Vascular Diseases
2.1 Cerebral Ischemia
Stroke is caused either by cerebral ischemia (70–80% of all cases) or by an intracerebral or subarachnoid hemorrhage (20–30%). It has an acute to subacute onset and is associated with focal neurologic symptoms that depend on the affected brain area. Older synonyms include “cerebral insult” and “cerebrovascular accident.” But regardless of the term used, every stroke patient requires an imaging investigation, which may need to be done quickly depending on the age of the patient, the acuteness of the stroke, and locally available treatment options. Imaging is the only available tool that can quickly and reliably determine the pathology and often the pathogenesis of the clinical event.
Note
Every stroke patient requires CT or MRI.
2.1.1 Epidemiology
The incidence of first stroke is between 150 and 350:100,000 per year. The risk of recurrence after an initial stroke is 10 to 15% per year. Approximately 15% of patients who suffer an ischemic stroke die within the first 3 months, some from the immediate effects of the infarction and others from secondary complications such as pneumonia, pulmonary embolism, or a second stroke. With improvements in treatment strategies, today approximately 40% of patients survive their stroke with no significant residual disability. Patients with cardiogenic embolism have the poorest prognosis, while patients with lacunar infarcts have the best prognosis.
The risk profile for cerebral ischemia is fairly well known. The greatest risk factor is arterial hypertension. The incidence of ischemic strokes is closely correlated with the level of the blood pressure, and antihypertensive therapy can significantly reduce the risk of ischemic stroke in terms of both primary and secondary prevention. The risk of ischemic stroke rises with aging, especially in patients with atrial fibrillation, left-sided heart failure, or diabetes mellitus. Men are more commonly affected than women, although the sex difference declines with aging. Heavy smoking triples the risk of stroke. Alcohol abuse, obesity, and hyperlipidemia may also increase stroke risk, although this link has not always been statistically significant in large studies. The correlation between hypertension and stroke risk is strikingly illustrated by some numbers: The stroke risk doubles with each 7.5 mmHg rise in diastolic blood pressure, and lowering the systolic blood pressure by 9 mmHg or the diastolic pressure by 5 mmHg will reduce stroke risk by 30 to 40%. Smoking increases stroke risk by a factor of approximately 1.5 to 2, and the risk rises in proportion to nicotine use. Cessation of smoking will significantly reduce the stroke risk within 2 to 5 years, with some studies even indicating a return to nonsmoker levels.
2.1.2 Clinical Manifestations and Treatment
In many ischemic strokes, the clinical presentation will indicate the site of the infarction. As a general rule, strokes in the anterior circulation have a more uniform presentation while infarcts in the vertebrobasilar circulation are considerably more complex and clinically less specific. It is a troublesome fact for neuroradiologists that many (emergency) referrals for suspected stroke are in patients with atypical symptoms which, when seen in isolation, would generally not be characteristic of stroke. These include an isolated decrease in level of consciousness, headache (except with subarachnoid hemorrhage), syncopal episodes (more likely to have a cardiogenic or epileptic cause), psychomotor agitation, and confusion. Dizziness is also frequently interpreted as a stroke symptom. Thus, everything that referring physicians may classify as a suspected stroke is not urgently suspicious for one. It would be helpful if more or even all of these patients were referred to a neurologist.
A thorough clinical examination conducted in the acute phase of stroke can supply very reliable information on the location and extent of the damaged brain area. The more skilled and experienced the examiner, the better the chance of clinically distinguishing lacunar symptoms from territorial infarcts and thus determining the urgency of sectional and vascular imaging for these different subsets of patients.
Infarctions in the carotid territory account for approximately three-fourths of all cerebral infarctions; the territory of the middle cerebral artery (MCA) is most commonly affected. Classically, MCA infarctions present with contralateral brachiocephalic or predominantly distal hemiparesis and hemisensory deficits. If the internal capsule is included in the infarcted area, the hemiparesis almost always affects the lower extremity as well. With infarctions in the dominant hemisphere, the symptoms often include neuropsychological deficits (aphasia).
A complete MCA infarction may lead to significant swelling of the affected hemisphere in the first 2–5 days, initially causing decreased consciousness that progresses to coma and then to death from transtentorial herniation. Decompressive craniectomy may be a life-saving intervention, especially in patients with a right hemispheric infarction. The goal of radiology in these cases is to provide the earliest possible indication that a malignant MCA infarction will develop.
Isolated infarcts in the territory of the anterior cerebral artery (ACA) are extremely rare. With unfavorable vascular anatomy or a hypoplastic A1 segment, however, an MCA infarction may very well be combined with a bilateral ACA infarction (occlusion of the carotid T with an asymmetrical cerebral arterial circle [circle of Willis]). In the author’s experience, however, ACA infarctions are most often caused by vasospasms following a potentially undiagnosed subarachnoid hemorrhage. In any case, the author would generally recommend catheter-based angiography in these patients if an obvious embolic source is not found.
