Fig. 45.1
(a) The equine and human skeleton. The equine distal limb (fingers and toes) is a reduced version of the human skeleton, e.g., consisting of one fully developed metacarpal/metatarsal bone and one proximal, middle, and distal phalanx. Note that the carpus and the tarsus are located halfway the fore- and hind limb. (b) The horse in real life
The equine musculoskeletal system incorporates several energy-saving mechanisms based on the elastic properties of tendons, which allow them to store elastic energy when being stretched and released upon recoil (Fig. 45.2). There are two of these elastic tendons arranged on the flexor side of the equine leg (i.e., the superficial and deep digital flexor tendons) traveling all the way from the distal end of the humerus/femur to the digit, crossing several joints, protected by tendon sheaths (type II tendons) and supported by their respective accessory ligaments (commonly known as the superior and inferior check ligament) and the ligamentous third interosseous muscle (commonly known as the suspensory ligament). The relatively straight horse leg changes direction dramatically in the metacarpo(tarso)phalangeal joint, which is hyperextended in the standing horse. During locomotion this joint becomes even more extended and causes the flexor tendons to stretch when the leg is on the ground; when the leg leaves the ground, the tendons relax and release their energy. Sesamoid bones at the level of the metacarpo(tarso)phalangeal and the distal interphalangeal joint (navicular bone) increase the distance between the joints and the flexor structures, thus increasing their lever arm. While these evolutionary modifications are advantageous for lowering the costs of locomotion, they come at a price. Light distal limbs and elastic tendons have small cross-sectional areas thus undergoing high stresses and hence being prone to injuries. The third metacarpus and proximal phalanges are the most common sites of fractures in racehorses, and the superficial digital flexor tendon is the most commonly affected soft tissue structure. Sesamoid bones come under high pressure by the flexor tendons wrapping around them, and this can lead to pathology. Disorders associated with the navicular bone and the deep digital flexor tendon are extremely common.
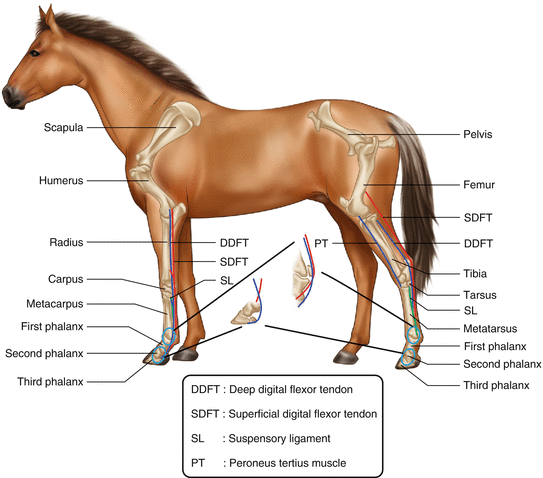
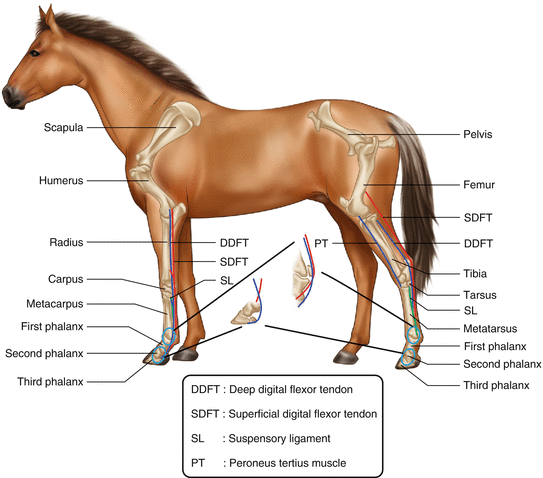
Fig. 45.2
Schematic representation of the equine flexor tendons. DDFT deep digital flexor tendon, SDFT superficial digital flexor tendon, SL suspensory ligament, PT peroneus tertius muscle, 1 scapula, 2 humerus, 3 radius, 4 carpus, 5 metacarpus, 6 first phalanx, 7 second phalanx, 8 third phalanx, 9 pelvis, 10 femur, 11 tibia, 12 tarsus, 13 metatarsus, 14 first phalanx, 15 second phalanx, 16 third phalanx
The anatomy of the neck, back, pelvis, and upper limb is basically comparable with human anatomy. Comparable to man, conformational limb defaults may predispose the individual to maladaptive musculoskeletal alterations or injuries. Despite the fact that most abnormalities can be considered as negatively influencing factors for all sport activities, some play a more dominant role depending on the type of activity (e.g., hyperextension of the hind limb fetlock predisposes dressage horses, already vulnerable to suspensory ligament (SL) injuries in the hind limb, to this type of lesion. Racehorses, prone to maladaptive remodeling of the carpal bones, with concave formation of the carpus are at increased risk to develop fractures in this area).
