoing MRI for screening concordantly found that the additional cancers diagnosed through mammographic screening mainly represent disease with limited, if any, prognostic importance. Author Year Journal No. of participants Additional cancer yield Rose et al 5 2013 AJR 9.499 1.1/1,000 (not significant) Ciatto et al 6 2013 Lancet Oncology 7.292 2.7/1,000 Skaane et al 7 2013 Radiology 12.621 2.3/1,000 Friedewald et al 8 2014 JAMA 454.850 1.2/1,000 Sharpe et al 9 2015 Radiology 5.703 1.9/1,000 McDonald et al 10 2015 AJR 15.571 1.3/1,000 Average additional cancer yield 1.25/1,000 Type Author Year Women Added cancers Additional cancer yield Fraction of DCIS PPV HH-US Gordon et al 12 1995 12.706 44 3.5 per 1,000 0 (0%) 16.0% HH-US Buchberger et al 13 2000 8.103 32 3.9 per 1,000 5 (13%) 9.0% HH-US Kaplan et al 14 2001 1.862 6 3.0 per 1,000 1 (16%) 10.0% HH-US Kolb et al 15 2002 13.547 37 2.7 per 1,000 1 (3%) 10.0% HH-US Crystal et al 16 2003 1.517 7 4.6 per 1,000 0 (0%) 18.0% HH-US Berg et al 17 2008 2.809 41 4.5 per 1000 1 (2%) 8.0% HH-US Ohuchi et al 18 2015 36.859 61 1.7 per 1,000 9 (15%) 9.7% ABVS Brem et al 19 2015 15.318 30 1.9 per 1,000 2 (7%) 9.8% Abbreviations: ABVS, automated breast volume scanner; DCIS, ductal carcinoma in situ; HH-US, handheld breast ultrasound; PPV, positive predictive value. The American Cancer Society guidelines recommend annual breast MRI for screening of women at an increased risk of breast cancer, defined as a 20% lifetime risk. 39 Many women will carry such a lifetime risk, yet only a minority of women who would need MRI for screening do indeed have access to MRI screening. 40 This is not because the respective MR systems would not be physically available. 41 Although the individual reasons for failure to undergo MRI screening for women at high risk of breast cancer are not well established, the perceived high cost of the examination, together with a lack of knowledge about the implications of a high lifetime risk, will play a major role. Trials that compared the diagnostic accuracy of MRI with that of screening mammography with or without ultrasound ( ▶ Table 3.3) yielded concordant results in that MRI was consistently more accurate than mammography and ultrasound. The actual sensitivity levels achieved by MRI varied between 71% and 100% in the different studies. The major reason for sensitivity levels below 80% observed in some of the early studies on MRI screening was failure to diagnose DCIS on MRI. Later publications by the same groups confirmed this. Accordingly, the sensitivity of MRI will range between 90 and 100%. Cancers missed by MRI are, in the vast majority of cases, due to low-risk (low-grade) DCIS. 42 The EVAluation of imaging methods for early diagnosis of familial breast cancer, or EVA trial, investigated the respective contributions of mammography, ultrasound, and MRI to the individual diagnosis of breast cancer in intermediate- to high-risk women undergoing screening and found that MRI is by far the most important single imaging method. In the EVA cohort, mammography alone exhibited an average sensitivity of just above 33%, which is in good concordance with the mammographic sensitivity established in the DMIST trial for women with intermediately dense and dense breast tissue. If ultrasound was added to mammography, the sensitivity rose by about 50% to an overall sensitivity of 48%. MRI alone offered a sensitivity of 93%. If mammography was added to MRI, there was a nonsignificant increase in sensitivity to 100%. The additional lesions depicted by mammography, that is, lesions that had been undetected by MRI, were three cases of low-grade DCIS. Accordingly, the results of the EVA trial suggested that (1) MRI could be used as a stand-alone imaging method and (2) the cancers missed by MRI represent cancers which could well be considered “insignificant.” The EVA trial thus provided initial evidence for the fact that MRI could avoid not only underdiagnosis of biologically important invasive disease, but also overdiagnosis due to diagnosis of low-grade DCIS. The appropriate metric to rate the success of breast cancer screening programs is the interval cancer rate observed in the screened cohort. In the different MRI screening cohorts, the reported interval cancer rates ranged