Note
Isolated infarcts in the ACA territory are very rare! There should be little hesitation in recommending diagnostic digital subtraction angiography (DSA) for these patients. In the author’s experience, vasospasms are the most frequent cause of ACA infarctions.
The posterior circulation supplies the brainstem, cerebellum, and in most cases the occipital lobe and medial portions of the temporal lobe. Depending on possible variants in the origin of the posterior cerebral artery (PCA), which arises directly from the internal carotid artery (ICA) in approximately 20% of cases, the supratentorial region supplied by the posterior circulation is highly variable. The location of brainstem infarcts is suggested by cranial nerve deficits, which give the neurologist an accurate picture of the size and craniocaudal extent of the infarcted area. For example, typical syndromes result from infarction of the posterolateral medulla oblongata (Wallenberg’s syndrome). Infarcts in the territory of the inferior cerebellar artery may cause a significant mass effect in the posterior cranial fossa, depending on the collateral supply and dominance of the occluded artery. This situation, like a malignant MCA infarction, would be an indication for surgical decompression of the posterior cranial fossa.
The clinical classification of ischemic stroke based on pathogenesis has become established at many centers. The causes of stroke fall into three broad categories: large-vessel disease, cardiogenic embolism, and small-vessel disease. Other causes are also recognized (e.g., vasculitis, coagulation disorder, underlying hematologic disease) and some cases have an indeterminate cause. Based on this classification, cardiogenic embolism is the leading cause of ischemic stroke, accounting for approximately 27% of cases, followed by small-vessel disease (21%) and large-vessel disease (13%). In over one-third of all patients (35%), however, a cause cannot be established. This percentage could probably be reduced if radiologists routinely used new technologies—magnetic resonance angiography (MRA) or computed tomographic angiography (CTA)—to image the aortic arch. Perhaps they could even add cardiac MRI, which may be superior to echocardiography for detecting thrombi at occult sites in the heart. Although this has not yet been proven scientifically, clinical experience lends very strong support to this recommendation. Imaging studies in patients with embolic stroke should routinely include visualization of the aortic arch and supra-aortic vessels. Currently, CTA may be superior to MRA because of its sensitivity to calcium.
Note
Stroke patients require imaging not only of the brain tissue but also of the supra-aortic vessels. In future it is likely that cardiac MRI will play a role in the investigation of presumed cardioembolic strokes.
To date, only thrombolytic therapy with recombinant tissue-type plasminogen activator (rt-PA, alteplase in a dose of 0.9 mg/kg body weight) has proven successful in the acute phase of stroke. Administered within 3 hours of stroke onset, it has been shown to reduce disability by approximately 30% compared with a placebo. In patients with basilar artery thrombosis, local thrombolysis can dramatically improve the catastrophic prognosis of this disease. Increasingly, mechanical recanalization procedures are being used even for occlusions in the anterior circulation. Contrary to long-established clinical practice, patients with an acute ischemic stroke should not be heparinized because the bleeding complications may outweigh the benefit gained from reducing ischemic injury. On the other hand, the early initiation of aspirin therapy at 150 to 300 mg/day leads to a slight reduction of mortality and risk of recurrent stroke.
The task of radiology is to determine the extent of the irreversibly infarcted brain area in the early phase of stroke and to define the location, and if possible the collateralization, of the vascular occlusion. Early imaging also serves an important triage function: A patient with a brainstem lacuna will not necessarily require treatment at a maximum-care (regional) hospital, whereas an MCA or cerebellar infarction with a potential mass effect should be managed at a maximum-care facility, preferably by an experienced stroke team with standby access to an intensive care unit.
2.1.3 Pathogenesis and Pathophysiology
A basic distinction is made between cerebral ischemia with a large-vessel (macroangiopathic) and small-vessel (microangiopathic) cause. The traditional classification of stroke by its time course as a transient ischemic attacks (TIA), prolonged reversible ischemic neurologic deficit (PRIND), or completed stroke is a clinical description that does not apply to diagnostic imaging. Thus, for example, MRI reveals morphologic lesions in approximately 30 to 50% of patients with TIAs, especially if the attack lasts longer than 30 minutes or higher brain functions are affected.
Note
Between 30 and 50% of TIAs are actually ischemic strokes with associated morphologic changes on MRI.