45.3 Diagnostic Imaging
The imaging modalities available for horses are the same as used in humans, including radiography (Fig. 45.3), ultrasonography (Fig. 45.4), computed tomography (CT) (Fig. 45.5), magnetic resonance imaging (MRI) (Fig. 45.6), and scintigraphy (Fig. 45.7). However, the application of these modalities differs considerably between man and horse due to the sheer size of the animal. Radiographic assessment is increasingly performed in the field using portable digital radiography systems. Digital radiography systems are now small and affordable and have become a standard piece of equipment for ambulatory vets. Hospital-based systems for horses use the same generators and registration systems (film screen/CR or DR systems) as human hospitals but are mounted differently to allow the use of horizontal and angled x-ray beams. Ultrasonography is considered a quite cheap modality, and nearly all veterinary practices have an ultrasound machine. This modality is strongly “operator dependent,” but each practitioner can decide for himself/herself for which indication he/she will use it depending on training and knowledge. Ultrasonography is used a lot in the practice mainly for orthopedic injuries but also for any body part giving an appropriate acoustic window. For orthopedic disorders, linear 7.5–15 MHz (tendons, small joints, e.g., fetlock, tarsus), microconvex 6–9 MHz (feet, back, pelvis), or convex 5–8 MHz (large joints, e.g., stifle, hip) transducers are used. In adult horses, tomographic modalities (MRI, CT) are limited to the head, cranial part of the neck (till C5), and (distal) limbs due to the limited gantry opening (60–70 cm). For the rest of the neck, back, pelvis, and the majority of the upper limb, only radiography, ultrasonography, and scintigraphy are used because they can technically be performed in these areas, not because they are the best choice for the suspected disease. Computed tomography is used at some specialist centers to allow accurate assessment of fracture configuration before surgical repair. The same systems are used in equine than in humans but with a custom-built horse table that allows loads of up to 2 t to accommodate horses. The head and neck can be scanned with the horse standing, while for the limbs, general anesthesia/recumbent positioning is required, which is not undertaken lightly due to the associated high risk of potentially fatal complications (especially at recovery). The most frequently used MRI system in horses is an open low-field magnet 0.27 T MRI system developed by Hallmarq (Hallmarq Veterinary Imaging Ltd). This system is operating with the horse standing and it allows imaging of the foot and lower limb. Other open or closed magnets for equine MRI are the scanners used in human medicine, with field strengths from 0.2 to 3.0 T, and they require general anesthesia and recumbent positioning. Due to the noncooperative nature of many equine patients, scintigraphy is usually performed in the standing, heavily sedated horse, often resulting in “swaying,” necessitating the application of motion correction software. For this same reason, dynamic images are acquired. The mounting of the gamma camera requires special modification to allow movement of the camera around the whole patient. This is usually achieved by suspending the camera from a railing system or the use of a forklift. The examination is time consuming, especially in cases where lameness cannot be attributed to a specific limb, because this requires total body imaging. Contrary to human patients, the classical total body acquisition is not possible, due to the size of the animal, and planar images of all the to-be-evaluated regions have to be obtained. Single-photon emission tomography (SPET) is not performed up till now because this necessitates general anesthesia and the limited gantry opening of conventionally used systems allow only investigation of selective parts of the equine body.
