Diseases of the peripheral nervous system are the most common neurologic disorders. The basic diagnostic workup of neuromuscular diseases and peripheral neuropathies relies on the clinical examination supplemented by electrophysiologic techniques of electromyelography and neurography. The latter test the electrical conduction of peripheral nerves and the excitability of muscles as electrical epiphenomena of neuromuscular impulse conduction. Thus, imaging should be viewed as an adjunct to electrophysiology, not a replacement.
CT does not have a significant role in the diagnosis of neuromuscular diseases and peripheral neuropathies because of its poor soft-tissue contrast and concerns about radiation exposure. There are cases, however, in which the direct visualization of osseous structures by CT may be necessary due to the limited bone-imaging capabilities of MRI. It can help diagnose a nerve lesion, for example, in cases where posttraumatic deformity or degenerative joint changes are associated with nerve irritation (e.g., late ulnar paralysis), or fracture callus or bone fragments have incited a focal neuropathy.
For a discussion of neurological ultrasound, we refer the reader to the specialized literature. It is beyond the scope of this chapter, which deals primarily with MRI.
Magnetic resonance neurography (MRN), or neurological MRI using specially adapted sequences and coils, plays a central role in the imaging of peripheral nerves. With a sufficiently high field strength of 3 T, the peripheral nerves can be resolved down to the fascicular structural level. Specific nerve branches, such as the anterior intraosseous nerve or the deep branch of the radial nerve, can be traced to a point just before their entry into the target muscle. In this way MRN can supply high-resolution information on lesion location and on the number and distribution pattern of multiple nerve lesions. The differentiation of monofocal and multifocal lesions is particularly relevant because monofocal neuropathies often involve a compression neuropathy that is potentially treatable by surgery. Multifocal neuropathies, on the other hand, often have an underlying systemic cause.
Note
The reliable differentiation between monofocal and multifocal nerve lesions on the basis of electrophysiologic testing and clinical examination alone is often difficult. MRN can add crucial diagnostic information.
18.2 Basic Technical Principles of Magnetic Resonance Neurography
The technical requirements for MRN include the highest possible field strength (i.e., 3 T in clinical diagnostic use) and flexible, malleable multichannel coils that can cover large body surface areas. Another key requirement is the use of special pulse sequences that can shorten the examination time while maintaining a strong signal.
The most important criterion for MRN in clinical use is the detection of nerve signal changes in high-resolution, fat-suppressed T2w sequences (sample sequence protocol shown in ▶ Table 18.1). A normal nerve appears iso- to slightly hyperintense to surrounding muscle tissue in T2w images. Increased signal intensity in T2w images is the most important and sensitive criterion for lesion detection in MRN. This criterion is largely nonspecific for determining whether the underlying lesion is benign or malignant, but allows detection with very high spatial resolution and comprehensive anatomic coverage. For example, lesions of individual nerve fascicles can be localized with pinpoint accuracy in anatomically complex nerve plexuses and also in fine distal nerve branches. Even without specifying the histopathology of a nerve lesion, this localizing information often has major diagnostic and therapeutic implications, because the complete or at least representative depiction of spatial lesion patterns is fundamental to the classification of peripheral neuropathies. A typical clinical MRN examination using a 3-T scanner and suitable receiver coils can provide a spatial resolution as high as 100 μm × 100 μm (pixel size within a two-dimensional image of the nerve cross-section). The T2w signal from the nerve fascicles can be received and processed quantitatively (by T2 relaxometry) or qualitatively (T2w TSE technique).
Sequence | Repetition time TR (ms) | Echo time TE (ms) | Inversion time TI (ms) | Slice thickness (mm) | Pixel spacing (mm2) | Field of view (mm) | Number of slices per imaging volume | Number of averagings |
Coronal SPACE-STIR sequence | 3800 | 267 | 180 | 0.8 | 0.781 × 0.781 | 250 | 72 | 2 |
Sagittal oblique T2w SPAIR sequence | 5530 | 45 | 3.0 | 0.469 × 0.469 | 150 | 51 | 4 | |
Axial fat-saturated T2w sequence | 7020 | 52 | 3.0 | 0.300 × 0.300 | 130 | 45 | 3 | |
SPACE = Sampling Perfection with Application optimized Contrasts using different flip-angle Evolutions; |
Tips and Tricks
When interpreting T2w signal changes in a nerve, remember that a nerve running at an oblique angle to the main magnetic field (B0) may show an artifactual increase in signal intensity. This “magic angle effect” can be avoided by positioning the nerve segment so that it is oriented in line with the main field axis.
Additionally, an artifactual increase in the signal intensity of a nerve can be clearly differentiated both quantitatively and qualitatively from true changes in signal intensity.
