Thyroid Disease—Radionuclide Diagnosis and Therapy
In 1941, the first patient was treated for thyroid cancer with radioiodine. Since then, radioiodine has proven invaluable in the assessment of thyroid disorders and treatment of thyroid cancer, Graves disease, and toxic thyroid nodules. Today, radioiodine I-123 and I-131 remain important diagnostic and therapeutic modalities, and the use of positron emission tomography (PET) iodine-124 is growing.
Thyroid Anatomy and Physiology
The thyroid gland lies in the anterior neck just inferior to the thyroid cartilage. It normally weighs 15 to 20 grams ( Fig. 8.1 ) and has two lobes connected by an isthmus that shows considerable anatomical variability. The pyramidal lobe, a remnant of the thyroglossal duct, is present in approximately two-thirds of patients, extending superiorly from the isthmus. Because of the thyroid’s embryological descent from pharyngeal pouches, ectopic tissue may be found anywhere from the foramen cecum at tongue base to the myocardium.
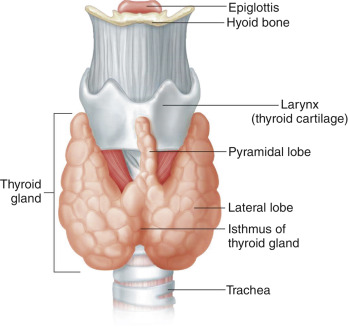
The gland is made up of follicles of varying size. The epithelial follicular cells at the periphery of the follicle synthesize and secrete thyroid hormone into the lumen, which contains colloid, where the hormone is stored ( Fig. 8.2 ). After oral ingestion, iodine is reduced to iodide (I-) in the proximal small intestine, where more than 90% is absorbed within 60 minutes. After distributing in the blood as an extracellular ion, it exits by thyroid and gastric extraction, then urinary and intestinal excretion.
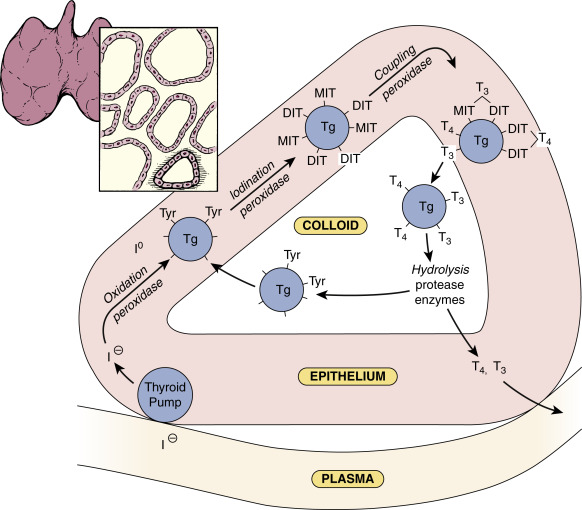
A high-energy sodium-symporter membrane traps iodide and concentrates it intracellularly 25 to 500 times greater than plasma. Thyroid peroxidase at the follicular cell–colloid interface promptly organifies it. The iodide then binds to tyrosine residues on thyroglobulin. These monoiodinated and diiodinated tyrosines couple to form triiodothyronine (T 3 ) and thyroxine (T 4 ), which are stored in the colloid-filled follicular lumen (see Fig. 8.2 ). Trapping and uptake can be blocked competitively by monovalent anions (e.g., potassium perchlorate), and organification can be inhibited by drugs used for Graves therapy, such as propylthiouracil (PTU) and methimazole.
Serum thyroid-stimulating hormone (TSH) initiates iodide uptake, organification, and thyroglobulin hydrolysis, releasing thyroid hormone into the bloodstream. Thyroglobulin itself is not released, except during disease states (e.g., thyroiditis or thyroid cancer). The major hormone released by the thyroid is T4, which is transported to peripheral tissues by thyroid-binding proteins and converted to the more metabolically active T 3 . Because the normal gland contains a 1-month supply of hormone, drugs that block hormone synthesis do not become fully effective in controlling thyrotoxicosis until intrathyroidal stores are depleted.
TSH secretion is primarily adjusted through the thyroid–pituitary feedback mechanism ( Fig. 8.3 ). When serum thyroid hormone levels are above normal, as in hyperthyroidism, serum TSH is suppressed, but when they are low, as in hypothyroidism, serum TSH serum levels increase.
