Clinical Presentation
The patient is a 63-year-old female patient with a history of two back surgeries in the distant past to remove herniated discs on the left at L5-S1. Patient has had chronic intermittent left lower extremity pain and numbness for many years since the surgery, attributed to known chronic recurrent L5-S1 disc herniation. She now presents with recent development of severe low back pain when bending forward and bilateral hip pain.
Imaging Presentation
Radiograph obtained reveals intervertebral disc space narrowing at L5-S1 associated with vertebral endplate sclerosis and marginal vertebral osteophyte formation ( Fig. 17-1 ) . Magnetic resonance (MR) imaging reveals an enhancing full-thickness posterior annular fissure (tear) at L4-5 and a right paramidline focal posterior annular fissure at L5-S1 ( Figs. 17-2 to 17-6 ) . A left paramidline L5-S1 herniated disc causes left anterolateral thecal sac deformity (see Fig. 17-6 ).
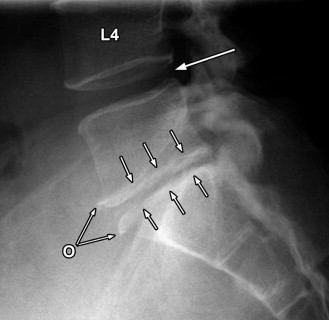
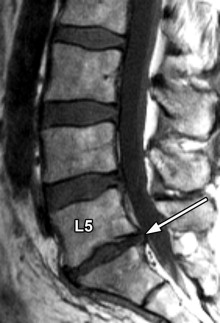
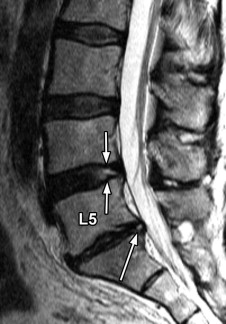
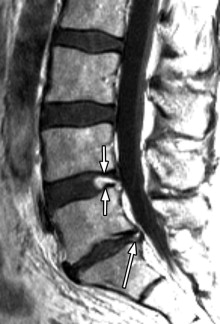
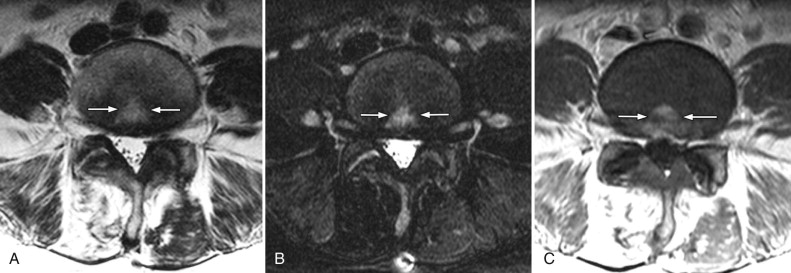
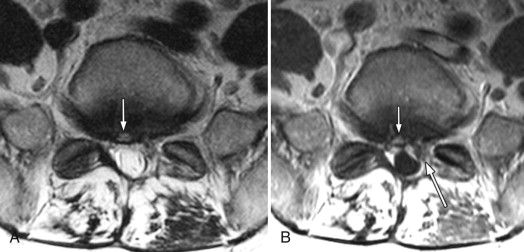
Discussion
Normal Intervertebral Disc
The intervertebral disc is a fibrocartilaginous structure comprised anatomically of a nucleus pulposus centrally, annulus fibrosus peripherally, and cartilaginous endplates superiorly and inferiorly ( Fig. 17-7 ) . The intervertebral disc is a fibrocartilaginous joint (it is not a synovial joint and therefore does not develop osteoarthritis). The intervertebral disc is composed of a mucopolysaccharide-rich ground substance, collagen bundles, chondrocytes, and water. The majority of the ground substance and water in the disc is located in the nucleus and inner portion of the annulus ( Fig. 17-8 ) ; 80% of the disc collagen is either type I or type II collagen, but small amounts of types III, V, IX, and XI collagen are also present in the disc.