From a clinical standpoint, efforts are being made to establish a new and better terminology for the temporal evolution of ischemic stroke. It has been suggested that TIA be renamed “transient cerebral dysfunction” with symptoms lasting less than 1 hour and no detectable infarction, thereby distinguishing it from a frank brain infarction. It would probably be better to avoid the term “transient” altogether, especially since it implies that the fleeting nature of the symptoms lessens the urgency of diagnostic investigation, when really the opposite is true. Because there is no sustained neurologic deficit, one must act swiftly to locate the source of the typically embolic ischemia and take appropriate action (medical or surgical) to prevent a definitive brain infarction. Thus, the diagnostic workup of a TIA should proceed as urgently as for an acute stroke, and it should always include imaging of the supra-aortic vessels (by CTA or MRA). An MR examination or at least CT should be performed within 24 hours of symptom onset. Perhaps in the future the primary MRI of stroke patients will routinely include cardiac imaging while the patient is still undergoing head imaging.
2.1.3.1 Large-Vessel Disease
The principal risk factor for large-vessel disease is arterial hypertension. The risk increases linearly with the systolic and diastolic pressure levels and is increased even when readings are slightly elevated. Besides hypertension, cigarette smoking is another important risk factor for supra-aortic large-vessel disease. Other risk factors are diabetes mellitus, age, male gender, race, and genetic disposition. Possible or likely risk factors are oral contraceptive use, alcohol abuse, migraine headaches, and low socioeconomic status.
In simplified terms, large-vessel ischemia results from the occlusion of a large blood vessel supplying the brain. The pathophysiology may involve arterio-arterial embolism, cardiogenic embolism, local thrombosis, or hemodynamically significant stenoses in supply vessels. Most arterio-arterial emboli to the anterior circulation develop at the origin of the ICA, while most emboli to the posterior circulation originate in a proximal vertebral artery stenosis. Less common embolic sources are the ascending aorta and the intracranial segment of the ICA. A high-grade stenosis or occlusion of extra- or intracranial arteries may lead to cerebral ischemia if the blood pressure or blood volume is low and there is poor collateralization via the cerebral arterial circle (circle of Willis). The infarction in such cases is typically located in the watershed area between the PCA and MCA or between the MCA and ACA. On the whole, however, hemodynamic infarctions are somewhat rare. Cardiologists in particular often overestimate the hemodynamic significance of a carotid artery stenosis because they fail to consider the markedly different collateralization of the brain compared with the heart.
Pitfall
Hemodynamic strokes are rare. As a general rule, a proximal stenosis must be present in addition to a variant cerebral arterial circle (circle of Willis) before the cerebral hemodynamic reserve is compromised.
It is also rare for a cerebral supply artery to be occluded by local thrombosis. This phenomenon is probably most common in the basilar artery. In the author’s experience, some basilar artery occlusions are caused by the acute thrombosis of a high-grade stenosis. Incidentally, this mechanism should be considered in the thrombolysis of an acute basilar artery occlusion, as some patients will develop a recurrent occlusion following initial successful recanalization. The underlying pathology in such cases is very often a high-grade stenosis, and these cases should probably be managed by primary stenting to prevent reocclusion.
Cardiogenic emboli are the underlying cause in approximately 30% of all ischemic strokes. The main risk factor for cardiogenic embolism is atrial fibrillation. Even intermittent atrial fibrillation is associated with an increased stroke risk. Endocarditis is also associated with a high risk of cardiogenic emboli. In 50% of patients, cardiac emboli are ejected into the systemic circulation and usually pass into the brain. Congenital and acquired valvular heart disease, especially mitral valve defects, also increase the risk of cerebral embolism, especially if there is concomitant atrial fibrillation. The risk of cerebral embolism within 5 years after a myocardial infarction is almost 10%; it is highest in elderly patients and patients with an extensive infarction or severely decreased cardiac output. Cardiac tumors are extremely rare, with an incidence of 0.2%, but approximately 40% of these tumors shed emboli to the brain. The most common cardiac tumor is atrial myxoma.
Multiple TIAs or infarctions in various vascular territories of the brain (see ▶ Fig. 2.1) or mild changes in the extracranial arteries are suggestive of cardiogenic embolism.
Fig. 2.1 Infarctions in various vascular territories. (a) T2w image of a partial infarction of the left MCA. The gyral pattern is typical of an infarction and is clearly distinguishable from a tumor. (b) FLAIR image of an ACA and MCA infarction on the left side. (c) FLAIR image of a right PCA infarction. A small infarcted area is also visible in the left PCA territory. The cause is an embolism in the basilar artery. (d) Sagittal T2w image of an infarction of the posterior inferior cerebellar artery.
Fig. 2.1 Infarctions in various vascular territories. (continued) (e) FLAIR image of a hemodynamic infarction in the watershed area of the left ICA. The patient has a high-grade stenosis of the left ICA accompanied by a contralateral ICA occlusion. (f) Sagittal T2w image following occlusion of the basilar artery. The image reveals a large infarct in the upper pons, which has caused a locked-in syndrome.