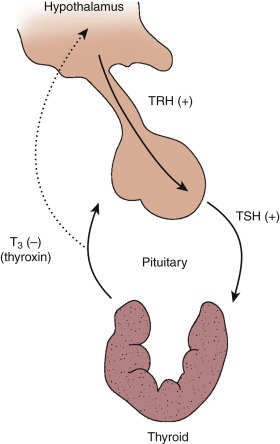
Radiopharmaceuticals
Radioiodine-131 (I-131) and -123 (I-123)
Like the stable iodine (I-127) normally encountered in the diet, radioactive iodine isotopes, such as I-131 or I-123, are selectively trapped by the thyroid and incorporated into thyroid hormone. Given this ability to localize, they are excellent tools for evaluating thyroid physiology, emitting gamma rays that provide clinically relevant qualitative and quantitative information.
Radioiodine doses are usually supplied as a capsule for oral administration. Although liquid I-131 may be available, it is volatile and increases the risk of exposing those around the patient. In the proximal small bowel, more than 90% of ingested iodine is absorbed rapidly. It is detectable in the thyroid within minutes of oral ingestion, reaching the thyroid follicular lumen by 20 to 30 minutes. Thyroid uptake normally continues to increase occurs over 24 hours ( Fig. 8.4 ), although imaging can be done as soon as 4 hours. The delay between radioiodine administration and imaging is not due to slow accumulation but rather the time needed to clear relatively high background activity. Radioiodine uptake can also be seen in the salivary glands, stomach, and choroid plexus; however, it is not concentrated or retained there. Excretion is via the kidneys and gastrointestinal tract.
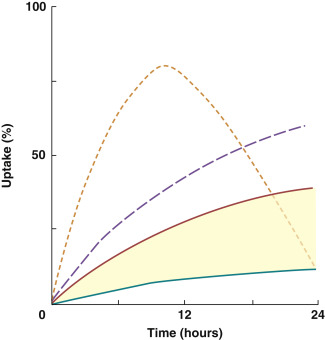
I-131 undergoes beta-minus decay (β–), emitting a principal primary gamma photon of 364 keV with an 8.1-day physical half-life ( Table 8.1 ). These photons are not ideal for gamma camera imaging. Count detection sensitivity is poor; half of the photons penetrate the collimator septa (even with a high-energy collimator) and the 3/8-in. sodium iodine camera crystal without detection, thus resulting in image degradation. High-energy β-particles (0.606 megaelectron volt [MeV]) are also emitted, which cannot be imaged but are valuable for therapy. The high-energy gamma, β-emissions, and long physical half-life result in a relatively high radiation dose to the thyroid (see the Appendix). Therefore, the dose for uptake is limited to 10 to 20 μCi. Even after thyroidectomy, doses are typically limited to 2 to 3 mCi (74 MBq) for thyroid cancer imaging because the β-emission could cause stunning in the residual cells, preventing uptake for effective therapy or future imaging. In the past, 30 to 50 μCi (1110–1850 MBq) of I-131 was sometimes used to determine whether an anterior mediastinal mass was due to a goiter. I-123 has replaced I-131 for this purpose.
Characteristics | Tc-99m | I-123 | I-131 | I-124 |
---|---|---|---|---|
Mode of decay | Isometric transition | Electron capture | Beta minus | Positron |
Physical half-life (T ½ ) | 6 hours | 13.2 hours | 8.1 days | 110 min |
Photon energy | 140 keV | 159 keV | 364 keV | 511 keV |
Abundance a | 89% | 83% | 81% | 100% |
Beta emissions | None | None | 606 keV | None |
a Abundance is the percent likelihood that a photon emission will occur with each radioactive decay.
I-123 decays by electron capture (13.2-hour half-life), and the principal emission is a 159-keV gamma photon (83.4% abundance), well suited for gamma camera imaging (see Table 8.1 ). There are some higher-energy emissions in low abundance, 440 to 625 keV (2.4%) and 625 to 784 (0.15%), making a medium-energy collimator preferable, although low-energy collimators are acceptable. Cyclotron production today results in I-123 that is 99.9% pure and no longer contaminated by long-lived isotopes (e.g., I-124, I-125). Given this and the lack of β-emissions, the radiation dose to the patient is roughly 100 times less than I-131 (10 rads/mCi) compared with 1000 rad/mCi. The standard uptake dose is 100 μCi (3.7 MBq) and 200 to 400 μCi (7.4 MBq) for routine thyroid scans. Higher doses can also be used in cancer imaging because I-123 does not cause stunning.
