The functional magnetic resonance (fMR) technique for brain mapping is a valuable tool for understanding both normal physiology and the dysfunction taking place in disorders of the brain. This article provides an overview of fMR imaging methods and their applications in the study of neurologic movement disorders. The article also reviews the current neuroimaging literature regarding parkinsonisms, dystonia, essential tremor, and Huntington disease, and includes a discussion of current methodological limitations and future directions for this exciting field.
The emergence of functional magnetic resonance imaging (fMRI) techniques for brain mapping has led to an explosion of data on normal brain physiology and the dysfunction that takes place with disease. This article provides a brief overview of fMRI methods and their applications in the study of neurologic movement disorders. There are today no clinical indications for the use of fMRI–based techniques in the area of neurologic movement disorders. We have therefore summarized seminal contributions in the various areas of movement disorder research, while providing a sketch of what this technology allows now and where this technology may lead.
History of functional neuroimaging
Human interest in visualizing the active brain is nothing new. The notion that regional cerebral blood flow could serve as a surrogate marker of underlying neural activity was first demonstrated by the work of Roy and Sherrington in 1890. Early efforts to map neural activity used short-lived radionuclides incorporated into compounds, such as glucose and water. These radionuclides could be quantitatively and qualitatively measured with CT, leading to the development of positron emission tomography (PET). The ongoing development of PET tracers has allowed for the virtually limitless ability to study any metabolic pathway, as long as a relevant radiotracer could be synthesized to target the area of interest. The primary limitations associated with PET remain the radiation exposure, albeit limited; the extensive infrastructure required for synthesis of the radiopharmaceuticals; and lack of temporal resolution during data collection.
The development of MRI techniques for functional brain mapping is a much more recent development. A wide variety of techniques fall under the category of fMRI, with most techniques using the different magnetic properties of hydrogen atoms (protons) comprising water molecules ubiquitous throughout the body. Despite the lack of targeting probes as seen with PET, MR-based techniques compare favorably because of the availability of scanners at various points of care, lower cost of implementation, noninvasiveness, and higher temporal resolution compared with PET. However, no single imaging modality is likely to provide a comprehensive understanding; the use of complementary modalities in serial or parallel is now becoming a standard part of research protocols.
Overview of fMRI techniques
The technique most commonly used in fMRI studies is based on the principle of blood oxygen level–dependent (BOLD) contrast changes. BOLD-fMRI uses the different magnetic properties of oxygenated and deoxygenated hemoglobin to identify regional blood flow changes in the brain. If we assume a baseline level of neural activity and blood flow, then activation of this neural region will lead to increased oxygen consumption. This increased oxygen demand leads to a brief reduction (100–200 milliseconds) in the concentration of oxyhemoglobin relative to deoxyhemoglobin at the vascular bed. This small reduction in oxyhemoglobin is followed by a disproportionately larger compensatory increase in blood flow leading to an increase in oxyhemoglobin and drop in deoxyhemoglobin that surpasses preactivation levels. The signal from these blood flow changes is derived from the drop in deoxyhemoglobin, and leads to an increase in BOLD signal within a few seconds, which peaks around 4 to 8 seconds before it returns to baseline 14 to 16 seconds after the stimulus onset ( Fig. 1 ).
Echoplanar imaging sequences are frequently used for these functional studies since they can rapidly collect multiple two-dimensional slice snapshots after the application of a single gradient radiofrequency pulse. With each slice being acquired in about 50 milliseconds, echoplanar imaging sequences can collect whole-brain volumes in a standard 2-second repetition time. Multiple volumes are collected during the course of a study.
