Chapter 10
Dynamic Imaging
Fluoroscopy
After completing this chapter, the reader will be able to perform the following:
1. Define all the key terms in this chapter.
2. State all the important relationships in this chapter.
3. Differentiate between fluoroscopic and radiographic imaging.
4. Recognize the unique features of an image-intensified fluoroscopic unit and explain how the image is created and viewed.
5. Explain the process of brightness gain and conversion factor during image intensification.
6. Define automatic brightness control and state its function.
7. Explain how using the magnification mode affects image quality and patient exposure.
8. Identify common types of image degradation resulting from image-intensified fluoroscopy.
9. Differentiate among the types of television cameras used to convert the output phosphor image for viewing on a television monitor.
10. List the types of recording devices available for image-intensified fluoroscopy.
11. Compare and contrast features of image-intensified units from digital fluoroscopic units.
12. State radiation safety procedures used to reduce exposure to the patient and personnel.
13. Recognize the need for quality control on fluoroscopic units.
The previous chapters discussed radiographic imaging to produce static radiographs of anatomic tissues. Imaging of the functioning or motion (dynamic) of anatomic structures is also needed for evaluation and is accomplished by fluoroscopy. For example, in order to visualize the stomach emptying its contents into the small bowel, images must be created in a continuous form for accurate evaluation of functioning. Most fluoroscopic procedures also require the use of contrast media to visualize internal structures and their functioning. This chapter discusses the components of fluoroscopic units, viewing and recording systems, and the digital fluoroscopy process in use today.
Fluoroscopy
Fluoroscopy allows imaging of the movement of internal structures. It differs from radiographic imaging by its use of a continuous beam of x-rays to create images of moving internal structures that can be viewed on a monitor. Internal structures, such as the vascular or gastrointestinal systems, can be visualized in their normal state of motion with the aid of special liquid or gas substances (contrast media) that are either injected or instilled.
In conventional fluoroscopy, the milliamperage (mA) used during imaging is considerably lower (0.5 to 5 mA) than radiographic mode, which is operated at a higher mA (100 to 1200 mA). A low mA provides for the increased time the fluoroscope is operated. Because the time of exposure is lengthened, the control panel includes a timer that buzzes audibly when 5 minutes of x-ray fluoroscopic time has been used. Another important feature of a fluoroscopic unit is the deadman switch. The continuous x-ray beam is activated by either a hand switch on the unit or a foot pedal that must be continuously depressed for the x-rays to be produced. Releasing the pressure applied to the pedal or switch terminates the radiation exposure.
Image Intensification
Image intensification (Figure 10-1) is the process in which the exit radiation from the anatomic area of interest interacts with the input phosphor (a light-emitting material, such as cesium iodide), for conversion to visible light. The light intensities are equal to the intensities of the exit radiation and are converted to electrons by a photocathode (photoemission). The electrons are focused by electrostatic focusing lenses and accelerated toward an anode to strike the output phosphor (coated with light-emitting crystals, such as zinc cadmium sulfide) and create a brighter image.
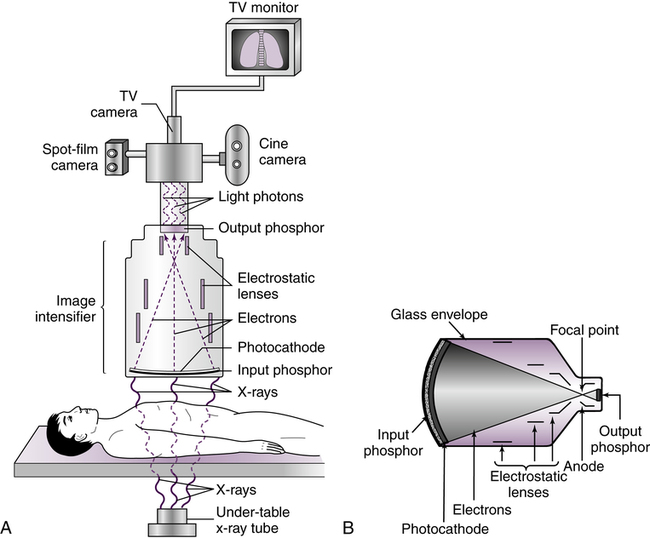
The image light intensities from the output phosphor are converted to an electronic video signal and sent to a television monitor for viewing. Figure 10-2 is an example of a typical radiographic and image-intensified fluoroscopic unit. Additional filming devices such as spot film or cine (movie film) can be attached to the fluoroscopic system to create permanent radiographic images of specific areas of interest.
Brightness Gain
A brighter image is a result of the high-energy electrons striking a small-output phosphor. Accelerating the electrons increases the light intensities at the output phosphor (flux gain). The reduction in size of the output phosphor image compared with the input phosphor image also increases the light intensities (minification gain). Brightness gain is the product of both flux gain and minification gain and results in a brighter image on the output phosphor.