between 0% and 6%. Accordingly, this rate is much lower than that observed in regular mammographic screening programs, where interval cancer rates as high as 50% have been reported. The low interval cancer rate observed in MRI screening trials was specifically remarkable in view of the high risk of the women included in the MRI screening trials; women with familial breast cancer tend to develop rapidly growing cancers. The higher the rate of cancers with fast growth, the more difficult it is to achieve low interval cancer rates. It should be well understood that, due to the frequently “atypical” imaging appearance of BRCA-associated breast cancers, and the high prevalence of benign enhancing lesions observed in young women at high risk, MRI screening of these women belongs to the most demanding applications of breast MRI in general. Profound personal experience with breast MRI in general, and with diagnosis of familial breast cancer in particular, is of utmost importance. It is important to realize that, as with all imaging methods, a learning curve exists for breast MRI as well that needs to be taken into consideration when reviewing published results on MRI screening. This is also true for the PPV; recent trials suggest that the PPV of MRI, if used for screening, is at least as high as that of mammographic screening in the same individuals. 43, 44 The rationale of “abbreviated breast MRI” (AB-MRI) is to reduce the image acquisition time, thus the magnet time, of breast MRI, as well as the image reviewing time, thus the radiologist time, needed for breast MRI interpretation ( ▶ Fig. 3.1). The aim is to reduce the cost associated with a breast MRI screening study, as well as to improve patients’ (or, rather, women’s) acceptance of breast MRI. The overall goal is to improve access to breast MRI for screening not only for women at high and intermediate risk, but also for those at average risk who wish to undergo screening with a test that grants the highest cancer detection rate that is currently achievable. The concept of AB-MRI was introduced by our group 45 and consists of the following four components: Acquire an abbreviated breast MRI protocol that lasts no longer than 5 minutes magnet time. Use maximum intensity projection (MIP) reconstructions of the first postcontrast subtracted (FAST) images for quick review and to decide upon the presence or absence of significant enhancement. Use the individual FAST images and, if necessary, their nonsubtracted pre- and postcontrast source images, for characterization of enhancing lesions. Have expert radiologists with specific expertise in breast MRI as well as in reading AB-MRI interpret the images. Complying with each of these components is essential to reproduce the results published by our group. Personal experiences with reading abbreviated protocols are easy to establish. A possibility is to prospectively read a larger number of MRI screening studies and review the first postcontrast series only. Details on acquisition protocols and interpretation guidelines will be discussed in Chapter 4 of this book. The usual, multiparametric, “full protocol” breast MRI provides information on high-resolution, cross-sectional lesion morphology, as well as functional information on a variety of tissue features. This includes information on tissue microvessel and macrovessel perfusion, vessel permeability, tissue relaxation times, tissue cellularity/proliferation rate, and interstitial pressure. All this information can be useful for tissue characterization, that is, for the distinction of benign versus malignant lesions, as well as for a further classification of the biologic and prognostic importance of breast cancers and their precursors (DCIS). Due to its complexity, its diagnostic power, and its cost, MRI has traditionally been used as a third-line imaging method, that is, done in patients in whom disease was already known to be present on clinical grounds or based on other imaging methods, and where an improved differential diagnosis or diagnosis of disease stage was desired. Accordingly, when breast MRI was developed in the 1990s, it had mainly been used to settle equivocal conventional imaging results and/or on patients with known breast cancer in whom an accurate delineation