Overview of fMRI techniques
The technique most commonly used in fMRI studies is based on the principle of blood oxygen level–dependent (BOLD) contrast changes. BOLD-fMRI uses the different magnetic properties of oxygenated and deoxygenated hemoglobin to identify regional blood flow changes in the brain. If we assume a baseline level of neural activity and blood flow, then activation of this neural region will lead to increased oxygen consumption. This increased oxygen demand leads to a brief reduction (100–200 milliseconds) in the concentration of oxyhemoglobin relative to deoxyhemoglobin at the vascular bed. This small reduction in oxyhemoglobin is followed by a disproportionately larger compensatory increase in blood flow leading to an increase in oxyhemoglobin and drop in deoxyhemoglobin that surpasses preactivation levels. The signal from these blood flow changes is derived from the drop in deoxyhemoglobin, and leads to an increase in BOLD signal within a few seconds, which peaks around 4 to 8 seconds before it returns to baseline 14 to 16 seconds after the stimulus onset ( Fig. 1 ).
Echoplanar imaging sequences are frequently used for these functional studies since they can rapidly collect multiple two-dimensional slice snapshots after the application of a single gradient radiofrequency pulse. With each slice being acquired in about 50 milliseconds, echoplanar imaging sequences can collect whole-brain volumes in a standard 2-second repetition time. Multiple volumes are collected during the course of a study.
fMRI experimental designs
Based on the area of interest and the hypothesis, three main types of study designs are used in fMRI experiments: block, slow event-related, and fast event–related. Block design paradigms are the most frequently used because of their simplicity, ease of implementation, and sensitivity. This design consists of continuous presentation of a task for at least 20 seconds followed by an alternate condition, frequently a rest period of similar duration. The data collected from a block design experiment allow each task condition to be averaged to improve the signal-to-noise ratio (SNR) and then a simple “contrast” can be performed to compare the two conditions. The contrast can be as simple as a subtraction of the BOLD responses of one condition from another or, more commonly, parametric statistics are performed at each voxel ( Fig. 2 ).
The prolonged duration required for each condition in a block design experiment, however, has many inherent design limits. For example, the long duration required for each condition limits the ability to study multiple conditions within the time constraints of a standard scanning session. The ability to extract temporal information about the brain responses is also limited because of the prolonged BOLD response. These restrictions among others have led to the use of slow and fast event–related designs. In the case of slow event–related paradigms, the standard experimental blocks are shortened while maintaining the alternating patterns of stimulation. Fast event–related experiments, on the other hand, consist of rapid stimulus periods (as short as 34 milliseconds) occurring repeatedly at varying intervals. All of these experimental designs assume a linearity with the BOLD signal, with fast event–related designs being most dependent on this principle. Despite the apparent advantages associated with the faster designs, each sacrifices some SNR (35% drop in SNR for slow event–related vs block ; 17%–25% drop in SNR for fast event–related vs slow event–related ), though this is arguably outweighed by the increased number of trials possible.
fMRI data analysis
A detailed summary of the various steps required to process fMRI data is beyond the scope of this review. For those interested in learning more about the various steps in preprocessing and data analysis at the single subject and group level, we recommend “Functional Imaging Analysis Contest (FIAC) Analysis According to AFNI and SUMA,” by Z.S. Saad and colleagues (Hum Brain Mapp 2006;27:417–24).
Clinical indications of fMRI to diagnose neurologic movement disorders
The rapid development of fMRI for both clinical and nonclinical applications provides a unique opportunity to use this exciting modality for answering questions related to pathophysiology and diagnostic uncertainty, for identifying mechanisms of therapeutic action, and for monitoring effectiveness of therapy, among many other tasks. Despite the wide array of opportunities for the use of fMRI as a clinical test, no indications are currently available for its use in neurologic movement disorders. The following sections provide an overview of the large volume of work that has taken place in this area, and points to potential directions for future clinical applications.