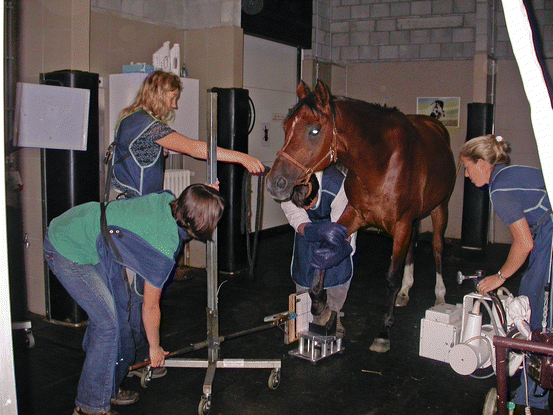
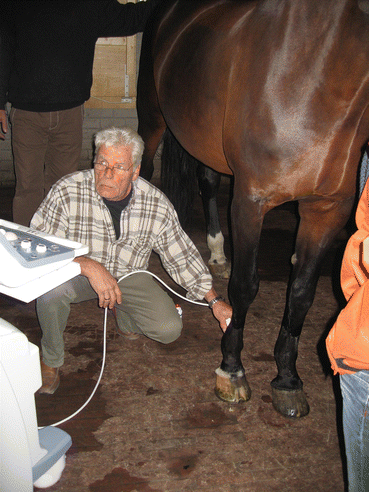
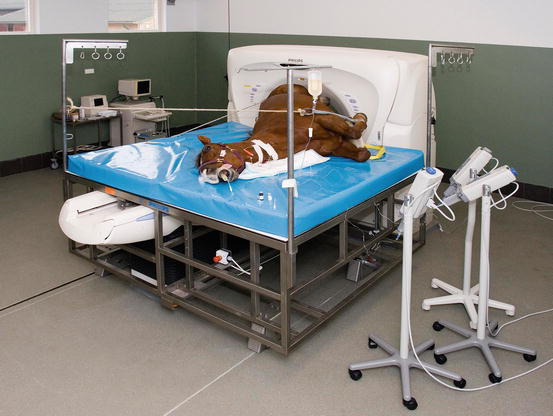
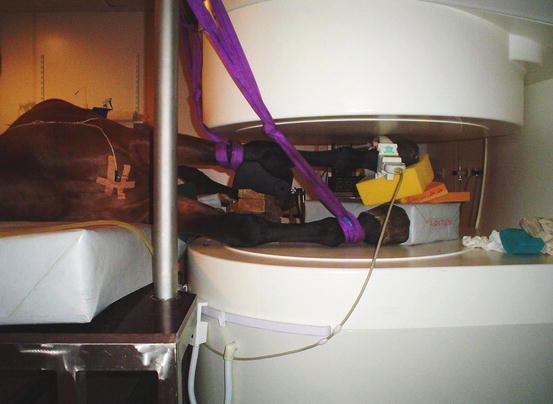
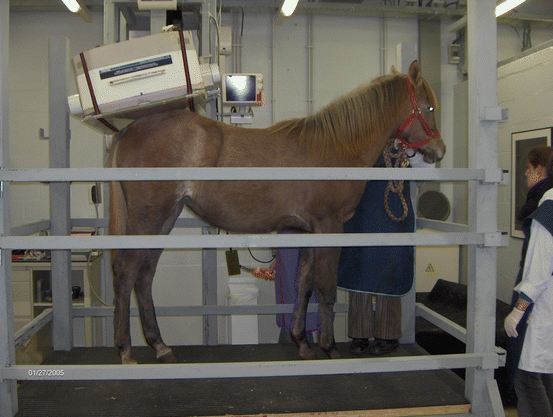
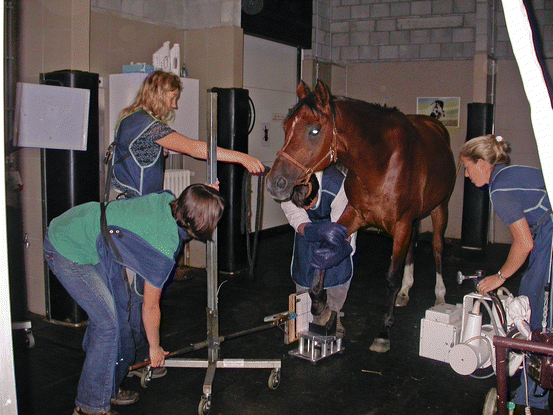
Fig. 45.3
Setup of a radiographic examination of the equine foot
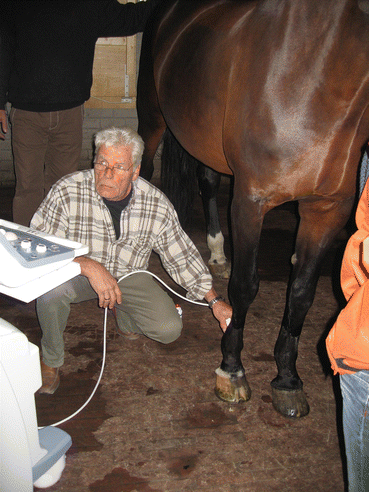
Fig. 45.4
Ultrasonographic tendon examination (the area under investigation is shaved)
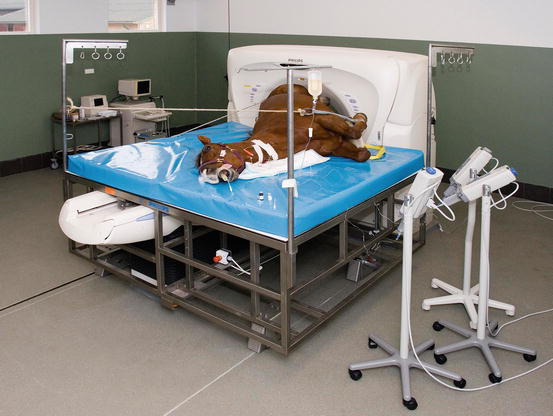
Fig. 45.5
Computed tomography of the equine stifle under general anesthesia
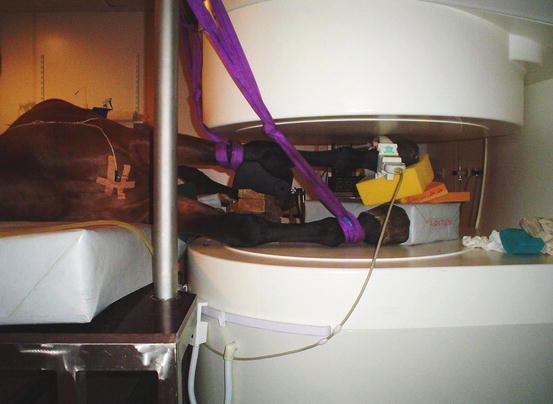
Fig. 45.6
Magnetic resonance imaging (open magnet system) of the equine distal right front limb under general anesthesia
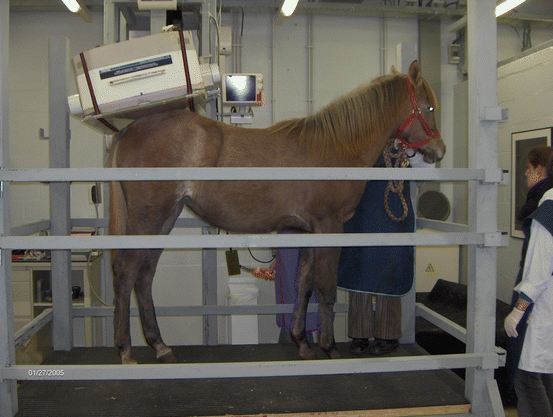
Fig. 45.7
Scintigraphic examination of the equine pelvic area
Preparation of the patient regarding urine contamination is a necessity. The lower limbs are bandaged and the hoofs are taped (Fig. 45.8). During the subsequent waiting period, the horses are stabled on shavings to increase the absorbing potential of the beddings and decrease contamination of the limbs. Some institutions use diuretics to induce voiding during this waiting period; however, this is considered a controversial procedure. During acquisition urinating may also occur as a result of the sedative, and containers to collect contaminated urine have to be in the immediate vicinity. After a scintigraphic examination, the horse remains hospitalized in an isolated stable for 24–48 h, and neither the horse nor its bedding is touched during this period (unless there is a medical emergency). The bone scan is extremely sensitive for imaging of bone (re) modeling. Increased bone metabolism may be the result of both adaptive and maladaptive bone modeling. Similar to humans, uptake