Temporal maturation of the major white matter tracts follows a nonlinear pattern, with the most significant growth during the first year of life, followed by a slower pace in the second year of life.
Spatial maturation of the major white matter tracts follows a central-to-peripheral, caudal-to-rostral trend during early brain development.
Significant genetic effects on the development of both global and local white matter diffusion properties are present, whereas common environmental effects are restricted to specific tracts during the first 2 years of life.
Lateralization of white matter is observed in the fiber bundles associated with language and sensorimotor functions, whereas gender effects are subtle during infancy.
Significant diffusion–cognition correlations have been observed in infancy, and abnormal development of white matter has been associated with different brain diseases/disorders.
4.1 Introduction
An understanding of early brain development is of great clinical importance because many neurological and neurobehavioral disorders have their developmental origins during this period.1,2 Conventional T1-/T2-weighted magnetic resonance imaging (MRI) has been routinely used for the clinical assessment of brain maturation processes, including gray/white matter growth, water content changes, and myelination.3,4 Although anatomical/morphological developments are readily apparent in conventional MRI, insights into the development of white matter (WM), particularly the myelination status of WM with age, have been more challenging to obtain until the introduction of diffusion tensor imaging (DTI).5,6 The underlying physical mechanism of DTI is that the relative angle between the directions of the applied diffusion gradients and water diffusion determines the extent to which magnetic resonance signal intensity is altered in the presence of diffusion gradients; the maximum signal-intensity reduction occurs at a 0-degree angle (i.e., parallel), whereas, theoretically, no signal-intensity change is anticipated at 90 degrees (i.e., perpendicular). Therefore, applying diffusion gradients along noncollinear directions, a tensor matrix can be employed to characterize the directions of water diffusion. Subsequently, the three eigenvalues/eigenvectors obtained through matrix diagonalization of the tensor matrix can be used not only to measure rotation-invariant water diffusion properties, such as mean diffusivity and fractional anisotropy (FA), but also to provide the preferred direction of water diffusion in a given voxel.
Major WM growth occurs prenatally. After the completion of neuronal migration during the last trimester of gestation, interneuron connections are built at two ends: neurons develop dendritic trees to make synaptic connections with other neurons within the gray matter and extend long axons running through the WM to establish long-distance connections. Although almost all prominent WM tracts can be identified using DTI after term birth despite low anisotropy values,7 continued growth of WM tracts occur postnatally, including increases of axon diameter, improvements of tract organization, developments of miscellaneous axonal cell organs, and, most importantly, development of myelin sheaths for insulating major fiber bundles.8 Life span examinations of the WM growth consistently show nonlinear developmental patterns featuring the most dramatic development of all major diffusion properties during the first 2 years of life,9,10 a critical period for WM development. This chapter reviews the recent literature on the use of DTI to study WM development during the first 2 years of life. The chapter discusses the temporal/spatial pattern of WM maturation and the issues related to lateralization and gender effects. Recent evidence is reviewed on the genetic control of early brain WM maturation and its behavioral correlations. Finally, the chapter summarizes recent progress on the clinical findings of DTI in early brain development.
4.2 Temporal Growth Pattern of Major White Matter Tracts
Measurements of intracranial gray matter, and WM volumes have consistently shown nonlinear brain growth, featuring dramatic growth during the first year, followed by more refined and slow growth during the second year of life.3 Total brain volume increases 105% during the first but only 15% during the second year of life.3 These temporal brain volume increases have been attributed to reflect different structural events, including synaptogenesis,11,12 dendrite elaboration,13 myelination,14,15 and continued subplate developmental changes,16 which have all been documented to undergo the fastest development during the first year. With these microstructural developments, it is highly plausible that water diffusion will be modified owing to the alteration of the biological microenvironment (barriers) such that a similar temporal pattern could be observed with DTI. Indeed, regardless of different diffusion parameters, results reported in the literature have generally converged on a nonlinear developmental trend.9,17 Specifically, using a region of interest (ROI)-based approach, Gao et al18 examined the spatial temporal development of eight ROIs located at the genu/splenium/body of the corpus callosum (CC), the internal capsule, the cortical spinal tract, the optic radiation, and the frontal/posterior peripheral white matter regions. All eight ROIs exhibit highly