Summary of fMRI findings in select movement disorders
Parkinson Disease
Clinical overview of Parkinson disease
Parkinson disease was first described in 1817 by James Parkinson in “An Essay on the Shaking Palsy.” Parkinson disease is the second most common neurodegenerative disorder, after Alzheimer disease, with a prevalence of 0.6%. The clinical hallmarks of Parkinson disease include:
Resting tremor
Muscle rigidity
Bradykinesia
Postural instability
At least two of these aforementioned features are required to make a formal diagnosis, though the symptoms of Parkinson disease include a diverse range of other motor and nonmotor impairments. Many of these impairments are attributed to a loss of dopaminergic cells in the substantia nigra, which project from the midbrain to such areas as the putamen and caudate in the striatum. Since the predominant abnormality in Parkinson disease involves a loss of dopamine production, therapy is targeted at restoring dopamine levels. Dopamine levels can be increased using a wide variety of approaches, including the use of dopamine precursors (levodopa) converted to dopamine by the brain, the use of dopamine agonists, or by slowing the breakdown or reuptake of dopamine at nerve terminals.
The constellation of clinical features combined with evidence of a functional improvement on dopaminergic therapy helps to confirm the diagnosis of primary or idiopathic Parkinson disease. Idiopathic Parkinson disease is the most common parkinsonism, accounting for 42% of cases. However, many varieties of parkinsonisms present with distinctive combinations of clinical findings and a lack of response to dopaminergic therapy. These dopamine-nonresponsive disorders are referred to as Parkinson-plus syndromes and include multiple system atrophy, progressive supranuclear palsy, and corticobasal degeneration. As a group, Parkinson-plus disorders progress more rapidly than primary or secondary parkinsonisms and have more widespread and severe neuro-degeneration. A review of each of these disorders and their similarities or differences is beyond the scope of this review, though the current best in vivo method for differentiating between primary, secondary, and Parkinson-plus disorders is a clinical examination by an experienced movement disorder neurologist. Furthermore, the distinctive characteristics of these various disorders frequently become evident only after a diagnosis of parkinsonism is made and treatments are found to be ineffective. Such limitations in early diagnosis constrain the ability of the clinician to develop a stratified plan of management based on the particular disease subtype.
fMRI studies of Parkinson disease
Early fMRI studies of Parkinson disease aimed to identify differences between patients and controls in the motor network. Such differences, it was hoped, could be used as signatures of the disorder. Early work by Tada noted decreased activation in the supplementary motor area during movement in Parkinson disease versus controls. This finding was confirmed by Sabatini and colleagues, who also demonstrated a common pattern of motor network activation (premotor, motor, parietal cortex, striatum, and cerebellum) in both groups, though cortical motor areas showed higher levels of activation in patients than in controls. Similar findings were reported using PET and single photon emission CT (SPECT), while others found reduced activation in motor-related cortical areas. These seemingly discrepant findings are disconcertingly common in the neuroimaging literature. It should be noted, however, that these findings emphasize the context-specific performance of the neural networks being studied. In the majority of these neuroimaging studies, efforts were made to ensure that state-related confounds (eg, differing performance, effort, anxiety) were minimized among the groups being compared. Despite such efforts, it is inevitable that such confounds exist even in patients with mild disease when compared with healthy controls.
The cognitive manifestations of Parkinson disease have also been studied with fMRI. The findings of these early studies have identified dysfunction in numerous cognitive domains, including working memory, set-shifting or the ability to alter behavior to dynamically changing circumstances, reward processing, and semantic sequencing. Such cognitive impairments also have the potential to affect motor performance. This was evident when subjects with Parkinson disease performed both simple and complex dual tasks during fMRI. The findings suggested that poorer performance of dual tasks related to impairments in attention, impairments in performance monitoring, and a limited capacity of such resources. Despite the behavioral basis of these impairments, administration of a dopaminergic agent led to both an enhancement of prefrontal cortex performance, relative to a baseline hypodopaminergic state, and normalization of fMRI activation patterns.
The development of a noninvasive measure to distinguish the various parkinsonian subtypes might also serve as a tool to monitor disease progression and the impact of therapy. Work by a number of groups using fMRI as a potential biomarker to differentiate disease subtypes and to monitor progression is ongoing. No clinical indications are yet approved for the use of fMRI in patients suspected of having parkinsonism.