can be seen in different strain-exposed areas that are not necessarily associated with pain (Drubach et al. 2001), and the findings on the bone scan have to be interpreted in the light of clinical findings and regional analgesia results. Scintigraphic examination is most valuable in the acutely lame animal and less in cases of chronic mild lameness or in horses with vague complaints such as decreased or suboptimal performance. The so-called false-positive scans may occur as a result of adaptive bone remodeling in sport-related predilection sites. Studies have been conducted in horses with different athletic activities to define the regions that show “physiologic” or sport-related stress-induced bone remodeling. These regions are however also potential sources for maladaptive bone alterations. Negative studies may be encountered when the lesion is primarily tendinous or ligamentous. False-negative scans may be due to suboptimal scan quality (camera distance to the region under investigation, insufficient counts, limited views) or due to vascular compromise. The “cold leg” phenomenon is well recognized in the equine patient. It is the result of the relatively poor perfusion to the lower limb, and preventative measures include bandaging of the lower limb or exercising the horse prior to the investigation (which is not without risk when a fracture is suspected). False-negative scans may also occur following traumatic injuries, especially in the pelvic area, a region additionally affected by image degrading factors such as motion, attenuation due to the heavy muscles overlying this area, and background radiation from the urinary bladder. It may take days before osteoblastic activity results in increased uptake (maximal tracer uptake occurs 8–12 days after bone injury in general but has