of extent or an accurate classification of disease was desired, or for response assessment. In such situations, it is appropriate to employ extensive pulse sequence protocols in order to achieve a maximum diagnostic accuracy for disease characterization (differentiation of benign vs. malignant disease and also assessment of cancer viability). Accordingly, current breast MRI protocols are time consuming to acquire and to read. A typical MRI study occupies the MR system for up to 40 minutes and generates several hundred images. Mammographic screening, on the other hand, is a highly standardized and relatively simple procedure. Women undergo mammography, usually without clinical examination, and the resulting mammograms are interpreted by highly trained and specialized screening radiologists who batch read up to 100 mammograms per hour. Accordingly, we proposed to use an approach to breast MRI screening that pursues a concept similar to that of mammographic screening programs. We proposed an abbreviated, short MRI protocol that is limited to the early postcontrast period, then to use standard image reconstruction tools (subtraction and MIP) to allow a very fast reading of these abbreviated protocols, and to compensate for the reduction of imaging information by having expert radiologists interpret this limited protocol. The aim was to substantially reduce image acquisition and reading time of screening MRI. Long-term goal is to increase the access to screening breast MRI. The rationale to focus on the early postcontrast period is as follows. The principal concept of dynamic contrast-enhanced breast MRI is to detect cancer based on their enhancement that will be faster and stronger compared with that of the adjacent fibroglandular tissue. Invasive breast cancers usually reach their maximal enhancement already in the early postcontrast period; this is then followed by either a plateau of enhancement or even a signal loss (washout). As opposed to this, benign changes as well as the background enhancement of the normal fibroglandular tissue will exhibit persistent enhancement, that is, it will become progressively brighter over time. Accordingly, the optimal contrast between a cancer and its environment is achieved in the very early postcontrast phase, where contrast enhancement in the cancer is maximal, and where signal of benign changes is still minimal. Accordingly, the early postcontrast phase is best suitable to detect significant enhancement, and also to characterize enhancing lesions based on their morphology. Anatomic details such as fine spiculations are best resolved when the contrast between the cancer and its surrounding tissue is optimal. The abbreviated protocol published by us consists of only one post-contrast phase; however, it does provide information on enhancement kinetics. Because images are acquired in the early post-contrast phase, AB-MRI captures the wash-in kinetics. With abbreviated protocols, we may lose the information on wash-out kinetics that is assessed through review of the additional dynamic frames. Yet while wash-out kinetics were essential in the early days of breast MRI, their importance was decreasing with the increasing spatial resolution that can be established with contemporary MR pulse sequences, and which improves the analysis of morphological details of enhancing lesions. Our regular breast MRI protocol, as well as the abbreviated protocol, consists of a dynamic series acquired without fat suppression, as detailed in Chapter 4. ▶ Fig. 3.2 demonstrates how we use non–fat-suppressed images to improve the depiction of anatomic details of enhancing lesions detected on FAST images. Fig. 3.1 Concept of abbreviated breast magnetic resonance imaging (MRI). (a) Schematic presentation of the abbreviated and the full diagnostic protocol. B-E MR images of a patient with left-sided breast cancer. (b) Midbreast section of the baseline (precontrast) dynamic acquisition (Dyn 0 in a). (c) Corresponding section of the first postcontrast dynamic acquisition (Dyn 1 in a). (d) Corresponding FAST (first postcontrast subtracted) image, generated by subtracting image (b) from image (c). E, maximum intensity projection (MIP), generated by fusing all FAST sections into a single 3D-like projection image.
