Basic Principles of Noninvasive Cardiovascular Imaging
M. Schaefers
R. Fischbach
The spectrum of cardiovascular imaging encompasses morphologic, functional, and molecular imaging modalities. Conventional angiography of the coronary arteries supplies morphologic information on vascular anatomy and hemodynamically significant stenoses. Left ventricular angiography can assess global and regional ventricular function. Angiography also has a key role in the planning and conduct of revascularization procedures.
CT. Multislice CT has assumed growing importance in the diagnosis of coronary heart disease. CT can sensitively detect sites of coronary artery stenosis, and it can directly visualize the artery wall and atherosclerotic plaques (Budoff et al. 2008; Gallagher et al. 2007, Vanhoenacker et al. 2007). It can also provide an accurate analysis of ventricular function comparable to the reference standard MRI (Juergens and Fischbach 2006).
Echocardiography. Echocardiography is a universally available and risk-free procedure that has become the clinical standard for morphologic and functional cardiac imaging. Potential limitations in clinical routine work are its examiner dependence and anatomical factors (acoustic windows).
MRI. The high temporal resolution of MRI, together with its excellent soft-tissue and contrast resolution, havemade MRI an important modality for cardiac imaging. Its clinical applications include myocardial perfusion imaging, high-resolution imaging of scar tissue, and delayed enhancement myocardial imaging (Lotz et al. 2009).
SPECT and PET. SPECT and PET are scintigraphic techniques that have been used for years in the assessment of myocardial viability, perfusion, and perfusion reserve (Schober 2007). Not only can nuclear medicinetechniques evaluate parameters of cardiac and vascular function, but currently they are the only techniques capable of visualizing and quantifying molecular processes in vivo. An established clinical example is myocardial viability assessment with [18F]FDG, which is incorporated into myocytes by glucose transporters, is phosphorylated by hexokinase, and accumulates inside the cells. A positive FDG signal can distinguish a viable population of myocardial cells from scar tissue. As a result, these techniques have become the gold standard for assessing myocardial viability prior to revascularization. The molecular imaging modalities of SPECT and PET are also very versatile owing to the potentially limitless number of radiolabeled molecules and molecular targets, and they have attracted great interest as tools for future cardiovascular imaging.
A crucial piece of information, the detection of ischemia, is gained by nuclear medicine techniques. Ischemia is a prerequisite for the performance of revascularization procedures.
All the imaging modalities mentioned above yield one main type of information (morphologic, functional, molecular) and have a particular range of diagnostic applications. No one modality can adequately address all clinical and scientific questions (Fig. 4.1). This accounts for the great potential of multimodal imaging systems such as PET-CT, SPECT-CT, and MR-PET, which permit the combined evaluation of morphology, function, and molecular properties in a single study.
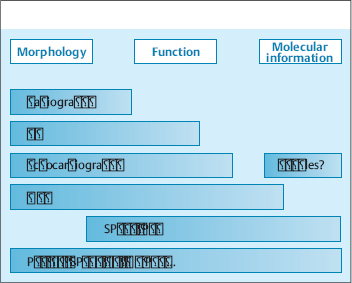
Basic Principles of Noninvasive Cardiovascular Imaging
Pathophysiology. Cardiovascular diseases are the leading cause of death in industrialized countries. Approximately one-third of all deaths in the US in 2006 (831000) had a cardiovascular cause. This high mortality is due chiefly to atherosclerosis of the coronary vessels, which leads to coronary heart disease and its complications—chest pain (angina pectoris) and myocardial infarction. While atherosclerosis is a chronic inflammatory disease of the vessel wall (atherosclerotic plaque), its most lethal complication, acute coronary syndrome, results from the sudden thrombotic occlusion of a coronary vessel, usually triggered by the mechanical disruption of atherosclerotic plaque. Atherosclerotic plaques that are especially prone to rupture are described as “vulnerable” or “unstable.” The rupture of vulnerable plaques is responsible for over 70% of all thrombosis-related coronary deaths throughout the world (Naghavi et al. 2003).
Diagnostic goals. The noninvasive diagnostic identification of vulnerable atherosclerotic plaques is one of the most important challenges in modern medicine. Morphology-based imaging modalities such as catheter angiography and CT do provide high-resolution morphologic information on the narrowing of coronary vessels by atherosclerotic plaque, but they do not furnish information on plaque stability. Unstable plaques are often deceptively nonocclusive and are therefore difficult to detect by imaging the vessel lumen. More than half of all plaques that precipitate a heart attack cause little or no narrowing of the coronary artery lumen (Falk et al. 1995). As a result, angiographic techniques cannot predict the individual risk of heart attack with an acceptable degree of confidence.
Molecular imaging offers new approaches to this problem. The goal is to identify metabolic processes that predict plaque rupture by noninvasive means. In addition to other modalities, scintigraphy, PET, and SPECT provide high sensitivity and are clinically available. The combination of PET and CT (PET-CT) is an attractive and innovative approach owing to its ability to provide simultaneous, spatially coregistered morphologic and molecular information.