not yet been studied in the horse) (Ross 2005). Severe disruption of the vascular supply may additionally hamper the regional delivery of the tracer. Scintigraphy is an expensive procedure; however, athletic horses are expensive to buy and even more expensive to train, which makes scintigraphic monitoring of these athletes worthwhile. The use of radioactive substances and the necessary legal radioprotective issues (which show variation among states and countries) limit its use to licensed institutions.
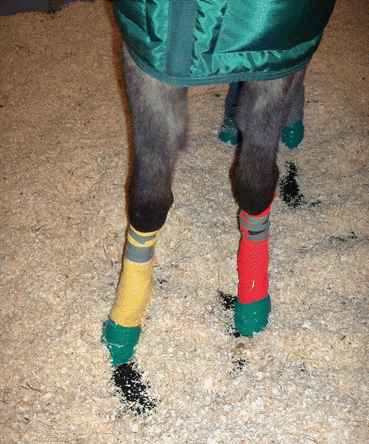
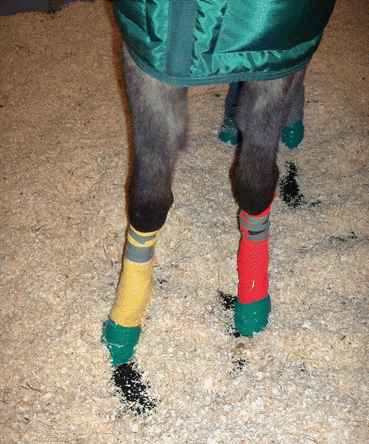
Fig. 45.8
Urine contamination prevention by bandaging the lower limbs and taping the feet
45.4 Diagnosis of Musculoskeletal Disorders
The diagnosis of orthopedic disorders can be challenging. Horses do not communicate orally and are less cooperative than humans. Therefore, the first mandatory step in the diagnostic process is to localize the site of pain. This process is quite different compared to adult human medicine and is comparable to small children medicine and starts with a clinical examination including observation of the standing and moving horse; palpation of the musculoskeletal structures; manipulation to elicit a pain response, e.g., flexion of joints; and systematic analgesia of specific nerves and/or joints from distal to proximal. The clinical examination can be compromised by the noncooperative nature of the patient and by nonspecific findings or may be limited by the nature of the suspected disease, e.g., systematic analgesia, and trotting a horse is contraindicated if fissures/fractures are suspected. The clinical findings guide the choice of the imaging modality subsequently used to characterize the cause of pain further, inform prognosis, and allow the appropriate choice of treatment.