significant increases of FA in neonates to 1-year-olds, followed by a modest increase during the second year of life (▶ Fig. 4.1). Although FA represents a highly informative metric capable of characterizing the degree of anisotropy of the underlying water diffusion within a given voxel, it does not separately evaluate water diffusion parallel or perpendicular to the long axis of axonal bundles. In contrast, axial diffusivity (AD) and radial diffusivity (RD) separately quantify water diffusivity parallel and perpendicular to the main axis of water diffusion, respectively, potentially offering additional insights into the microarchitecture of axonal bundles. Using these two metrics, Gao et al further demonstrated that, although a temporal developmental pattern similar to that seen with FA was observed for axial and radial diffusivities during the first 2 years of life (see ▶ Fig. 4.1), a more rapid reduction of radial diffusivity to 44% to 69% of that at birth is observed during the second year of life, whereas the axial diffusivity decreases to only ~ 75 to 95% of the neonatal value (▶ Fig. 4.2). These findings suggest a more rapid development of radial constraints (e.g., myelination) than the growth of axonal structures during the first year of life. Consistent with these DTI findings, Haynes et al8 conducted a histological study with growth-associated phosphoprotein (GAP-43) staining, a marker of axonal growth and elongation. They showed a high level of GAP43 staining from 24 to 64 postconceptional (PC) weeks, implying rapid axonal development spanned from pre- to postnatal periods. Subsequently, a slower, adult-like level of axonal development was observed beyond 17 postnatal months (1.5 postnatal year). In contrast, myelination begins from 54 PC weeks (2.5 postnatal months) and develops at a high speed until 72 to 92 PC weeks (6.5 postnatal months to 11.5 postnatal months), spanning approximately the entire first year after birth. Together, these results suggest that the onset of axonal growth is earlier (prenatal) and fastest during the first 5 postnatal months, whereas myelin maturation begins about 2.5 months postnatally and continues at a fast growth pace throughout the first year of life. Therefore, these histological results by Haynes et al8 suggest the potential physiological underpinnings of the observed relatively faster decrease of radial diffusivity than axial diffusivity by Gao et al15 because the maturation of myelin is likely the dominant process during this period of life.
Fig. 4.1 Statistical comparison results of the development of fractional anisotropy (FA), axial diffusivity (AD), and radial diffusivity (RD) across the first 2 years of life. Red/yellow/green regions of interest are shown for illustration of the relative significance in age-dependent development (red: p < 0.001; yellow: p < 0.05; green: p > 0.05). GCC, genu of the corpus callosum; SCC, splenium of the corpus callosum; BCC, body of the corpus callosum; IC, internal capsule; CST, cortical spinal tract; OR, optic radiation; FPW, frontal peripheral white matter; PPW, posterior peripheral white matter. (Reproduced from Gao et al, with permission,15 original Fig. 3.)
Fig. 4.2 Development pattern of axial diffusivity, radial diffusivity, and FA across the first 2 years of life. The experimentally measured Life values of the 1- and 2-year-old groups are normalized to those of the neonates. GCC, genu of the corpus callosum; SCC, splenium of the corpus callosum; BCC, body of the corpus callosum; IC, internal capsule; CST, cortical spinal tract; OR, optic radiation; FPW, frontal peripheral white matter; PPW, posterior peripheral white matter. (Reproduced from Gao et al, with permission,15 original Fig. 4.)
More information could be revealed by considering the relationship between axial/radial diffusivity and FA changes. For example, when examining FA alone, three of the eight ROIs evaluated by Gao et al showed no changes in FA (i.e., splenium of the corpus callosum, internal capsule, and frontal peripheral WM) implying nonsignificant growth during the second year of life. However, further inspection of the radial and axial diffusivity revealed completely different pictures (see ▶ Fig. 4.1). Specifically, consistent with the finding of FA, the splenium of the corpus callosum exhibited no changes in both axial and radial diffusivities. In contrast, significant changes of both axial and radial diffusivities were observed in frontal WM, indicating continuing myelination and axonal growth. However, the extent to which axial and radial diffusivities were altered appeared to cancel their contributions in the formulation of FA calculation, leading to a stable FA. Finally, a significant reduction of radial diffusivity in the internal capsule was observed, suggesting continuing myelination. Furthermore, although a significant elevation of FA was observed in the genu/splenium/body of the corpus callosum, the cortical spinal tract, and peripheral WM between 1 and 2 years of age, these regions exhibited significant changes only in radial and not in axial diffusivity, suggesting that their growth is dominated by continued myelination rather than axonal growth. Together, these results underscore the importance of combined examination of both FA and axial/radial diffusivity measures for a better depiction of the complex underlying microstructural changes during the first 2 years of life.