Although the term brightness gain continues to be used, it is now common practice to express this increase in brightness with the term conversion factorConversion factor is an expression of the luminance at the output phosphor divided by the input exposure rate, and its unit of measure is the candela per square meter per milliroentgen per second (cd/m2/mR/s). The numeric conversion factor value is roughly equal to 1% of the brightness gain value. For example, a brightness gain of 20,000 would have a conversion factor of 200. The higher the conversion factor or brightness gain value, the greater the efficiency of the image intensifier. See Box 10-1 for brightness gain and conversion factor formulas.
Automatic Brightness Control
The radiographer must also be familiar with automatic brightness control (ABC), a function of the fluoroscopic unit that maintains the overall appearance of the fluoroscopic image (contrast and density) by automatically adjusting the kilovoltage peak (kVp), mA, or both. ABC generally operates by monitoring the current through the image intensifier or the output phosphor intensity and adjusting the exposure factor if the monitored value falls below preset levels. The fluoroscopic unit allows the operator to select a desired brightness level, and this level is subsequently maintained by ABC. ABC is a little slow in its response to changes in patient tissue thickness and tissue density as the fluoroscopy tower is moved about over the patient; this is visible to the radiographer as a lag in the image brightness on the monitor as the tower is moved.
Magnification Mode
Another function of some image intensifiers is multifield mode or magnification mode. Most image intensifiers in use today have this capability. When operated in magnification mode, the voltage to the electrostatic focusing lenses is increased. This increase tightens the diameter of the electron stream, and the focal point is shifted farther from the output phosphor (Figure 10-3). The effect is that only the electrons from the center area of the input phosphor interact with the output phosphor and contribute to the image, giving the appearance of magnification. For example, a 30/23/15–cm trifocus image intensifier can be operated in any of those three modes. When operated in the 23-cm mode, only the electrons from the center 23 cm of the input phosphor interact with the output phosphor; the electrons about the periphery miss and do not contribute to the image. The same is true of the 15-cm mode. Selecting magnification mode automatically adjusts x-ray beam collimation to match the displayed tissue image and avoids irradiating tissue that does not appear in the image. The degree of magnification (magnification factor [MF]) may be found by dividing the full-size input diameter by the selected input diameter. For example: MF = 30 ÷ 15 = 2× magnification.
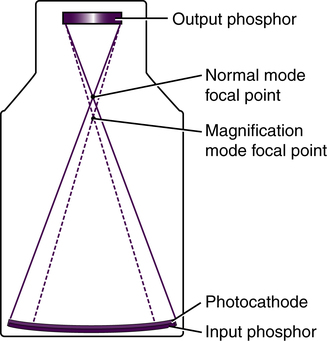
This magnification improves the operator’s ability to see small structures (spatial resolution, discussed shortly) but at the expense of increasing patient dose. Remnant x-ray photons are converted to light and then to electrons and are focused on the output phosphor. If fewer electrons are incident on the output phosphor, the output intensity decreases. To compensate, more x-ray photons are needed at the beginning of the process to produce more light, resulting in more electrons at the input end of the image intensifier. ABC automatically increases x-ray exposure to achieve this. Again, with an increase in x-rays used comes an increase in patient dose.
Magnification modes improve spatial resolution, which refers to the smallest structure that may be detected in an image. Spatial resolution is measured in line pairs per millimeter (Lp/mm), and typical fluoroscopic systems have spatial resolution capabilities of 4 to 6 Lp/mm but depend greatly on the rest of the imaging chain (i.e., the viewing and recording systems).
Distortion is also an issue with image-intensified fluoroscopy. In radiography, distortion is a misrepresentation of the true size or shape of an object. In the case of fluoroscopy, shape distortion can be a problem. In fluoroscopy, distortion is a result of inaccurate control or focusing of the electrons released at the periphery of the photocathode and the curved shape of the photocathode. The combined result is an unequal magnification (distortion) of the image, creating what is called a “pincushion appearance” (Figure 10-4). This problem also causes a loss of brightness around the periphery of the image, which is referred to as vignetting.
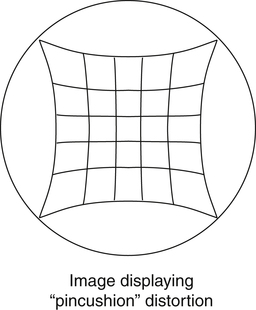
One last factor to consider with image intensifiers is noise. Image noise results when insufficient information is present to create the image. In the case of fluoroscopy, this lack of image-forming information ultimately goes back to an insufficient quantity of x-rays. If too few x-rays exit the patient and expose the input phosphor, not enough light is produced, which decreases the number of electrons released by the photocathode to interact with the output phosphor. A “grainy” or “noisy” image results (Figure 10-5). Although other factors in the fluoroscopic chain may contribute to noise, the solution generally comes back to increasing the mA (quantity of radiation). See Box 10-2 for common quality control tests specific to fluoroscopic equipment.