45.4.1 Injuries of the Appendicular Skeleton
45.4.1.1 Bone Injuries
Bone injuries may result from traumatic events, such as a fall or collision, but are much more commonly the consequence of chronic repetitive overload causing “stress” remodeling and ultimately fractures. Risk factors for falls and related injuries during races have been distillated particularly in racehorses, and length of race, age, and race experience of the horse play an important role. Risk for fractures increases when horses do not perform fast exercise during training or when they are in their 1st year of racing, with increasing race distance and firm/hard race ground conditions. Increasing distance at canter and gallop in short time periods in previously untrained animals increases the risk for tibia and pelvic fractures (review: Clegg 2011).
Traumatic fractures can occur in any bone but are more commonly seen in the lower part of the limbs. These fractures can usually be visualized with radiography (Fig. 45.9a, b). Computed tomography is sometimes performed presurgically in complex fractures allowing multiplanar two-dimensional and 3D reconstruction of the fracture. Both CT and MRI are useful in the assessment of acute distal phalanx fractures that are not always easy to spot on radiographs.
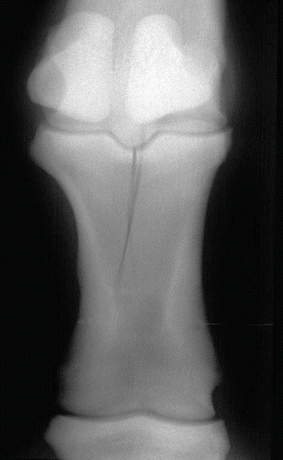
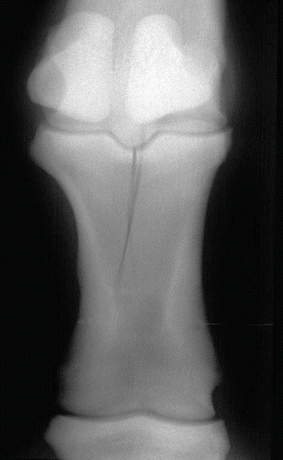
Fig. 45.9
A typical example of an incomplete proximal phalanx fracture
Stress-induced bone changes are the result of a mismatch between training-induced load and bone metabolism causing microfractures and changes in bone material properties. Stress-induced (re)modeling can be found in cortical bone as well as in cancellous bone, leading to alterations in bone microstructure, microfractures, and finally overt fractures. In horses with subchondral stress-related bone injuries, osteoarthritis is also a possible sequel. These stress-induced bone changes are most commonly, but not exclusively, observed in athletic horses performing at high speed such as gallop, trotting, and pacing racehorses. Remodeling changes have been found in horses suffering fractures of the humerus, pelvis, carpus, and distal metacarpus/metatarsus, indicating underlying pathology (review: Davidson and Ross 2003). The changes observed form a continuum, and it is not always easy to determine where the transition between adaptive and maladaptive bone changes lays. In early stages of disease, the horses may only show a loss of performance, while in more advanced cases, the horse will be overtly lame, ranging from mild (often bilateral) to severe lameness, once the changes have progressed to a proper fracture. The most common sites for stress remodeling are the third and radial carpal bone, the dorsal cortex of the metacarpus/metatarsus, and the distal metacarpal/metatarsal epicondylar region (Ross 1998). Concave conformation of the carpus (“back at the knee”) predisposes to carpal bone injury (Fig. 45.10a, b). Maladaptive changes result in typical slab or chip fractures in the radial carpal bone and the third carpal bone. Stress remodeling of the dorsal cortex of the metacarpus (dorsal metacarpal disease, DMD, or “bucked/sore shins”) is often seen in young Thoroughbreds at the start of their training. To avoid the risk to develop DMD, gradual introduction of high-speed exercise has been recommended in order to induce an adaptive bone response (Clegg 2011). In most cases, DMD subsides with less intensive training. Stress fractures in the distal metacarpal/metatarsal regions may be accompanied by first phalanx fractures, a combination that usually finalizes the horse’s sporting career. Stress remodeling and overt fractures are, similar to human running athletes, also seen in the tibia and femur.