Besides the diagnosis, classification, and evaluation of treatment response in coronary heart disease, noninvasive multimodal imaging strategies will be important in other cardiovascular diseases as well. Examples are heart failure, which is becoming more prevalent in the aging population owing to advances in acute therapy (e.g., for myocardial infarction), and cardiac arrhythmias, in which purely morphologic imaging techniques are often unrewarding (idiopathic heart disease). It is reasonable to predict that the combination of noninvasive morphologic, functional, and molecular imaging will bring significant progress in the diagnosis, classification, and monitoring of these diseases.
PET
The working myocardium (contraction), the coronary arteries (myocardial perfusion), and the often disregarded issue of myocardial innervation are all essential in maintaining and controlling cardiac function. Specific PET techniques, some of which have been available for years, can be used to evaluate each of these aspects of cardiac performance. Table 4.1 reviews clinically established techniques of cardiac PET imaging.
Evaluation of Myocardial Function
Myocardial viability and confirmation of ischemia are the most important clinical issue in the PET evaluation of myocardial function, especially when selecting patients for revascularization procedures. Scar tissue can be distinguished from ischemic but viable (“hibernating”) myocardium on the basis of abnormal wall motion. Only viable ischemic myocardium will benefit functionally from restoration of perfusion (Schäfers 2002). FDG PET is best suited to the assessment of myocardial viability. Generally the FDG PET finding is compared with a PET perfusion study or myocardial perfusion SPECT so that typical match/mismatch findings can be obtained and analyzed.
Compartment | Investigation | PET radiopharmaceutical |
Working myocardium | Glucose metabolism | [18F]FDG |
Fatty acid metabolism | [18F]Fluoropalmitate | |
Oxidative metabolism | [11C]Acetate | |
Coronary arteries | Perfusion, perfusion reserve | [15O]water, [13N]NH3, 82Rb |
Autonomic innervation | Sympathetic nervous system, presynaptic | [11C]Hydroxyephedrine |
Sympathetic nervous system, postsynaptic | [11C]CGP 12177 | |
Parasympathetic nervous system, postsynaptic | [11C]MQNB |
CGP, Choline glycerophospholipid; FDG, fluorodeoxyglucose; MQNB, methylquinuclidinyl benzilate.
A postinfarction scar shows no evidence of FDG up-take, and so an FDG defect in the myocardium indicates s arring. This means that the patient would not benefit from revascularization. On the other hand, the presence of FDGuptake in the myocardium is a definite sign of viable but often ischemic tissue. In this case a perfusion studyshould be performed for comparison (Fig. 4.2). These areas of the myocardium will benefit from revascularization, showing a postoperative improvement in pump function and a reduced risk of cardiovascular events. FDG PET has positive and negative predictive values in the range of 90%–95% for predicting the recovery of pump function after revascularization. ECG-gated acquisition of FDG PET scans additionally provides data on ventricular volumes, ejection fraction, and regional wall motion that can further improve the assessment of myocardial viability.
Perfusion Imaging
Perfusion and perfusion reserve. The most frequent current application of PET in clinical cardiovascular diagnosis and research is the investigation of coronary perfusion and perfusion reserve. The radiopharmaceuticals most commonly used for PET imaging of myocardial perfusion are [13N]ammonia ([13N]NH3), [15O]water ([15O]H2O) and rubidium 82 (82Rb). These PET perfusion agents differ in their physiologic parameters and imaging characteristics.
[15O]Water diffuses freely through tissue membranes and is not subject to energy-dependent transport. This makes it an ideal radiopharmaceutical for the absolute quantification of myocardial blood flow over a wide range of flows. A [15O]water perfusion scan is illustrated in Fig. 4.3.
The potassium analogs 82Rb and [13N]NH3 undergo a proportional myocardial uptake in low- to moderate-flow regions, but their rate of extraction from the blood decreases in high-flow regions. [13N]NH3-PET yields excellent perfusion images of the heart that can be visually analyzed. For this reason, [13N]NH3 is the radiopharmaceutical of choice for routine clinical tests. It can also be used for the quantification of blood flow. Given the capacity for the absolute quantification of perfusion (in mL/min per gram of myocardium), pharmacologic and ergometric stress protocols are of special interest as a means for determining the perfusion reserve.
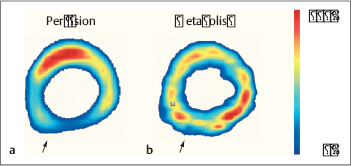
Fig. 4.2a, b Coronary heart disease.
Perfusion SPECT (a) and FDG PET (b). The perfusion image shows decreased resting perfusion in the posterior left ventricular wall (a, arrow), while the FDG PET image shows preservation of glucose metabolism in the affected myocardial segment (b, arrow). This “perfusion-metabolism mismatch” is typical of dysfunctional but metabolically viable hibernating myocardium.
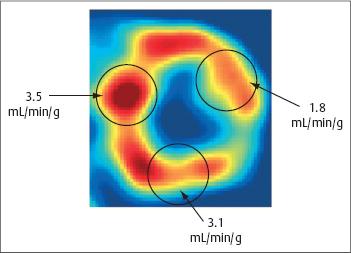
Fig. 4.3 Quantification of myocardial parfusion using PET.