I-124 is a positron emitter that has been used as an alternative to I-123 or I-131 for thyroid cancer imaging, largely in an experimental capacity. It is cyclotron produced, decays by electron capture (75%) and positron decay (26%), and has a half-life of 4.18 days (see Table 8.1 ). Studies suggest it is superior to I-123 or I-131 for the detection of thyroid cancer metastases, and it has a potential role for thyroid cancer dosimetry before therapy. However, radiation dosimetry is relatively high (see Table 8.1 ).
Tc-99m pertechnetate is produced from a Mo-99/Tc99m generator, making it inexpensive and readily available, unlike the costlier I-123, which must often be ordered a day in advance. Because it is taken up by the same mechanism as iodine, Tc-99m pertechnetate can be used for thyroid assessment. The 140-keV photopeak (89% abundance) and lack of high-energy emissions are optimal for gamma camera imaging (see Table 8.1 ). In contrast to oral administration of radioiodine, Tc-99m pertechnetate is administered intravenously and rapidly taken up by the thyroid. However, it is not organified or retained in the thyroid, and imaging must be performed early at peak uptake time, 15 to 30 minutes after injection. The lack of particulate emissions and short 6-hour half-life result in a low radiation dose to the thyroid, far less than I-131 or I-123 (see the Appendix). Thus, the administered activity of Tc-99m pertechnetate can be much higher than that for I-123, usually 3 to 5 mCi (111–185 MBq), and the large photon flux results in high-quality images.
Special Considerations and Precautions
I-123 is the agent of choice for most adult thyroid imaging. Tc-99m pertechnetate may be preferred in children because of its lower radiation dosimetry and high count rate. However, because it is not organified, it is not recommended for nodule evaluation, and the rarely attempted Tc-99m pertechnetate uptake calculation is more difficult and less reliable.
For thyroid cancer imaging, the long half-life of I-131 is an important advantage over Tc-99m pertechnetate. Diagnostic I-131 scans are routinely acquired 48 hours after administration and 7 days posttherapy. The result is a high target-to-background ratio due to the time allowed for background clearance, resulting in good detectability of thyroid cancer metastases. Even for this diagnostic indication, I-123 is replacing I-131 because it permits earlier patient imaging at 24 hours, better image quality, and similar accuracy, and I-123 does not cause thyroid cell “stunning,” a situation where the cells are damaged by the emitted β, preventing future radioiodine uptake for imaging or therapy. These same high-energy I-131 β-emissions result in effective therapy not only for thyroid cancer but also for Graves disease and toxic nodules.
Uptake Inhibitors
A patient history, including food, drug, and prior imaging, should be obtained before thyroid uptake and imaging studies or radioiodine therapy. Thyroid replacement medications prevent imaging radiotracer uptake, as will thyroid-blocking medications. Exogenous iodine suppresses uptake and may preclude successful imaging or accurate uptake measurements. As little as 1 mg of stable iodine can cause a marked reduction in uptake; 10 mg can effectively block the gland.
Iodinated oral and intravenous radiographic contrast is a common source of iodine that interferes with thyroid studies. Given the high amount of iodine present in intravenous contrast, even water soluble, radionuclide diagnostic and therapeutic studies should be delayed for approximately 4 weeks following contrast administration.
The iodine normally found in foods and medications can also interfere with radionuclide thyroid studies ( Table 8.2 ). Greater amounts of iodine in the normal diet over the years in the form of iodized salt has resulted in lower normal values for the percent radioiodine uptake (%RAIU). Chronic renal failure impairs iodide clearance, expands the iodide pool, and lowers the %RAIU. Hypothyroidism reduces the glomerular filtration rate and slows urinary clearance of radioiodine from the body; hyperthyroidism increases the clearance rate.
Decrease Uptake | Duration of Effect |
---|---|
Thyroid hormones | |
|
|
| 2 weeks |
Excess iodine (expanded iodine pool) | |
| 2–4 weeks |
| 2–4 weeks |
| 2–4 weeks |
| Months |
| 2–4 weeks |
Congestive heart failure | |
Renal failure | |
| |
|
|
| Months to years |
| |
|
|
|
|
|
|
| |
|
|
| 5–7 days |
| |
| |
| |
Increase uptake | |
| |
| |
| |
| |
|
Iodine Allergy
The amount of iodine in a radioactive uptake or even a therapy dose is subpharmacologic and has not been associated with allergic reactions, even in patients with a documented iodine allergy.