T1w sequences before and after contrast administration are helpful in the diagnosis of neoplasms such as nerve sheath tumors and in postoperative settings (e.g., checking for incomplete decompression or recurrent tumor). Also, excellent survey images of the brachial plexus and lumbosacral plexus can be obtained with three-dimensional isotropic sequences (e.g., SPACE-STIR), i.e., sequences with exceptionally high spatial resolution that allow for artifact- and distortion-free reconstruction of the target structures in all spatial planes. The intradural course of nerve roots and their constituent fibers (intradural filaments) can be accurately defined with heavily T2-weighted sequences (e.g., CISS or SPACE). At the peripheral level, all structures of the supraclavicular, infraclavicular, and axillary brachial plexus can be imaged at high resolution with special multichannel surface coils. When this special setup is used to image the brachial plexus, all of its elements (spinal nerves, trunks, divisions, fascicles) and the nerves that arise from the plexus to supply the arm, including the axillary nerve, can be differentiated from one another. Besides these established morphologic sequences, other sequences for the acquisition of functional parameters in peripheral nerve tissue, such as diffusion tensor imaging (DTI), are still undergoing technical development and clinical validation.
18.3 Pathologic Conditions
18.3.1 Traumatic Neuropathies
In patients with peripheral nerve trauma, it is often difficult in early stages to determine whether the affected nerve has maintained its continuity or has been severed. This cannot be done confidently by clinical examination or electrophysiologic testing but has a major bearing on further management (spontaneous regeneration of a nerve with intact continuity versus nerve reconstruction if continuity has been lost). MRN is a reliable method for evaluating the continuity of peripheral nerves and neural plexuses. This applies not only to superficial nerves but also to more deeply situated structures. In many cases, therefore, MRN is a valuable adjunct to a previous sonographic or neurosonographic examination, as sonographic techniques are limited in their ability to evaluate nerve or plexus segments that are close to bone or deeply embedded in the soft-tissue envelope. In the case of traumatic lesions, MRN can distinguish among a true neuroma, an in-continuity neuroma, and a functional lesion with intact continuity and no neuromatous expansion.
We can illustrate this principle for the brachial plexus. Trauma may cause the avulsion of intradural filaments or may cause a complete peripheral transection followed by neuroma formation. In both cases MRN can supply an accurate diagnosis ( ▶ Fig. 18.1). If there are no contraindications to MRI, postmyelographic CT scanning of the intradural nerve roots is obsolete for reasons of radiation exposure.
Fig. 18.1 Intradural nerve root avulsions following a motor vehicle accident. The survey image is a coronal reformatted high-resolution CISS sequence. Complete intradural nerve root avulsions are visible at the C7 and C8 levels on the left side (arrows). The diagnosis can be established only by comparison with the healthy side and the direct detection of absent intradural filaments, not by the indirect “pseudomeningocele” sign.
Pitfall
Detecting a ▶ pseudomeningocele on MRN does not prove that all intradural filaments have been avulsed. Rather, the confident diagnosis of a nerve root avulsion requires direct visualization of the injury (see ▶ Fig. 18.1).
Imaging of the brachial plexus should be delayed until approximately 4 weeks after severe trauma so that plexus evaluation is not hampered by soft-tissue edema or hematoma. When there is a complete continuity disruption with neuroma formation, MRN has an essential role in selecting patients for early operative treatment ( ▶ Fig. 18.2). If an in-continuity neuroma is present, imaging cannot reliably predict whether or not spontaneous regeneration is likely to occur. If there is only a functional lesion with no significant change in caliber, characterized on MRN by T2w hyperintensity of the affected nerve segments as in a traction injury, it is reasonable to take an initial wait-and-see approach with regard to spontaneous regeneration.
Fig. 18.2 True avulsion injury of the supraclavicular brachial plexus (superior trunk). The solid arrow indicates the distal neuroma stump (the proximal stump has retracted outside the field of view). The dashed arrow indicates the intact distal plexus elements. The fact that the structures distal to a true neuroma are intact is relevant for surgical nerve repair, as it is a prerequisite for successful regeneration distal to the repair site.
18.3.2 Nerve Compression Syndromes
The first scientific studies on MRN dealt with carpal tunnel syndrome as the most common compression neuropathy. In this syndrome the nerve is flattened at the compression site and usually shows only slight hyperintensity in T2w sequences ( ▶ Fig. 18.3). In severe cases the nerve proximal and distal to the compression site typically shows marked T2w hyperintensity and swelling. MRN mainly has a clinical role in the less common, diagnostically challenging cases of carpal tunnel syndrome where there are possible associated nerve lesions (differentiating between multifocal neuropathy and polyneuropathy) or an unsatisfactory postoperative course.
Fig. 18.3 Typical appearance of carpal tunnel syndrome on MRN. The median nerve is markedly hyperintense at the compression site in the T2w image. This case features the normal variant of a bifid median nerve. The fascicles closest to the flexor retinaculum show the greatest signal increase in the T2w image (solid arrow). The fascicles that lie somewhat deeper in the palm, and thus farther from the retinaculum, show less hyperintensity (dashed arrow).