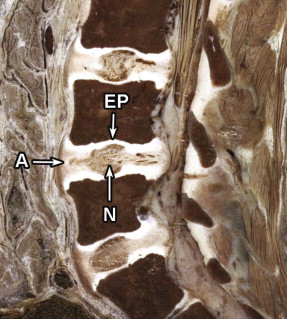
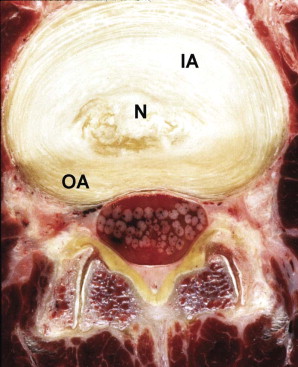
The nucleus pulposus is centrally located in the intervertebral disc and is gelatinous in nature, composed of approximately 85% water, 15% mucopolysaccharide material, and a tiny amount of type I collagen located at the nuclear equator called the reticular stratum . The mucopolysaccarides in the intervertebral disc are high-molecular-weight protein proteoglycans (also called glycosaminoglycans ). These proteoglycans include chondroitin sulfate, keratin sulfate, and small amounts of hyaluronic acid. The proteoglycans are negatively charged molecules that have an affinity to bind with water. The proteoglycans and water are concentrated in the nucleus and inner annulus. The amount of water in the disc at any given time is the result of a balance between hydrostatic forces from axial loading and intrinsic osmotic forces. The proteoglycans bind water in the disc, predominantly in the nucleus and inner annulus, and are responsible for the high intrinsic hydrostatic pressure of the normal intervertebral disc, which allows the disc to resist and distribute compressive (axial loading) forces. The disc height and intradiscal T2 MR signal intensity normally changes depending on the time of the day (diurnal variation). This diurnal variation in intradiscal MR signal intensity is less in degenerated discs. When a compressive load is placed upon the disc, water leaves the disc (predominantly through the endplates), and when the compressive load is removed, water returns to the disc. As much as 40% to 60% of the intradiscal water can be removed by mechanical compression of the disc in vitro.
The annulus fibrosus anatomically is divided into inner and outer regions ( Fig. 17-9 ) . The inner portion of the annulus contains less concentrated collagen, proteoglycans, and water and more closely resembles the nucleus pulposus histologically and on MR images; 80% of the collagen in the disc is type I or type II collagen. The outer annular collagen is predominantly type I collagen and is arranged in 15 to 25 concentric rings. These lamellae are not completely separate from adjacent lamellar rings, often splitting and obliquely joining adjacent lamella. Approximately 3% of the collagen in the annulus is type V collagen, which may play a role in regulating the spatial orientation and dimensions of the lamellae. Tiny, obliquely oriented or perpendicular interlamellar collagen bridges, visible on high-detail cryomicrotome images, also join the concentric lamellar rings ( Fig. 17-10 ) . The outermost portion of the annulus is mainly dense fibrocartilage, concentrically arranged into lamellar bundles of mainly type I collagen, similar to the fibrocartilage found in the meniscus of the knee. These fibers are T2 hypointense on MR images ( Fig. 17-11 ) . These outermost annular collagen bundles, often referred to as Sharpey’s fibers , are normally attached to the periosteum of the vertebral body corners, bone that was formed from the ring apophyes (see Fig. 17-9 ). The anterior and posterior longitudinal ligaments merge imperceptibly with the outermost fibers of the annulus ( Fig. 17-12 ) .