In contrast, to examine regional maturation patterns of WM, Geng et al19 exploited a tract-based approach where several major WM tracts were evaluated. The findings observed were similar to those reported using ROI-based approaches regarding the temporal developmental trend of WM fiber tracts during the first 2 years of life. Specifically, they examined the growth of FA, axial diffusivity (AD), and radial diffusivity (RD) for 10 major WM fiber tracts: commissural bundles of the genu, body, and splenium of the corpus callosum; projection fiber tracts of the bilateral anterior/posterior limb of the internal capsule, motor, and sensory tracts; and association tracts of the bilateral uncinate fasciculus tracts, inferior longitudinal fasciculus, and arcuate fasciculus tracts. All fiber tracts showed increasing FA (16.1–55%) and decreasing RD (24.4– 46.4%) and AD (13.3–28.2%) in the first 2 years of life (▶ Fig. 4.3), featuring faster development in the first year than the second year. Consistently, RD showed larger changes than AD, in line with the ROI-based findings.
Fig. 4.3 Scatter plots of average fractional anisotropy (FA), axial diffusivity (AD), and radial diffusivity (RD) along seven representative tracts versus postnatal age. Horizontal lines represent the overall mean of the average values in one age group. Different tracts are visualized in different colors. UF, uncinate fasciculus; ILF, inferior longitudinal fasciculus; ALIC, anterior limb of internal capsule; PLIC, posterior limb of internal capsule. (Reproduced from Geng et al., with permission19)
4.3 Spatial Growth Pattern of Major White Matter Tracts
As previously described, extensive myelination processes of major WM tracts occur during the first year of life are likely the dominant factor attributes to the observed changes of WM diffusivities during infancy. Spatial maturation of WM tracts has been documented following a central-to-peripheral and caudal-to-rostral pattern. Specifically, myelination increases from the splenium of the corpus callosum and optic radiation (at 3–4 months), the occipital and parietal lobes (at 4–6 months), and, finally, to the genu of the corpus callosum and frontal and temporal lobes (at 6–8 months).20 Consistent with histological studies, DTI findings also show a similar pattern. Zhai et al17 demonstrated that the central WM areas consistently exhibit higher FA and lower mean diffusivity (MD) values when compared to the peripheral WM regions in neonates. Gao et al15 further showed that the general maturation pattern begins centrally (genu/splenium/body of the corpus collosum), followed by the cortical spinal tract, internal capsule, optic radiation, and peripheral WM. Moreover, the occipital matures earlier than the frontal peripheral WM regions (▶ Fig. 4.4). Additionally, Chen et al21 inspected linear (Cl) and planar (Cp) anisotropy. The linear anisotropy is defined as the trace normalized difference between the primary and second eigenvalues, quantifying percentages of the shape of a diffusion tensor matrix attributable to a cylindrical object. In contrast, planar anisotropy quantifies percentages of the shape of a diffusion tensor matrix attributable to a planar object. Their results showed that the central WM had a significantly higher Cl and lower Cp when compared to the peripheral WM during infancy, suggesting that highly organized fiber bundles have already formed at birth in the central major WM regions. In addition, the observed rapid growth velocity of Cl during the first 2 years further enhances the cylindrical shapes of diffusion tensors in these major WM regions, likely driven by the rapid myelination process. Similar findings were observed using tract-based analysis19; callosal bundles show larger FA changing rates in central than in peripheral regions. Motor and sensory tracts show a higher rate of changes of FA and RD in anatomical locations close to the cortical regions when compared to those close to the cerebral peduncle. The splenium of the CC is one of the tracts that has the largest FA and smallest RD in the first year, and maintains this high level of maturation in the second year. The genu of the CC has a relatively small RD until the second year of life. Overall, DTI measures reveal a central-to-peripheral and caudal-to-rostral pattern. These findings have profound functional implications and are consistent with the notion that brain functional development starts from basic brain functions, such as motor, sensory, and visual functions and proceeds to higher-order brain function.22 Maturation of the primary sensory functions requires either rapid up-down information relaying through center projection fibers (e.g., the cortical spinal tract) or interhemispheric communication through midbrain callosal fibers, consistent with the early maturation of central WM. In contrast, higher-order brain functions likely require the maturation of peripheral and anterior WM tracts,22 which undergo continuing maturation through adolescence and young adult life.
Fig. 4.4 Spatial development pattern of axial diffusivity (upper left), radial diffusivity (upper right), and fractional anisotropy (bottom) across regions of interest during the first 2 years of life. GCC, genu of the corpus callosum; SCC, splenium of the corpus callosum; BCC, body of the corpus callosum; IC, internal capsule; CST, cortical spinal tract; OR, optic radiation; FPW, frontal peripheral white matter; PPW, posterior peripheral white matter. (Reproduced from Gao et al, 15 with permission, original Fig. 5.)