2.1.3.2 Small-Vessel Disease
The main underlying pathology of small-vessel lesions in the brain is lipohyalinosis of the perforating arteries in a setting of arterial hypertension. Depending on their location, these changes may lead to lacunar infarcts in the basal ganglia or brainstem or to subcortical atherosclerotic encephalopathy with diffuse demyelination of the white matter. Cerebral small-vessel disease is distinguished from extracranial cerebrovascular occlusive disease, occlusive disease of the large intracranial arteries at the level of the brain surface, and occlusive disease of the major dural sinuses. As a general rule, cerebral small-vessel disease cannot be diagnosed angiographically due to the small size of the affected vessels but must be inferred from the effects on the brain parenchyma. The affected vessels are the long perforating arteries, which have a luminal diameter of 0.5 to 0.05 mm and are characterized by a marked discrepancy between the length of the arteries and their small caliber. They are end-arteries with essentially no capacity for collateral circulation. Ischemic lesions in these arteries are typically supratentorial with a bilateral and multifocal distribution.
Cortical involvement is likely to occur in patients with cerebral amyloid angiopathy, certain forms of vasculitis, MELAS syndrome (mitochondrial encephalopathy with lactic acidosis and stroke), and toxemic vasculopathy during pregnancy and lactation.
The pathogenesis of classic small-vessel disease in the brain is not precisely known. Several risk factors have been identified, however:
Advanced age.
Arterial hypertension.
Diabetes mellitus with hyperinsulinism.
Hyperhomocysteinemia.
The cortex, incidentally, is not affected by typical subcortical atherosclerotic encephalopathy because it is supplied directly by short perforating branches from the leptomeningeal network of superficial arteries.
Until recently it was believed that cerebral small-vessel disease was a more or less uniform entity, but there is growing evidence that it is a very heterogeneous group of diseases consisting basically of a hemorrhagic form and a purely ischemic form. The coming years will undoubtedly bring many new discoveries on this group of diseases.
Most patients show good clinical recovery from lacunar infarcts. Subcortical atherosclerotic encephalopathy is often marked by clinical deterioration and dementia over time. The main clinical symptoms of this disease are cognitive impairment, bladder dysfunction, and short shuffling steps, which are a frequent cause of falls in elderly patients.
Note
Small-vessel disease does not correlate with hypertension alone. The less common diseases should also be known and considered in the differential diagnosis.
If MR signs of small-vessel disease are found in the absence of hypertension, amyloid angiopathy should be considered in older patients, while cerebral autosomal dominant arteriopathy with subcortical infarcts and leukoencephalopathy (CADASIL) is a more likely diagnosis in younger patients. Typical lacunar symptoms are pure motor hemiparesis, pure sensory stroke, dysarthria–clumsy hand syndrome, ataxia and hemiparesis, and sensorimotor hemiparesis without neuropsychological deficits. When the presence of these symptoms is combined with normal CT scans or only a small lesion on MRI, there is probably no need to proceed with intra- and extracranial vascular imaging ( ▶ Fig. 2.2).
Fig. 2.2 Lacunes and subcortical atherosclerotic encephalopathy. (a) T2w image of a lacune in the left thalamus. (b) Diffusion image of the thalamic lacune in (a). (c) Typical pontine lacune in the left paramedian area. When this finding is accompanied by supratentorial small-vessel disease, no further imaging studies are required. (d) FLAIR image of massive subcortical atherosclerotic encephalopathy with confluent hyperintensities in the subcortical white matter on both sides.
2.1.3.3 Rare Causes of Stroke
Some rare causes of stroke are:
Inflammatory vascular disease.
Vascular dissection or trauma.
Medication and drug abuse.
Coagulation disorders.
For the treating physician, it is imperative for the radiologist to identify the pathogenesis whenever possible, not just to diagnose an “infarction.” This applies, incidentally, not just to patients with acute ischemia but also patients who are no longer in the acute phase when hospitalized. Diagnosed with a lacunar lesion, these patients may benefit from withholding further tests (e.g., cardiac studies) and proceeding with effective prophylaxis to prevent a second stroke. A swift, comprehensive workup can be particularly important in patients who have experienced a TIA.
Cerebral vasculitis may be part of a systemic vasculitis or may occur as an isolated condition (primary cerebral vasculitis). Besides cognitive impairment, frequent symptoms are headache, confusion, altered consciousness, and focal signs in the form of TIAs. Diagnosis based on MRI alone is not easy, but it is by no means certain that the gold standard of DSA will yield a definitive positive or negative result. If doubt exists and especially if an aggressive treatment is considered, brain biopsy and meningeal biopsy are still practiced even today. But vasculitis remains a difficult differential diagnosis, even in the age of high-tech imaging.