Dystonia
Dystonia is a neurologic movement disorder characterized by sustained muscle contractions that lead to disabling repetitive movements or abnormal postures. Dystonia can begin at any age from childhood through late adulthood. Early-onset cases (before the age of 25), referred to as primary dystonia , typically present with a generalized pattern of muscle involvement and can lead to disabling muscular and orthopedic deformities. Patients with a later onset of dystonia typically present with more focal or segmental regions of involvement, with the most common presentation being cervical dystonia. The prevalence of primary generalized dystonia is estimated to be 3.4 per 100,000, while the frequency of focal dystonias is 29.5 per 100,000.
The etiologic mechanisms of dystonia are not known and no test is currently available for diagnostic confirmation, with exception of a small number of inherited subtypes (eg, DYT-1 mutation) confirmed by genetic testing. One possible cause of dystonic symptoms involves neurotransmitter changes that lead to an imbalance of dopamine transmission within the basal ganglia. Direct evidence for this comes from dopa-responsive dystonia. Indirect evidence for this hypothesis comes from the development of dystonic symptoms in patients treated with the dopamine precursor levodopa (used to treat Parkinson disease) or following the use of dopamine antagonists frequently used to treat psychosis. Additionally, observations of patients with dystonia note difficulty initiating and performing voluntary movements using only the appropriate muscles required to carry out the action. In such cases, antagonist muscles are simultaneously activated, leading to muscular “co-contraction” and the appearance of dystonic sustained muscular contractions. This observation has led to the hypothesis that normal brain mechanisms leading to the inhibition of surrounding antagonist muscles are impaired in dystonia.
Routine structural imaging of the brain in patients suspected of having dystonia show no consistent abnormalities. Studies using diffusion tensor imaging to characterize the architectural integrity of white matter tracts have identified changes in subgyral sensorimotor cortex and the lentiform nucleus, with the latter area appearing to normalize 4 weeks after treatment with botulinum toxin. Voxel-based morphometry methods have similarly been useful in identifying subtle changes in the gray matter volumes of subjects with dystonia.
Functional imaging methods, such as PET and fMRI, have provided additional insights into the neural mechanisms underlying dystonia. Abnormalities in premotor, supplementary motor, and primary motor cortices have been identified during movement in both generalized and focal dystonias. These results are confusing because one study showed decreased activity of patients relative to controls, and other studies showed increased activity. Future studies would likely benefit from the inclusion of behavioral measures, such as electromyography during fMRI, to allow for better differentiation of task from rest, while matching performance with healthy controls.
Essential Tremor
Essential tremor, a common movement disorder, is seen as early as childhood with increasing incidence with age. It affects up to 14% of people over age 65. Essential tremor is most frequently exemplified by rhythmic shaking of the arms, but may also involve the head, tongue, legs, voice, and face. Essential tremor is recognized as a heritable disorder with apparent Mendelian autosomal dominant transmission, though no disease-causing genes have yet been clearly identified. Treatments are based primarily on pharmacologic agents, although surgical intervention may be an option in the most disabling cases.
The pathophysiology of essential tremor has been only partially defined. A number of direct and indirect neurophysiological studies have implicated a neuronal network involving the thalamus, the sensorimotor cortex, the inferior olivary nuclei, and cerebellum in the production of essential tremor. This is also supported by an animal model using harmaline, which induces a reversible essential tremor–like state with abnormal tremor-specific oscillations observed in the olivocerebellar pathway.