Pregnancy and Lactation
The fetal thyroid begins to concentrate radioiodine by 10 to 12 weeks of gestation. Thus, significant exposure of the fetal thyroid can occur after therapeutic doses given to the mother, resulting in fetal hypothyroidism. A serum pregnancy test is mandatory before treating a female patient with I-131. Radioiodine is excreted in human breast milk. Because of the long half-life of I-131, nursing should be discontinued after diagnostic or therapeutic studies and not resumed. Breastfeeding may resume 48 hours after administration of I-123 and 24 hours after Tc-99m pertechnetate. According to the Nuclear Regulatory Commission (NRC), patients receiving I-131 for therapy or imaging should receive written as well as verbal radiation safety instructions if they are breastfeeding in addition to the routine instructions and normally required written directives.
Thyroid Uptake (%RAIU)
Thyroid uptake measurements for benign disease are acquired using a nonimaging gamma scintillation probe detector ( Figs. 8.5 and 8.6 ). The %RAIU can be determined with either I-131 or I-123 ( Box 8.1 ). Clinical indications are limited ( Table 8.3 ). It is most commonly used in the calculation of an I-131 therapy dose or to diagnose the cause of thyrotoxicosis, most commonly to differentiate Graves disease from subacute thyroiditis. Thyroid uptake values in other diseases are listed in Box 8.2 . The %RAIU is increased in Graves but suppressed in subacute thyroiditis.
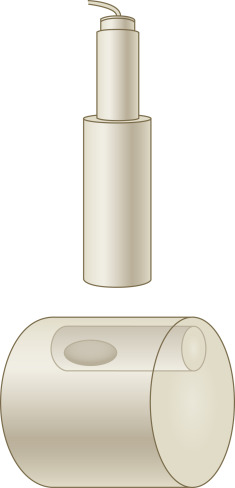
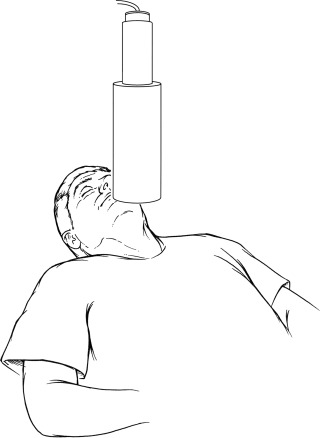
Percent Radioiodine Uptake (%RAIU)
- 1.
Preliminary measurements
Place dose capsule in neck phantom and count for 1 minute.
Count patient’s neck and thigh (background) for 1 minute.
- 2.
Administer oral dose capsule.
- 3.
Uptake measurement at 4 to 6 hours and 24 hours:
Count patient’s neck for 1 minute.
Count patient’s thigh for 1 minute.
- 4.
Calculation
%RAIU=Neck(backgroundcorrected)counts/minDosecapsule(decaycorrectedbackgroundcorrected)counts/min×100
Tc-99m Pertechnetate %Uptake
Before and after injection, the syringe is imaged to obtain counts (preinjection counts – postinjection counts = administered counts).
Scan regions of interest (ROIs) are drawn for thyroid, thyroid background.
Areas of interest are normalized for pixel size, and thyroid and syringe counts are normalized for time of acquisition.
Normal values are 0.3% to 4.5%.
Thyroid Scan | %Thyroid uptake |
---|---|
Functional status (cold, hot) of thyroid nodule | Differential diagnosis of thyrotoxicosis |
Detection of ectopic thyroid tissue (e.g., lingual thyroid) | Calculate Graves I-131 therapy dose |
Differential diagnosis of mediastinal masses (substernal goiter) with I-123 | Whole-body thyroid cancer scans pretherapy, posttherapy, and follow-up—to quantify residual or recurrent disease |
Thyroid cancer whole-body scan:
|
Increased Uptake
Graves disease
Toxic nodular goiter
Hashitoxicosis
Hydatidiform mole, trophoblastic tumors, choriocarcinoma
Metastatic thyroid cancer
Decreased Uptake
Subacute thyroiditis
Granulomatous thyroiditis (de Quervain)
Silent thyroiditis
Postpartum thyroiditis
Iodine-induced thyrotoxicosis (Jod–Basedow)
Amiodarone-induced thyrotoxicosis
Thyrotoxicosis factitia
Struma ovarii (decreased in thyroid, increased in ovarian tumor)
Medications that interfere with the radioiodine uptake should be discontinued before the study, with the length of time based on their half-lives (see Table 8.2 ). Patients should have nothing by mouth for 4 hours before radioiodine ingestion to ensure good absorption. I-123 and I-131 are usually administered in capsule form.
