Chapter 2
The X-ray Beam
After completing this chapter, the reader will be able to perform the following:
1. Define all the key terms in this chapter.
2. State all the important relationships in this chapter.
3. Describe construction of the x-ray tube.
4. State the function of each component of the x-ray tube.
5. Describe how x-rays are produced.
6. Explain the role of the primary exposure factors in determining the quality and quantity of x-rays.
7. Explain the line focus principle.
8. State how the anode heel effect can be used in radiography.
9. Differentiate among the types of filtration and explain their purpose.
11. Recognize how changing generator output, kVp, mA, and filtration affect the x-ray emission spectrum.
12. List the guidelines followed to extend the life of an x-ray tube.
X-Ray Production
Anode
The anode of an x-ray tube is a positively charged electrode composed of molybdenum, copper, tungsten, and graphite. These materials are used for their thermal and electrical conductive properties. The anode consists of a target and, in rotating anode tubes, a stator and rotor. The target is a metal that abruptly decelerates and stops electrons in the tube current, allowing the production of x-rays. The target can be either rotating or stationary. Tubes with rotating targets are more common than tubes with stationary ones. Rotating anodes are manufactured to rotate at a set speed ranging from 3000 to 10,000 revolutions per minute (RPM). Figure 2-2 shows how a rotating anode and stationary anode differ in appearance.
The target of rotating anode tubes is made of a tungsten and rhenium alloy. This layer, or track, is embedded in a base of molybdenum and graphite (Figure 2-3). Tungsten generally makes up 90% of the composition of the rotating target, with rhenium making up the other 10%. The face of the anode is angled to help the x-ray photons exit the tube. Rotating targets generally have a target angle ranging from 5 to 20 degrees. Tungsten is used in both rotating and stationary targets because it has a high atomic number of 74 for efficient x-ray production and a high melting point of 3400° C (6152° F). Most of the energy produced by an x-ray tube is heat, so melting of the target can sometimes become a problem, especially with high exposures.
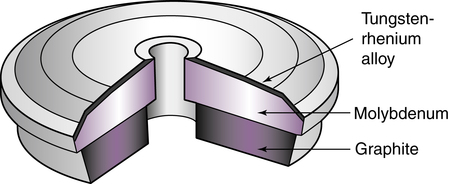
In order to turn the anode during x-ray production, a rotating anode tube requires a stator and rotor (Figure 2-4). The stator is an electric motor that turns the rotor at very high speed. The rotor (made of copper) is rigidly connected to the target through the anode stem (made of molybdenum), causing the target to rotate rapidly during x-ray production. High-strength ball bearings in the rotor allow it to rotate smoothly at high speeds.
Rotating anodes can withstand high heat loads. The ability to withstand high heat loads relates to the actual focal spot, which is the physical area of the target that is bombarded by electrons during x-ray production. With stationary targets, the focal spot is a fixed area on the surface of the target. With rotating targets, this area is represented by a focal track. Figure 2-5 shows the stationary anode’s focal spot and the rotating anode with its focal track. The size of the focal spot is not altered with a rotating anode, but the actual physical area of the target bombarded by electrons is constantly changing, causing a greater area—a focal track—to be exposed to electrons. Because of the larger area of the target being bombarded during an exposure, the rotating anode is able to withstand higher heat loads produced by greater exposure factors. Rotating anode x-ray tubes are used in all applications in radiography, whereas stationary anode tubes are limited to studies of small anatomic structures such as the teeth.
Target Interactions
Bremsstrahlung Interactions
Bremsstrahlung is a German word meaning “braking” or “slowing down radiation.” Bremsstrahlung interactions occur when a projectile electron completely avoids the orbital electrons of the tungsten atom and travels very close to its nucleus. The very strong electrostatic force of the nucleus causes the electron suddenly to “slow down.” As the electron loses energy, it suddenly changes its direction, and the energy loss then reappears as an x-ray photon (Figure 2-7).