Migraine is an independent risk factor for strokes in women (usually before age 45). It is still uncertain whether the risk of stroke is increased in certain types of migraine (e.g., migraine with aura) and whether the stroke risk is increased in migraine patients over 45 years old, regardless of gender. In any case, when radiologists evaluate a stroke in a migraine patient, they should not automatically conclude a causal relationship and the workup should routinely include vascular imaging as it would in any other patient.
Note
The International Headache Society has developed the following criteria for migrainous stroke:
The patient has a migraine with aura.
The present attack corresponds to previous attacks but has an aura symptom lasting longer than 60 seconds.
Imaging studies show an ischemic infarct in a corresponding brain area.
The stroke cannot be attributed to any other cause.
The last point in particular implies that these patients should be examined as carefully for embolic sources as patients who do not have a migraine history. Migraine-related strokes most often occur in the posterior circulation, have a better prognosis, and generally occur in the absence of other risk factors.
Drug abuse is an increasingly common cause of stroke, especially in the U.S. Indeed, after cardiac causes, drug abuse is the second leading identifiable cause of stroke in persons under 35 years of age in the U.S. Thus, drug screening is an essential part of the diagnostic cascade in juvenile stroke patients. Drug-related strokes have been most closely linked to the abuse of cocaine, amphetamines (including ecstasy), opiates, hallucinogens such as lysergic acid diethylamide (LSD), and cannabinoids. The most commonly abused substance, cocaine, can induce stroke by a direct mechanism. Often the cause is vasculitis, which may lead to aneurysmal bleeding and hemorrhagic infarction or to local vascular occlusion. The recreational drug ecstasy can also cause vascular changes that may progress to an ischemic or hemorrhagic stroke. Again, the most likely causative mechanism in these cases is vasculitis, although hypertensive cerebral hemorrhage may occur in some patients.
Tips and Tricks
Drug screening should be routine in juvenile stroke patients.
2.1.4 MRI Findings
2.1.4.1 Large-Vessel Infarcts
The detection of infarcts on sectional images is a matter of pattern recognition. When the arterial distributions are known, infarcted brain areas can be assigned to specific vascular territories.
Large-vessel infarcts are most commonly located in the distribution of the MCA. Depending on the site of the vascular occlusion and the function of the leptomeningeal collaterals, the infarction may involve all of the MCA territory including the basal ganglia or it may be confined to one gyrus. Isolated basal ganglia infarcts are a special subtype of territorial MCA infarction. They result from occlusion of the M1 segment of the MCA accompanied by full leptomeningeal collateralization of the cortical branches ( ▶ Fig. 2.3). Because the lenticulostriate arteries are end-arteries, collateral flow is not delivered to the basal ganglion arteries when the M1 segment is occluded. Before this pathophysiology was known, these infarcts were often referred to as “giant lacunes.” While this is an apt descriptive term, it should be avoided because it suggests that the infarction is caused by small-vessel disease. “Embolic basal ganglia infarct” would be a more accurate term. Unlike the case of a lacunar infarct, a comprehensive search for an embolic source should be instituted in these patients.
Fig. 2.3 Partial infarction in the right anterior MCA territory. The affected area shows massive disruption of the blood–brain barrier 14 days after stroke onset. (a) Axial T2w image shows an anterior basal ganglia infarction on the right side. (b) Axial T1w image after contrast administration shows typical disruption of the blood–brain barrier after the ischemic infarction.
Note
Giant lacunes in the basal ganglia are embolic infarcts. The term “lacune” is misleading in this context and should not be used.
Infarcts in the territory of the ACA account for a relatively small percentage of all strokes (approximately 5%). Apparently this is because the ACA handles a considerably small blood volume than the MCA or PCA, and the anterior communicating artery can provide adequate collateral flow, at least in the case of proximal occlusions. The areas most commonly affected by an ACA infarction are the cingulate gyrus and frontobasal brain areas. The corpus callosum is rarely infarcted because it receives an excellent collateral supply from the posterior circulation. As noted earlier, vasospasm secondary to subarachnoid hemorrhage should be considered in patients with an isolated ACA infarction.
Infarcts in the territory of the PCA generally have an occipital location but may extend far into the temporomedial region. As a general rule of thumb, temporolateral infarcts are almost never caused by emboli in the posterior circulation. It is sometimes difficult to distinguish between a low-grade astrocytoma and an infarction, but a lesion that involves both the medial and lateral portions of the anterior temporal lobe is more likely to be a tumor. It is rare to encounter simultaneous embolic infarctions in different vascular territories.
Note
Lesions of the medial and lateral temporal lobe usually do not have a vascular cause.