3.2 Candidate Novel Breast Cancer Screening Methods
3.3 Evidence for the Use of Breast MRI for Screening
3.4 The Rationale and Concept of “Abbreviated Breast MRI”
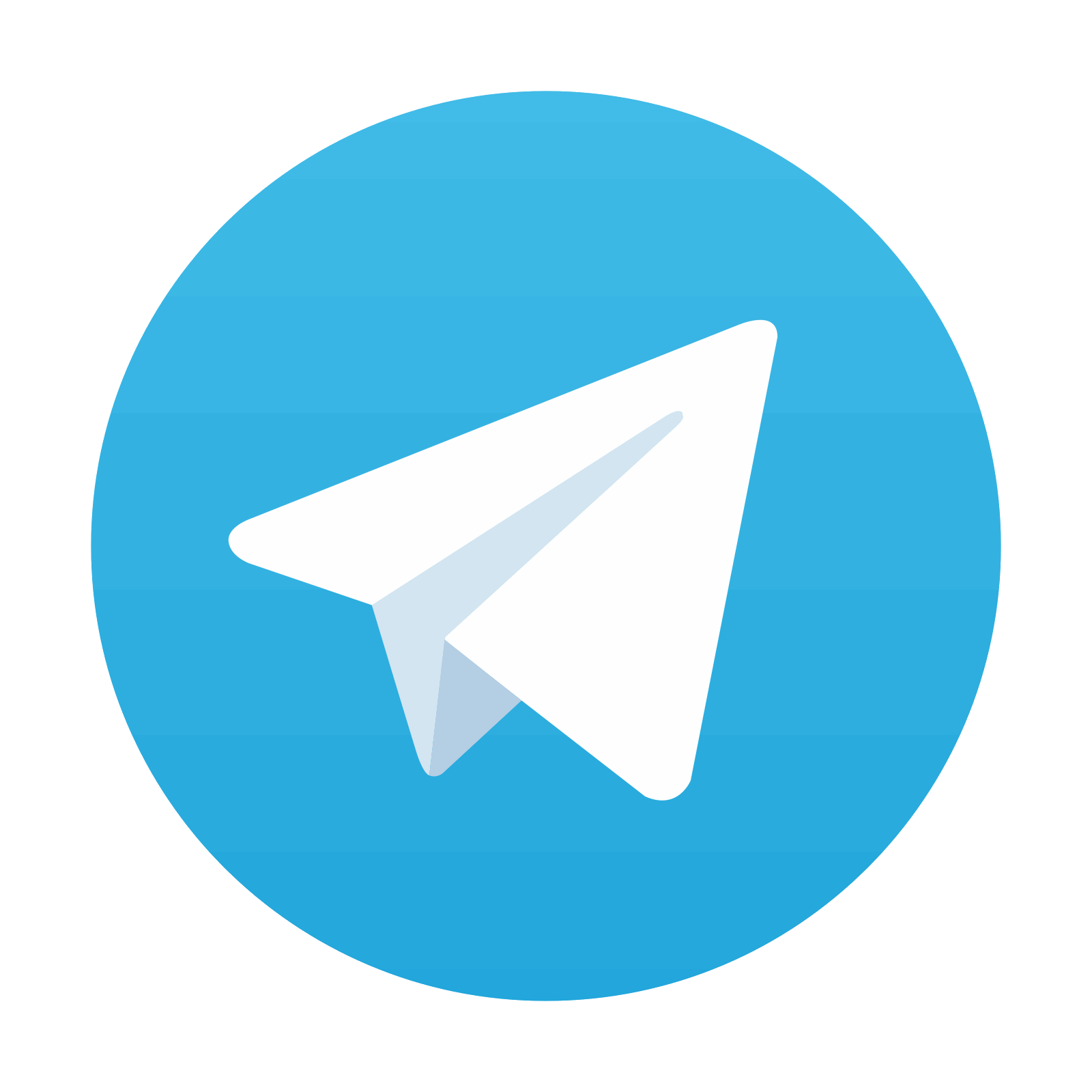
Stay updated, free articles. Join our Telegram channel

Full access? Get Clinical Tree
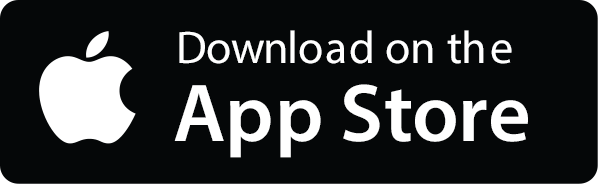
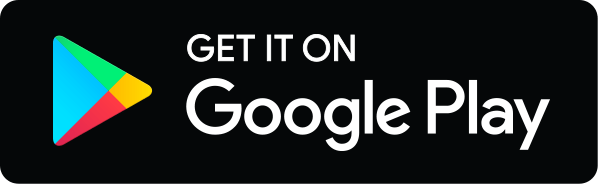