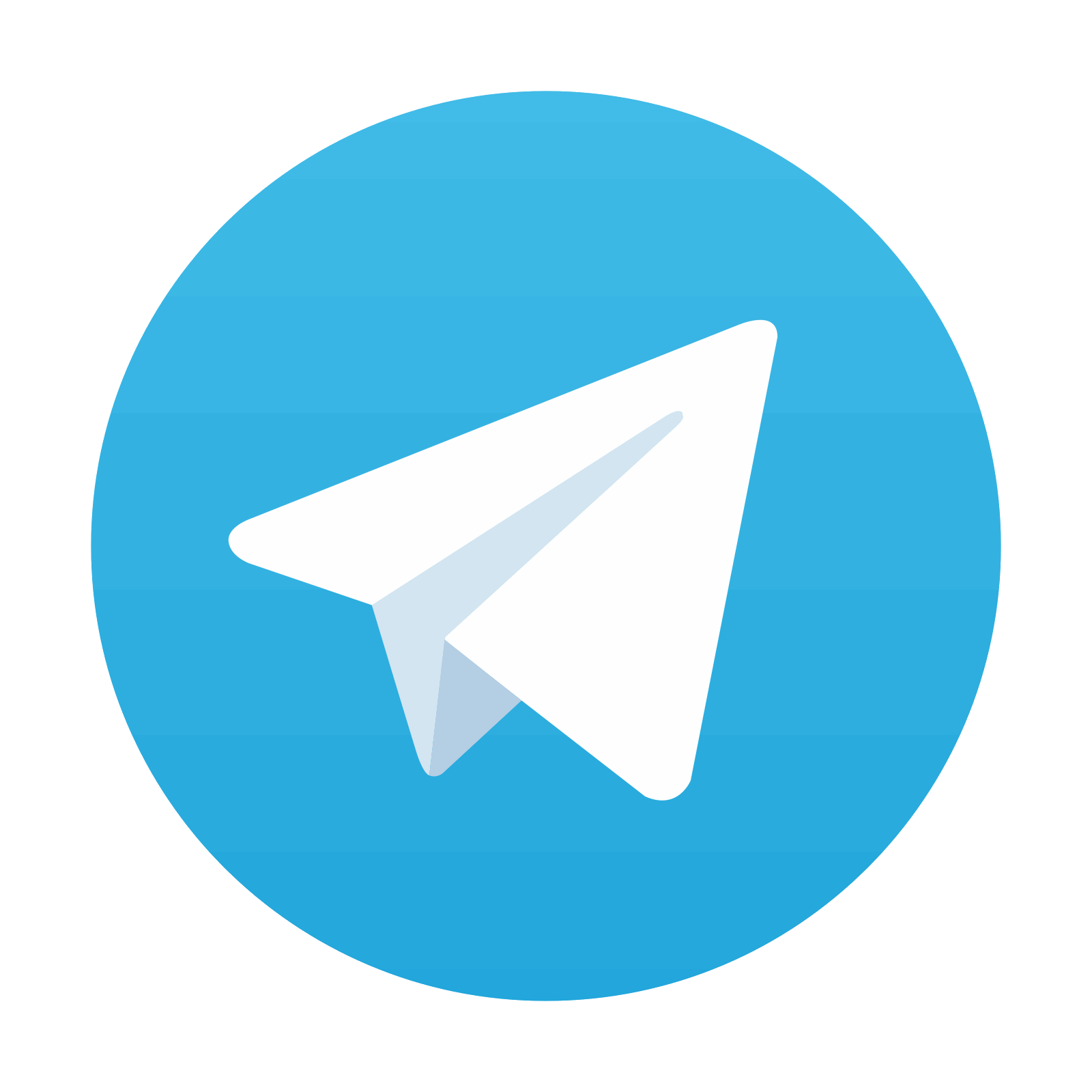
Stay updated, free articles. Join our Telegram channel

Full access? Get Clinical Tree
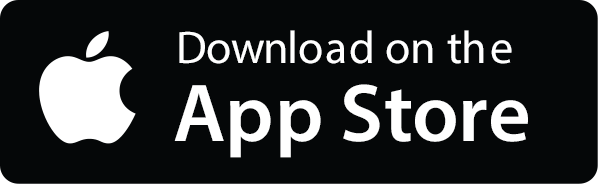
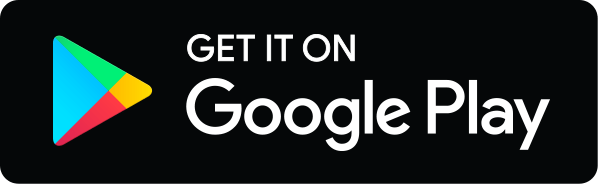