Infratentorial territorial infarcts predominantly affect the cerebellum. The inferior surface of the cerebellum is supplied by the posterior inferior cerebellar artery, the middle cerebellar peduncle by the anterior inferior cerebellar artery, and the upper portions of the cerebellum by the superior cerebellar artery. It is noteworthy that the inferior cerebellar artery on one side may often supply small portions of the cerebellar undersurface on the contralateral side. With bilateral infarctions of the posterior inferior cerebellar artery, it is not strictly necessary to look for a cardiac source of embolism. Due to the partial bilaterality of the blood supply, an embolic source may conceivably be present in a vertebral artery.
Note
Bilateral infarcts in the territory of the posterior inferior cerebellar artery (usually asymmetrical in extent) almost always result from an embolic source in the aortic arch or dominant vertebral artery. A cardiac source is unlikely.
MRI is superior to CT for detecting hemorrhagic transformation of an infarcted zone, especially in embolic infarctions. The change is best appreciated on T1w images, which often show a narrow cortical zone of increased signal intensity. Similar to the pattern seen on CT, massive disruption of the blood–brain barrier is most apparent between days 12 and 21. This period is marked by scalloped rim enhancement of the infarcted brain area, which may even be mistaken for a tumor in rare cases. Contrast administration does not contribute to the imaging diagnosis of stroke per se, although it may be helpful in narrowing the differential diagnosis. So far there is no published evidence that the use of paramagnetic contrast medium has any effect on the prognosis of stroke patients.
2.1.4.2 Small-Vessel Infarcts
Given the very small size of the affected arteries, the diagnosis is not generally advanced by angiography (either classic catheter-based angiography or sectional modalities). Small-vessel disease is diagnosed from its effects on the brain parenchyma itself, i.e., lacunar infarcts (subcortical by definition) in the basal ganglia and brainstem and ischemic leukoencephalopathy (= subcortical atherosclerotic encephalopathy = leukoaraiosis). Mixed subcortical and cortical lesions are sometimes found. The affected arteries are end-arteries. Cortical involvement is seen in certain forms of vasculitis, in MELAS syndrome, and in toxemic vasculopathy during pregnancy and lactation—conditions that are also classified as small-vessel diseases.
Small-vessel disease can be diagnosed from morphologic MRI findings based on certain pattern of parenchymal brain lesions. The radiologist should understand, however, that this diagnosis always involves some degree of uncertainty, which can be resolved only by careful correlation with the clinical presentation. Otherwise there is a danger of misinterpreting very different diseases (e.g., cerebral storage diseases, multiple sclerosis, infectious diseases) as cerebral small-vessel disease.
On MRI, small-vessel disease is most easily recognized on T2w or fluid-attenuated inversion recovery (FLAIR) images. Lacunar lesions appear as focal hyperintensities, usually circular and measuring a few millimeters in diameter, located in the basal ganglia, thalamus, or brainstem. Subcortical atherosclerotic encephalopathy is characterized by symmetrical hyperintensities that start in the periventricular region and spread over time to involve all of the supratentorial white matter. The U-fibers (short association fibers) are almost always spared ( ▶ Fig. 2.4).
Fig. 2.4 Small-vessel infarcts. (a) Axial FLAIR image shows marked signs of subcortical atherosclerotic encephalopathy. (b) Image 2 years later shows definite progression of small-vessel disease. (c) Even more pronounced subcortical atherosclerotic encephalopathy with confluent white matter lesions sparing the subcortical U-fibers.
Lacunes require differentiation from ▶ Virchow–Robin spaces, which are perivascular spaces filled with cerebrospinal fluid (CSF). The Virchow–Robin spaces tend to enlarge with aging or long-standing hypertension but may occur at any age as normal variants without pathologic significance. Their high signal intensity makes them indistinguishable from lacunes on T2w images. In FLAIR images, however, they are isointense to CSF (i.e., dark) and cannot be confused with lacunes. While Virchow–Robin spaces are located predominantly in the deep basal ganglia (where the lenticulostriate arteries enter the brain), they are also found in the mesencephalon and subcortical white matter. Additionally, Virchow–Robin spaces never have a hemorrhagic component on T2*-weighted (T2*w) images ( ▶ Fig. 2.5).
Fig. 2.5 Virchow–Robin spaces. (a) Axial T2w image shows conspicuous Virchow–Robin spaces, which are even larger on the right side than on the left. (b) FLAIR image (same patient as in (a)). (c) Radial distribution of Virchow–Robin spaces in the white matter of a different patient. These prominent Virchow–Robin spaces are sometimes interpreted as signs of coexisting hypertension, but they are not (or not yet) classified as small-vessel disease. (d) Again, these lesions in a third patient should not be mistaken for lacunar infarcts. They are Virchow–Robin spaces in the substantia perforata of both cerebral crura.