If a scan is not needed, 5 to 10 μCi I-131 or 50 μCi I-123 is adequate for a %RAIU because of the gamma probe’s high detection sensitivity. If a scan is ordered, Tc-99m pertechnetate can be utilized following the I-131 uptake. Both the scan and uptake can be performed with the I-123 scan dose (200–400 μCi). The %RAIU is best acquired at 4 and 24 hours after ingestion, although some acquire it at only one time period. The 24-hour uptake is the standard for I-131 therapy dose calculation.
Methodology for %RAIU
The nonimaging gamma scintillation probe detector used for thyroid uptake studies has a 2-cm-thick by 2-cm-diameter sodium iodine crystal with an open, single-hole lead collimator coupled to a photomultiplier tube and electronics. Room background activity is determined. The radioiodine capsule with known calibrated activity is placed in a Lucite neck phantom (see Fig. 8.5 ). Counts are obtained with the detector placed at a standardized distance of 30 cm. The radioiodine dose is then administered to the patient. At 4 and/or 24 hours, the probe is placed 30 cm from the anterior surface of the patient’s neck (see Fig. 8.6 ). Counts are obtained for 1 minute. The patient’s thigh is counted for a similar time to correct for background. The %RAIU is calculated according to the following formula:
%RAIU=Neckcounts/min∗Administreddosecapsulecounts/min∗correctedfordecayandbackground×100
The normal range for the %RAIU is approximately 4% to 15% at 4 to 6 hours and 10% to 30% at 24 hours. The early uptake informs that the %RAIU is elevated or suppressed. Some centers extrapolate from 4-hour uptake to estimate the 24-hour uptake for I-131 therapy dose planning. A problem with this approach is that some hyperthyroid patients have rapid thyroid iodine turnover. These patients may show an elevated 4- to 6-hour %RAIU but a lower value at 24 hours, thus underestimating the therapy dose needed (see Fig. 8.4 ). Patients with very high 4-hour uptakes can be brought back at 24 hours to ensure correct dosing, but having both time points is always optimal. The significance of uptake values must be determined by considering the entire clinical picture. Causes for various uptake values are outlined in Table 8.4 .
%Radioiodine Thyroid Uptake | |||
---|---|---|---|
Thyroid Function | Increased | Normal | Decreased |
Thyrotoxicosis | Graves disease Hashitoxicosis | Antithyroid drugs Propylthiouracil Methimazole | Contrast, high iodine exposure Subacute thyroiditis, thyrotoxic phase Thyrotoxicosis factitia Antithyroid drugs Struma ovarii |
Euthyroid | Rebound after antithyroid drug withdrawal Recovery from subacute thyroiditis Compensated dyshormonogenesis | Decompensated dyshormonogenesis | |
Hypothyroid | Decompensated dyshormonogenesis Hashimoto disease | Hashimoto disease After I-131 therapy Subacute thyroiditis, recovery phase decompensated dyshormonogenesis | Hypothyroidism: primary or secondary |
A Tc-99m pertechnetate percentage uptake is not commonly performed. Advantages are that peak uptake occurs at 20 to 30 minutes compared with 4 and 24 hours for radioiodine. Disadvantages are a considerably lower accuracy than %RAIU, often a lack of commercial software available for calculation, and a 24-hour uptake is not possible because it is not organified. The methodology is described in Box 8.1 .
Thyroid Scan
Iodine-123 is administered orally, and the scan is acquired 4 hours later. Imaging at 24 hours is possible; however, the low count rate at that time requires longer acquisition, increasing the likelihood of patient motion and image degradation. Four-hour images have superior image quality. Tc-99m pertechnetate is administered intravenously, and scan acquisition begins 20 minutes later. Detailed protocols are described for both in Box 8.3 .
Patient Preparation
Discontinue medications that interfere with thyroid uptake (see Table 8.2 ).
Nothing by mouth for 4 hours before study.
Radiopharmaceutical
Iodine I-123, 200 to 400 μCi (3.7–14.8 MBq), orally in capsule form (or)
Tc-99m pertechnetate, 3 to 5 mCi (111–185 MBq), intravenously
Time of Imaging
Iodine I-123, 4 hours after oral dose administration
Tc-99m pertechnetate, 20 minutes after injection
Imaging Procedure
Gamma camera with pinhole collimator
Energy window:
Tc-99m pertechnetate: 15% to 20% energy window centered at 140 keV
I-123: 20% window centered at 159 keV
Position the supine patient with the chin up and neck extended.