Studies of essential tremor using fMRI have helped to confirm the neural network involved. Such studies have typically contrasted the brain activations observed during postural or action tremors in essential tremor patients with the brain responses of patients during rest when the tremor resolves, or with healthy controls mimicking tremor. Findings from a number of studies have consistently shown involvement of a widespread motor network associated with tremor, including primary motor cortex, primary sensory cortex, thalamus, globus pallidus, red nucleus, cerebellar hemispheres, and dentate nucleus of the cerebellum. Mimicked tremor by healthy controls led to a similar network becoming active, though at slightly lower levels than in patients. These findings suggest that tremor is generated through the same neural network that generates voluntary movement. Studies to date, however, have been unable to elucidate the location of the tremor oscillations. Determining whether there are changes in functional connectivity may also help to explain the occurrence of the tremor during posture or action, but not at rest.
Studies in essential tremor give some indication about the difficulties in this sort of work. Studies at rest in the patients seem theoretically fair to compare in the treated and untreated condition, and even to compare with normals. However, tremor is not present in this situation. Studies with voluntary movement and tremor are difficult to compare with normal voluntary movement, even if tremor is mimicked.
Huntington Disease
Huntington disease is a hereditary progressive neurodegenerative disorder with neurologic and psychiatric features. The classic signs of Huntington disease include the development of chorea (diffuse, involuntary, rapid, irregular, jerky movements) and a gradual loss of thought processing and acquired intellectual abilities (dementia). Although symptoms typically become evident during the fourth or fifth decades of life, the age at onset is variable and ranges from early childhood to late adulthood (eg, 70s or 80s). Huntington disease is transmitted has an autosomal dominant inheritance pattern, with disease severity and age of onset correlating with the number of trinucleotide DNA repeats on chromosome 4. The neuro-degeneration associated with Huntington disease primarily affects the basal ganglia (especially the caudate nucleus) and the cerebral cortex.
The use of fMRI to study Huntington disease has primarily focused on two goals: characterizing the neural dysfunction and developing a biomarker for presymptomatic diagnosis. To assess the impact of Huntington disease on various neural domains, performance of functional tasks was assessed during fMRI and compared with that of healthy controls. Huntington disease patients were found to have widespread cortical and subcortical increased BOLD responses on maze performance and the Simon effect task, especially in motor and visual processing areas. A lack of motor response inhibition also correlates with patterns of impaired activation in the caudate and thalamus. The constellation of fMRI changes in activation and connectivity suggests that the neuro-degeneration or decreased activation observed in basal ganglia leads to compensatory recruitment (hyperactivation) of accessory motor pathways.
The development of a biomarker to predict the onset of Huntington disease in the presymptomatic stage is critical to improvements in disease management. Efforts to develop such a test based on the fMRI patterns previously described have led to a variety of potential measures, though further work is needed to validate these findings before clinical implementation can proceed. The task-related patterns of basal ganglia hypoactivation noted in symptomatic disease are preceded by a period of increased activation in the caudate, thalamus, and frontotemporal cortices, which likely signifies local compensation taking place in the setting of early neuro-degeneration. Activation of the left dorsal anterior insula during the processing of disgusted facial expressions was also absent in Huntington disease. These and numerous other changes on fMRI have been able to identify presymptomatic Huntington disease as early as 12 years before the estimated disease onset. Despite these promising results, it should be noted that all studies to date have reported these differences at the group level. Because of the high degree of variability inherent in presymptomatic Huntington disease, assessing risk of future disease at the single subject level is not currently possible.