T2*w or susceptibility-weighted imaging (SWI) should be performed in all patients with lacunar infarcts. These images will reveal microhemorrhages or hemorrhagic lacunes in 30–50% of these patients, who are at higher risk for cerebral hemorrhage and apparently higher risk for cognitive impairment. It is unclear at present whether these patients are more susceptible to cerebral hemorrhage in response to anticoagulant or antiplatelet therapy. In addition, this “microbleed microangiopathy” calls attention to the fact that cerebral small-vessel disease is much more clinically and radiologically diverse than was believed just a few years ago ( ▶ Fig. 2.6).
Fig. 2.6 Apparent typical small-vessel disease. (a) Axial FLAIR image of apparent typical small-vessel disease with white matter hyperintensity. (b) SWI in the same patient shows multiple subcortical microhemorrhages, which are most consistent with amyloid angiopathy.
Unlike multiple sclerosis, small-vessel disease almost never involves the corpus callosum, and this provides a simple differentiating criterion at imaging. Sagittal T2w sequences are helpful for this purpose.
Tips and Tricks
If spinal cord lesions are found in a patient with suspected subcortical atherosclerotic encephalopathy, it is reasonable to conclude that the patient has multiple sclerosis. Small-vessel disease never produces any (radiologically visible) spinal cord lesions ( ▶ Fig. 2.7).
Fig. 2.7 Dawson fingers in multiple sclerosis. (a) Axial FLAIR image. The fingerlike periventricular pattern is not characteristic of small-vessel disease but is consistent with the MRI appearance of multiple sclerosis. (b) Sagittal T2w image demonstrates the typical Dawson fingers even more clearly.
A frequently misidentified subform of cerebral small-vessel disease is CADASIL disease. First described by Tournier-Lasserve in 1991, this disease occurs throughout the world and to date has been diagnosed in 200 families in Germany. It is caused by a point mutation on chromosome 19. Its pathogenesis involves the deposition of granular eosinophilic material on the basement membrane of small vessels in various organs. T2w MR images demonstrate hyperintense white matter lesions that are also found at the temporal poles. The temporal lobe is almost never involved by hypertensive vascular leukoencephalopathy. Clinically, one-third of CADASIL patients have migraine, often with visual or sensory auras. Other clinical manifestations are ischemia, depression, dementia, and epileptic seizures. CADASIL patients are also considerably younger than patients with subcortical atherosclerotic encephalopathy. The diagnosis can be established by cutaneous biopsy, although CADASIL cases with a negative skin biopsy have been reported. A more accurate method is direct individual molecular genetic testing with detection of the NOTCH3 gene. Increased signal intensity in the temporal poles has a sensitivity of 90% and specificity of approximately 86%. Neuropathologically, the temporal pole changes represent a mixture of enlarged perivascular spaces and demyelination. Involvement of the subcortical U-fibers (especially in the frontal lobes and temporal poles) also appears to be highly specific in distinguishing CADASIL from degenerative small-vessel disease ( ▶ Fig. 2.8). MRI at higher field strengths can also demonstrate cortical infarcts in CADASIL. The MRI changes antedate clinical manifestations by up to 10 years. A good differentiating criterion from multiple sclerosis is involvement of the basal ganglia. A high lesion burden on MRI appears to signify more rapid progression of the disease.
Fig. 2.8 Typical MRI appearance of CADASIL. Temporal lobe involvement is virtually pathognomonic for this disease and distinguishes it from typical small-vessel disease. (a) As in hypertensive small-vessel disease, the white matter in both hemispheres is affected. (b) Involvement of the temporal lobe and especially the two temporal poles are typical of CADASIL disease and distinguish it from subcortical atherosclerotic encephalopathy.
Note
CADASIL that is suspected from radiologic findings can be confirmed by genetic analysis.
2.1.4.3 Cerebral Amyloid Angiopathy
This is an etiologically diverse group of vascular diseases characterized by congophilic deposits in the vessel wall on light microscopy. Cerebral amyloid angiopathy causes predominantly cerebral hemorrhages, especially in the form of recurrent lobar bleeds. Some cases also present with ischemic stroke and leukoencephalopathy. The disease is much more prevalent in older individuals than is generally believed; it is likely that 10% of persons over 75 years of age have significant amyloid angiopathy. Although amyloid angiopathy is basically a histologic diagnosis, imaging is helpful. T2*w images and SWI demonstrate small subcortical hemorrhages that are associated with few or no symptoms and can rarely be documented on standard T2w images. Also, the distribution pattern is unlike that of hemorrhagic lacunes, although there may be a degree of overlap between the two conditions. Mild trauma or neurosurgical procedures (caution: bleeding after stereotactic biopsy for tumor exclusion) may cause devastating cerebral hemorrhage ( ▶ Fig. 2.9).
Fig. 2.9 Cerebral amyloid angiopathy. (a) The FLAIR image suggests the presence of typical subcortical atherosclerotic encephalopathy. (b) SWI (b,c) demonstrates multiple microhemorrhages. (c) SWI in a different axial plane.