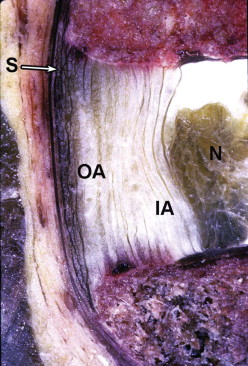
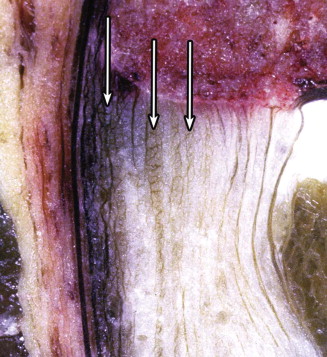
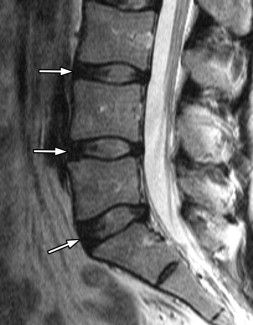
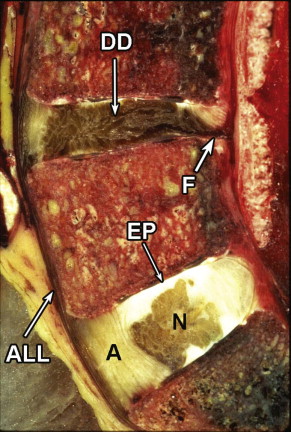
The collagen bundles in the outer annulus give the disc its tensile strength, offering resistance to radially oriented (shear) forces, which are produced when a compressive load is placed upon the nucleus and inner annulus. The outermost portion of the annulus is normally innervated by both somatic and autonomic (sympathetic) sensory fibers, many of which are unmyelinated. In degenerated discs, nerves may penetrate into the nucleus. As the disc degenerates , annular fissures develop and connective tissue containing blood vessels and nerve endings infiltrate these fissures. The nerves in this connective tissue have nociceptors that detect and transmit pain sensation responsible for discogenic pain.
The cartilaginous disc endplate is made up of a fine, loosely arranged fibrillar network of predominantly type II collagen, similar to articular hyaline cartilage (see Figs. 17-7 and 17-12 ). The vertebral cortical bone endplate and the cartilaginous disc endplate form a unit that serves to provide structural and nutritional support to the intervertebral disc. The cartilaginous endplate is in close proximity to a rich vascular network in and adjacent to the vertebral body endplates, which facilitates the exchange of intradiscal water, nutrients, and metabolic waste. The cartilaginous endplates also provide intradiscal support and resistance to compressive (axial loading) forces.
All portions of the adult intervertebral disc are normally devoid of blood vessels. Nutrients and metabolites, for disc nourishment, pass mainly by diffusion, but some pass by active transport from blood vessels that reside in small perforations located in the vertebral cortical endplates, and these substances pass across the cartilaginous endplates into the disc. Some water and nutrient exchange also occurs along the peripheral margin of the disc. It is believed that this nutrient exchange mechanism is facilitated by compression and decompression of the disc during normal daily activity. Interference with this nutrient exchange system by discovertebral infection, for example, causes damage to the vertebral endplates and results in destruction of the intervertebral disc.
Aging Disc
There is no general agreement as to what actually constitutes a normal aging disc versus a degenerated disc or when the transition from normal to aging to degenerated disc occurs. In persons of all ages, the collagen concentration is greater in the outer annulus. With increasing age, the relative collagen content increases in the disc from cephalad to caudad, and from peripheral to central.
The most striking changes in the intervertebral disc with advancing age occur in the disc nucleus and inner annulus. With age, the water content of the disc diminishes, but only by a relatively small amount. In children, 85% of the disc nucleus contains water, and 78% of the disc annulus contains water. Intervertebral discs in patients of advanced age still contain approximately 70% water. Therefore, it is estimated that the disc water content is reduced by only 15% to 20% from approximately age 10 to 70 in the “normally aging” disc. Therefore, the amount of disc desiccation that occurs in the normally aging disc is actually not that great on a percentage basis. In degenerating discs, a greater degree of disc dehydration is expected to occur.