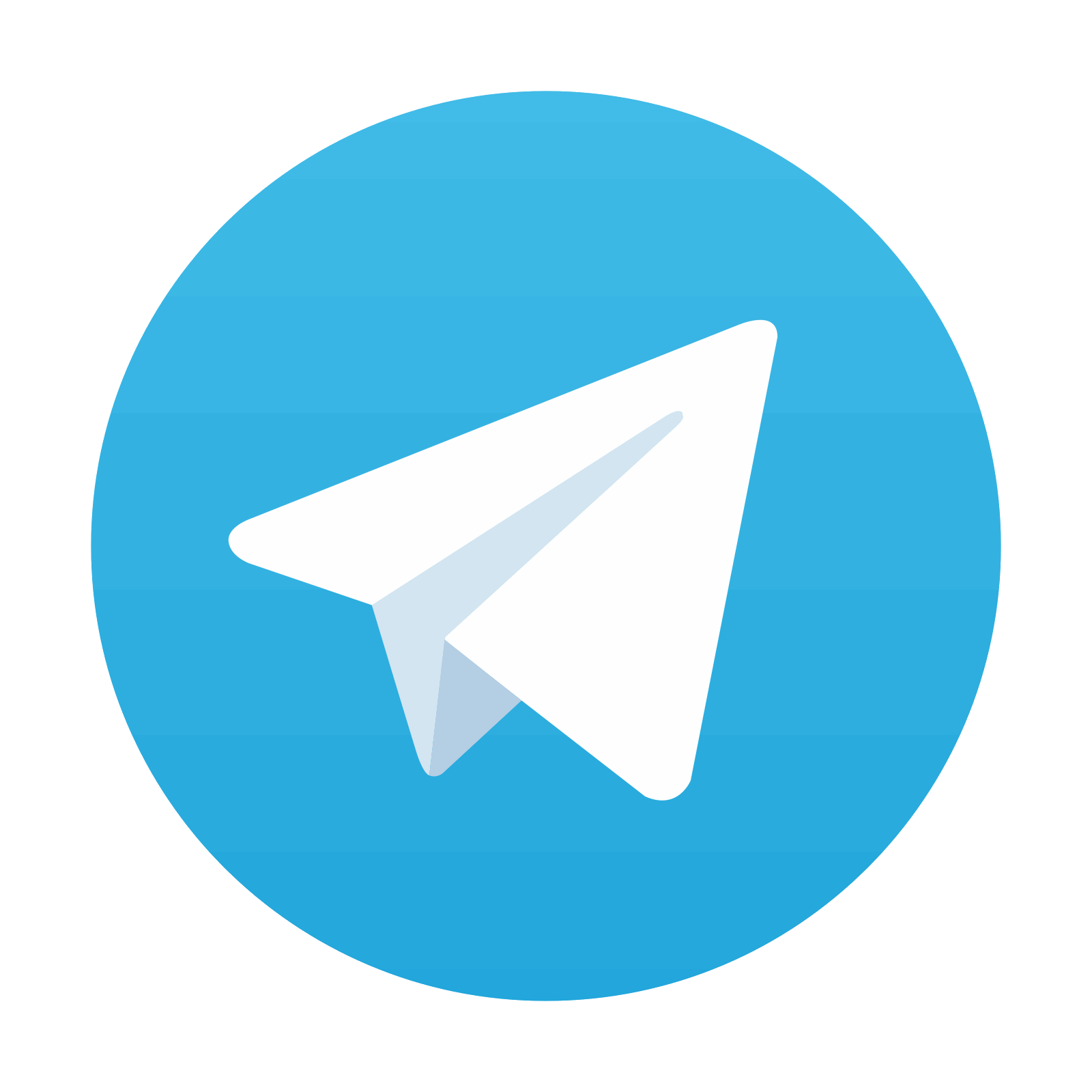
Stay updated, free articles. Join our Telegram channel

Full access? Get Clinical Tree
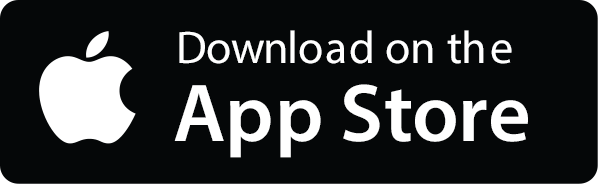
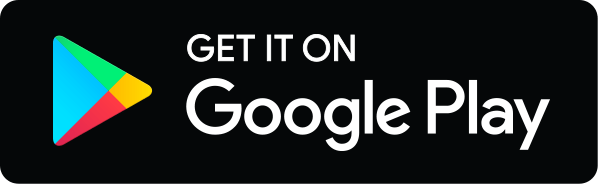