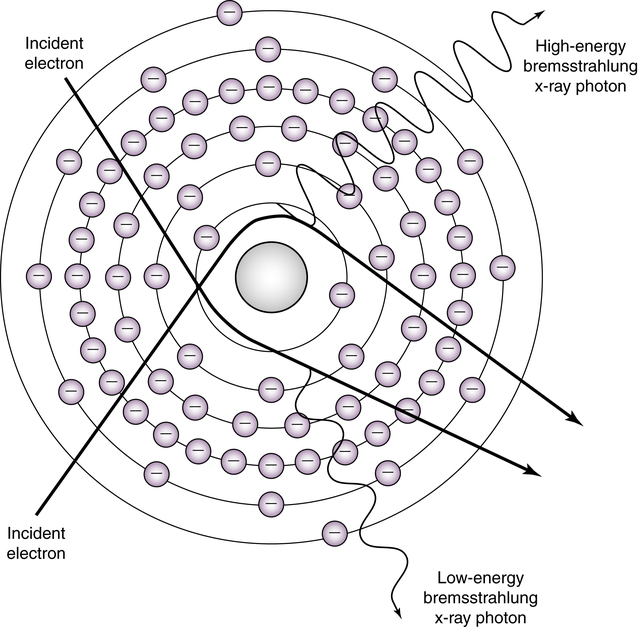
Characteristic Interactions
Characteristic interactions are produced when a projectile electron interacts with an electron from the inner shell (K-shell) of the tungsten atom. The electron must have enough energy to eject the K-shell electron from its orbit. K-shell electrons in tungsten have the strongest binding energy at 69.5 keV. For a projectile electron to remove this orbital electron, it must possess energy equal to or greater than 69.5 keV. When the K-shell electron is ejected from its orbit, an outer-shell electron drops into the open position and creates an energy difference. The energy difference is emitted as an x-ray photon (Figure 2-8). Electrons from the L-, M-, O-, and P-shells of the tungsten atom are also ejected from their orbits. However, the photons created from these interactions have very low energy and, depending on filtration, may not even reach the patient. K-shell characteristic x-rays have an average energy of approximately 69 keV; therefore, they contribute significantly to the useful x-ray beam. At less than 70 kVp (with a tungsten target), no characteristic x-rays are present in the beam. At greater than 70 kVp, approximately 15% of the beam consists of characteristic x-rays. X-rays produced through these interactions are termed characteristic x-rays because their energies are characteristic of the tungsten target element.
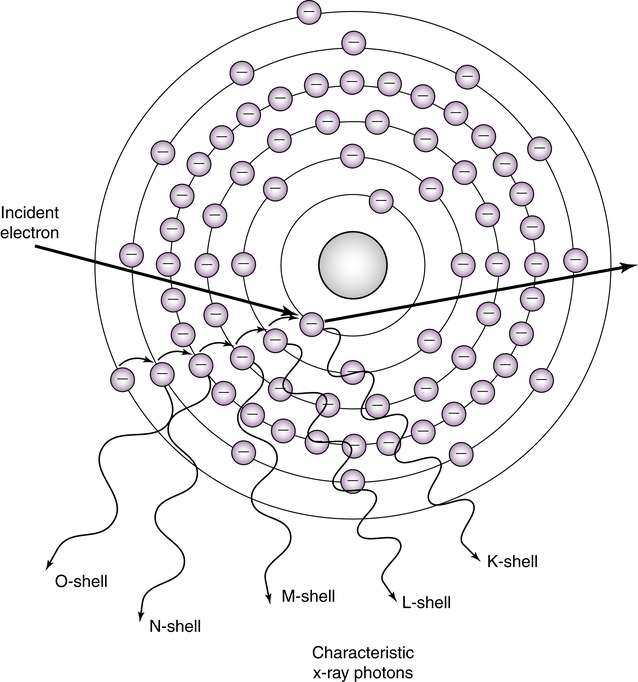
X-Ray Emission Spectrum
X-ray energy is measured in kiloelectron-volts (keV) (1000 electron volts). The x-ray beam is polyenergetic (many energies) and consists of a wide range of energies known as the x-ray emission spectrum. The lowest energies are always approximately 15 to 20 keV, and the highest energies are always equal to the kVp set on the control panel. For example, an 80-kVp x-ray exposure technique produces x-ray energies ranging from 15 to 80 keV (Figure 2-9). The smallest number of x-rays occurs at the extreme low and high ends of the spectrum. The greatest number of x-ray energies occurs between 30 keV and 40 keV for an 80-kVp exposure. The x-ray emission spectrum, or the range and intensity of x-rays emitted, changes with different exposure technique settings on the control panel.