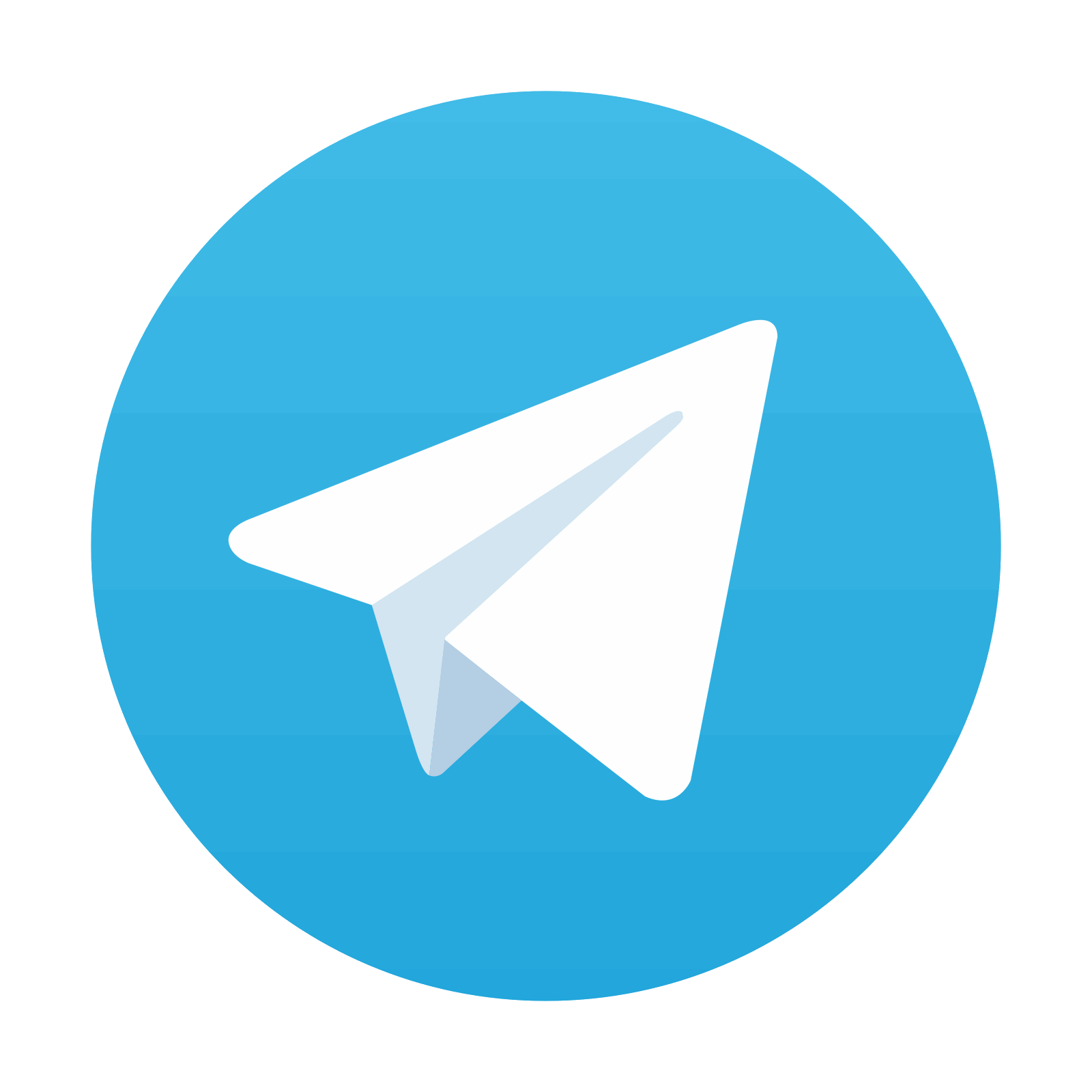
Stay updated, free articles. Join our Telegram channel

Full access? Get Clinical Tree
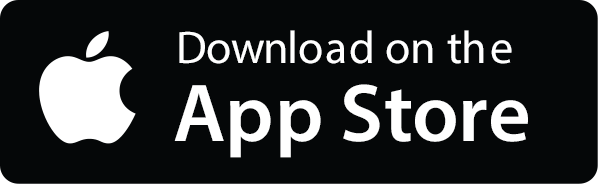
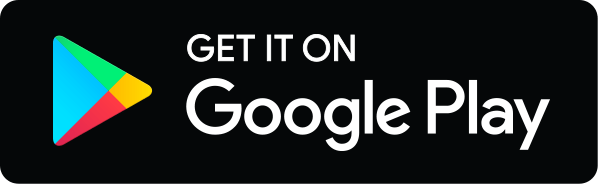