This case illustrates a midventricular short-axis scan (factor image from a dynamic [15O]water scan) with ROIs and the results of quantification with the single-compartment model.
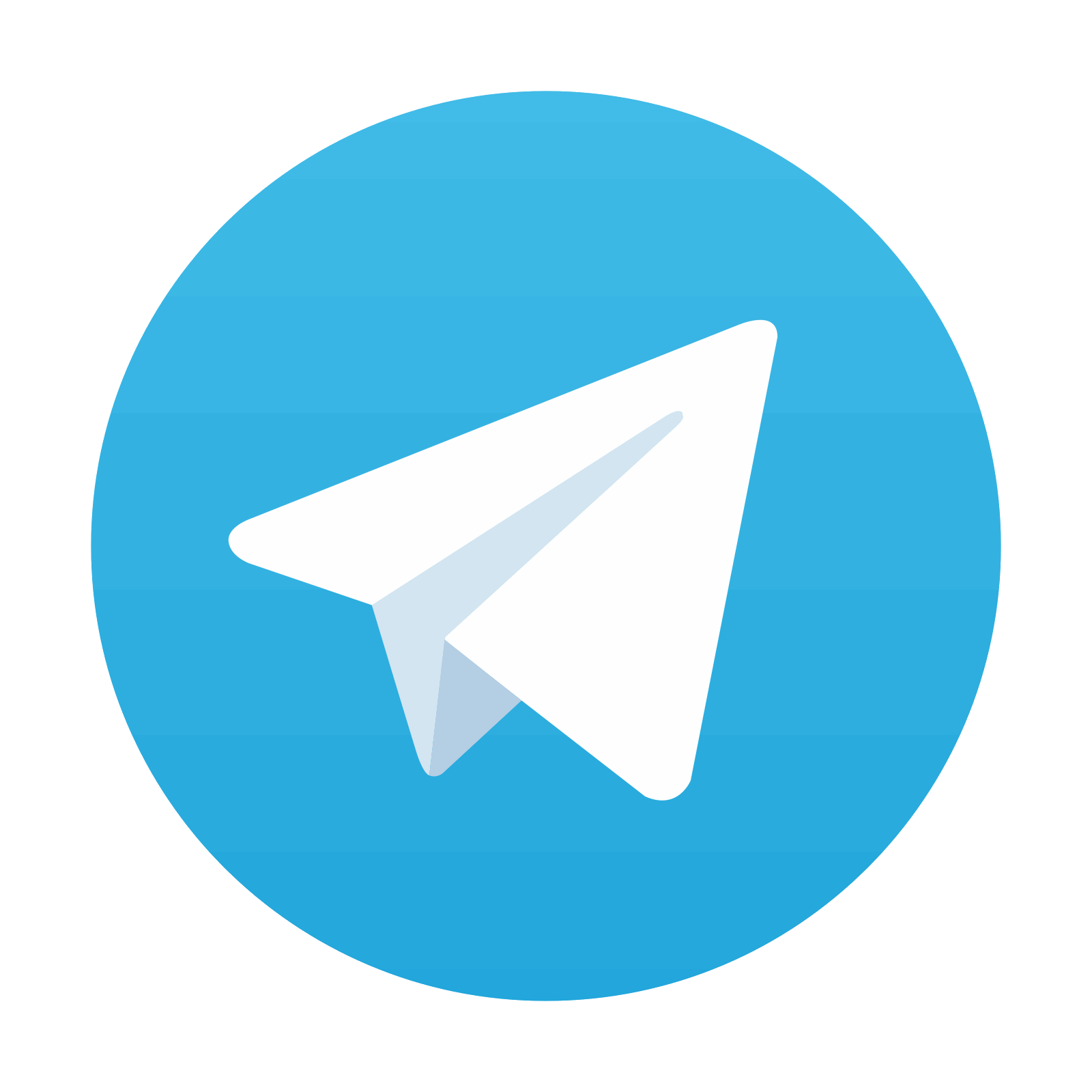
Stay updated, free articles. Join our Telegram channel

Full access? Get Clinical Tree
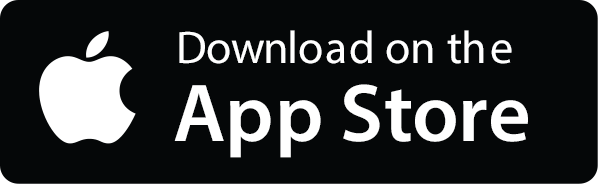
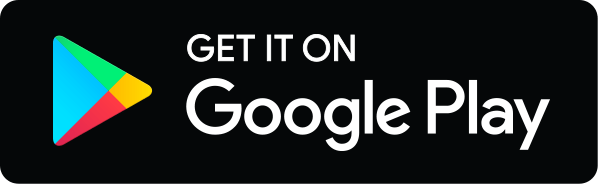