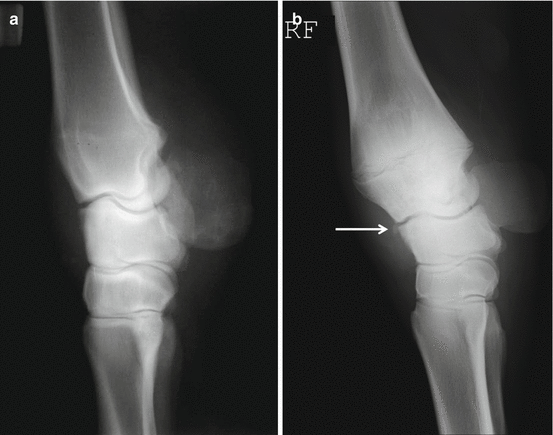
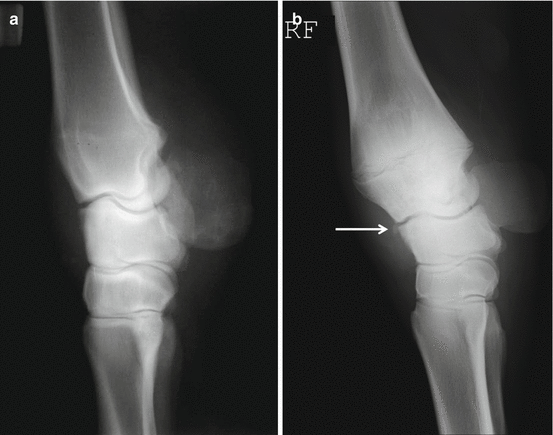
Fig 45.10
(a) Lateral (lateral to medial) radiograph of the normal carpal conformation. (b) Lateral radiograph of a carpus showing the typical “back at the knee” conformation with a chip fragment dorsal to the radiocarpal joint (arrow)
Clinical signs of cortical bone remodeling are periosteal thickening and local pain. However, some regions can be difficult to palpate due to overlying muscles (e.g., lateral tibia). Regional analgesia carries a risk for development of a complete fracture and has to be performed with caution. Regional analgesia of the upper limb regions (above carpus and tarsus) is insufficient or impossible. Clinical features of subchondral remodeling are variable. In horses with early disease, overlying joint cartilage will not be affected and clinical symptoms related to joint disease may be lacking. Focal sensitivity may not be present and intra-articular analgesia may be negative.
The value of radiography in stress-related bone remodeling is limited in the early stages due to its low sensitivity in depicting changes in bone density, while in more advanced stages, radiography is very useful to demonstrate periostitis, sclerosis, and fissure or fracture lines. In general, the use of radiography is limited by the size of the animal in the more proximal regions such as the pelvis. Also, radiography is not able to discern clinical silent disease from active bone remodeling. Scintigraphy has an excellent sensitivity in depicting changes in bone metabolism and is the imaging modality of choice to assess horses with stress-induced bone changes (Fig. 45.11). Planar images are acquired 2.5–3 h after injection of Tc99m-methylendiphosphonate at a dose of 1GBq/100 kg (a standard racehorse would require about 5GBq). Scintigraphy allows identifying changes at a very early stage and consequently can be used to alter the training regime in order to prevent progression of disease. Scintigraphy may also be of help to recognize subtle radiographic changes and to determine clinical significance of findings. The use of scintigraphic patterns of uptake in the tibia, as is used in humans, to define the severity of pathology and predict the duration of decreased training regimes, has been reported to be of no use in the equine athlete (Ramzan et al. 2003). It is of paramount importance that an adequate number of high-quality views are taken. Scintigraphic findings are often a helpful guide for subsequent use of other imaging modalities, mainly ultrasonography. The bone scan has also been used to determine specific areas subject to stress-related bone remodeling depending on the type of sport activity (Davidson and Ross 2003; Ehrlich et al. 1998). Magnetic resonance imaging is useful in the detection of bone pathology such as bone edema, and it seems promising to show early stages of stress-induced bone remodeling. However, unlike scintigraphy, it is mainly limited to the lower part of the limbs. Ultrasonography, as a very accessible modality, can be a helpful tool. Cortical disruption, irregular bone contours, and hematoma formation are the common occurring signs (Fig. 45.12).