The total proteoglycan content in the disc does decrease with age. However, the ratio of keratin sulfate to chondroitin sulfate in the disc actually increases with age. The collagen content in the disc increases dramatically with age and may be the primary reason discs appear “dark” on T2-weighted MR images. By age 80, the “normal” disc nucleus is almost completely replaced by dense fibrocartilage, but the disc height remains preserved.
It is possible that some small annular fissures can occur as a result of the normal aging process. Small annular fissures are sometimes observed in anatomic specimens of the disc in persons 15 to 20 years of age. By age 30, annular fissures are found in nearly all anatomic disc specimens.
Degeneration of the Intervertebral Disc (Disc Derangement)
There is no general consensus or unifying theory as to what actually causes or constitutes intervertebral disc degeneration or disc derangement . Disc degeneration is likely caused by multiple interactive factors. Many believe that the degenerated, internally deranged disc is the result of acceleration of the normal aging process. It is likely that genetic factors or some metabolic dysfunction renders the disc susceptible to chronic derangement or acute disruption. In degenerating discs, the water content is definitely reduced, but it is uncertain whether water reduction occurs primarily or is secondary to reduction in proteoglycan concentration, because proteoglycan synthesis and water binding in the disc are interrelated. In the aging and degenerating disc, it is also known that collagen, which predominates normally in the disc periphery, infiltrates the inner annulus and nucleus replacing portions of the disc previously occupied by proteoglycans and water. Dehydration of the disc likely predisposes it to annular fissure formation, but the exact mechanism by which annular fissure formation occurs is not known. In the end stages of disc degeneration, the disc is replaced almost entirely by amorphous fibrocartilage with no distinction between nucleus and annulus (see Fig. 17-12 ).
An acute traumatic episode can disrupt the disc but is not likely the major factor that produces disc degeneration, as was once believed. A history of trauma is obtained in only a minority of patients who have herniated intervertebral discs. Biomechanical studies have shown that the disc is less likely than the vertebral body to fail as a result of trauma. However, repetitive microtrauma may cause damage to the osteocartilaginous endplate or intervertebral disc, which may eventually weaken the disc and make it unable to withstand daily biomechanical stress.
Because the intervertebral disc normally lacks a blood supply, nutrients and water are exchanged between capillaries and the extravascular spaces in the vertebral bodies and epidural space via the osteocartilaginous endplates and outermost annular disc margins, which function similarly to semipermeable membranes. Metabolic disorders or inflammatory processes that damage or interfere with the exchange of water and nutrients across the endplates or at the peripheral disc margins may therefore play a role in the pathogenesis of disc degeneration.
There is mounting evidence that genetic factors predispose the disc to degeneration and hence disc herniation. Inherited genetic traits likely render the disc more susceptible to degeneration, disc herniation, or weakening of the vertebral bone. Cytokines, such as interleukin-1, may contribute to disc degeneration by causing release of enzymes that degrade proteoglycans. Inflammatory cytokines are believed to be one of several mediators of back pain. Enzymes capable of disc degradation, such as metalloproteinases and aggrecanase, occur in high concentrations in degenerating discs and in even higher concentrations in herniated discs.
Regardless of the pathogenesis of disc degeneration, the result of the biomechanical stress placed upon the disc either acutely or chronically is the development of annular fissures (annular tears). Radially oriented fissures, in particular, are believed to be associated with primary biomechanical failure of the annulus, and these annular tears are consistently found in degenerated symptomatic and asymptomatic intervertebral discs viewed with discography. Radially oriented partial or full-thickness annular tears extend across and disrupt the lamellar rings ( Fig. 17-13 ) . Fissures that disrupt the lamellar rings should always be considered pathologic, and their presence always indicates internal disc derangement. These radially oriented fissures often involve the inner annulus initially and later extend to involve the outer annulus. Annular fissures most often occur with concomitant reduction in disc height and are often associated with discogenic pain because they contain vascularized granulation tissue with nerves that have nociceptors that transmit pain sensation.