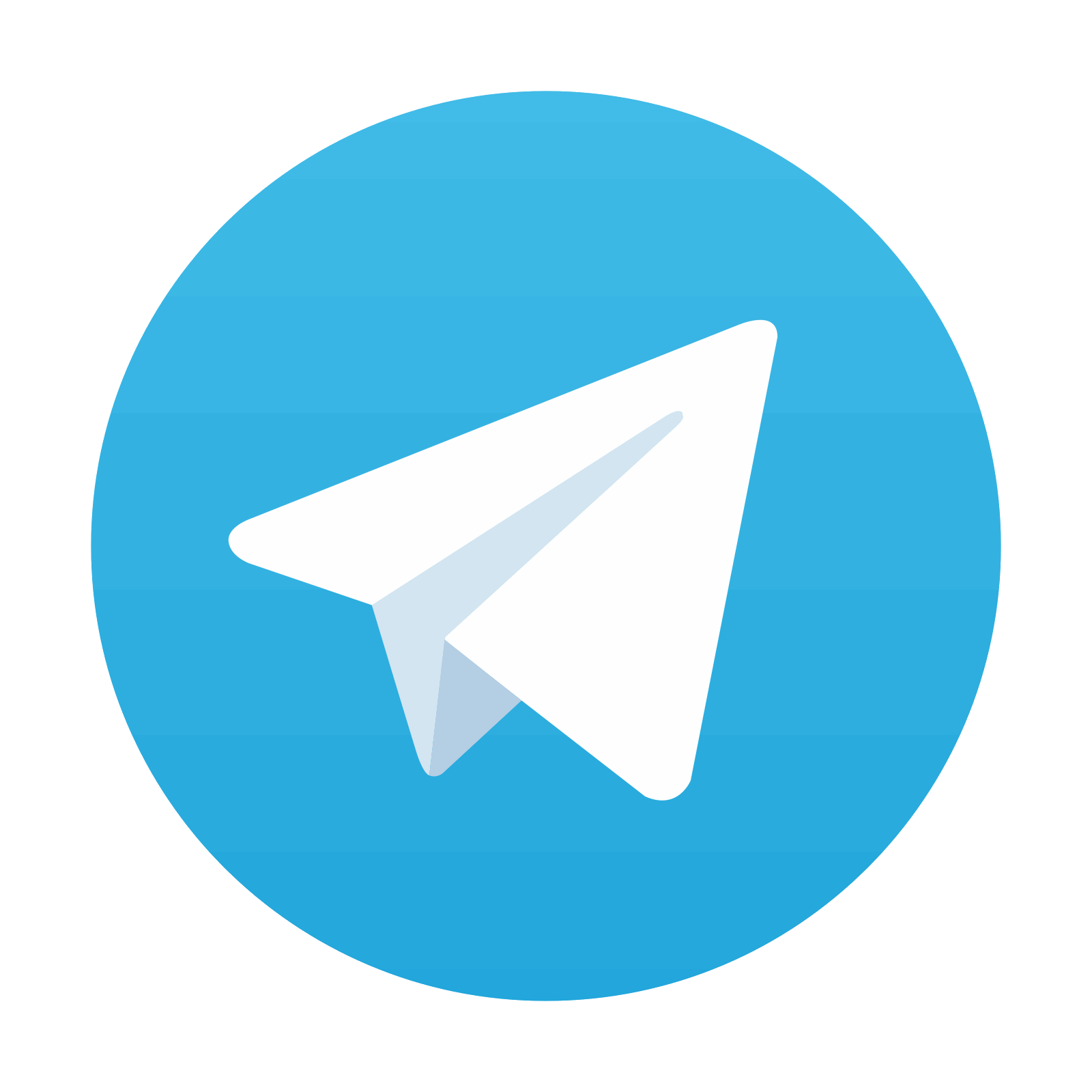
Stay updated, free articles. Join our Telegram channel

Full access? Get Clinical Tree
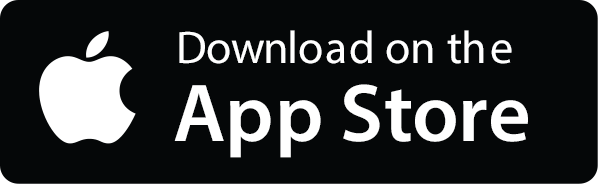
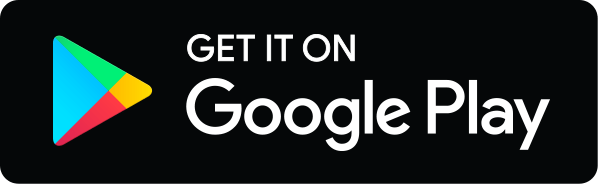