Acquire initial anterior view for 100,000 counts or 5 minutes with collimator placed to include right side and suprasternal notch markers.
Place the collimator closer so that the thyroid fills about approximately two-thirds of the field of view.
Acquire anterior, left anterior oblique, and right anterior oblique images for equal time compared with anterior view.
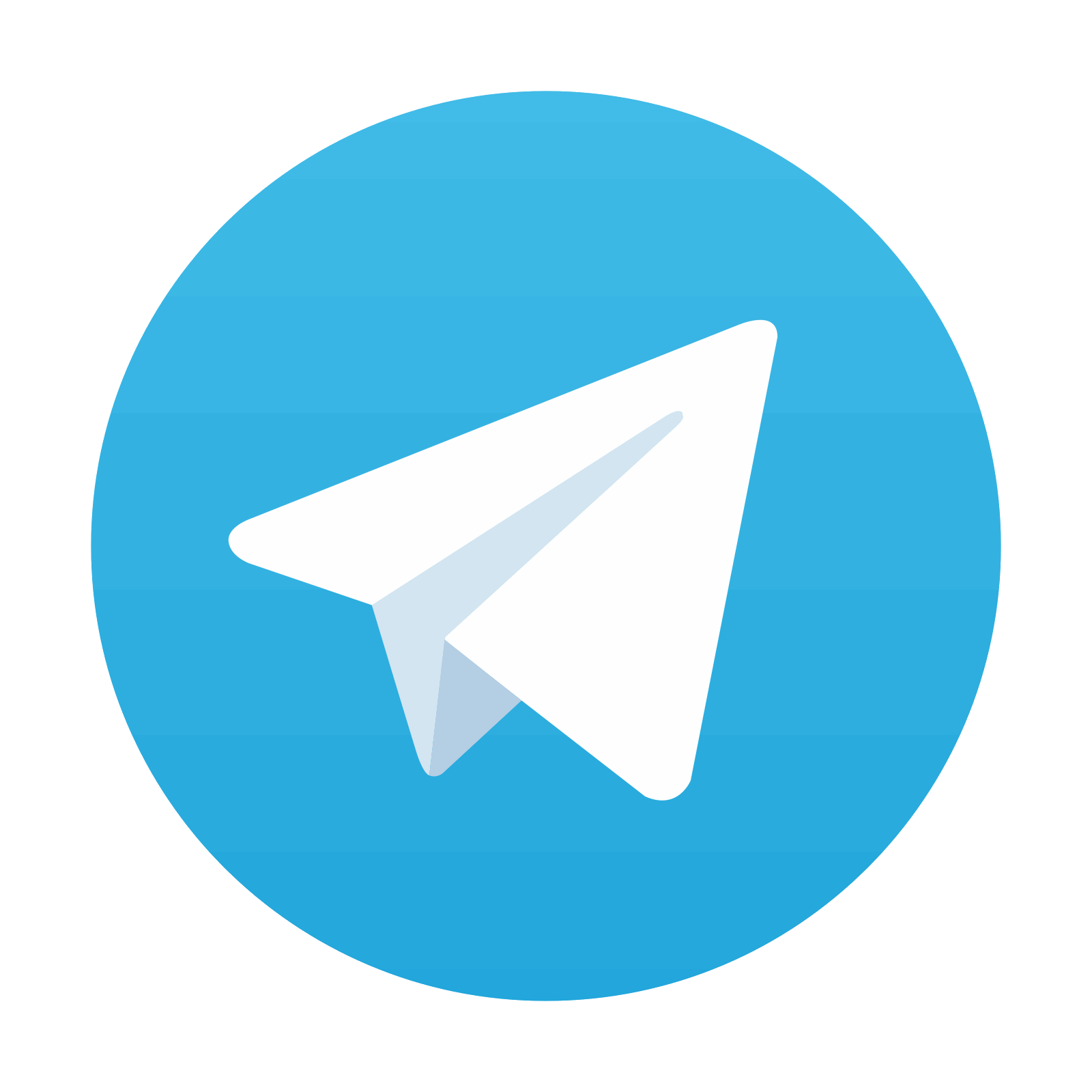
Stay updated, free articles. Join our Telegram channel

Full access? Get Clinical Tree
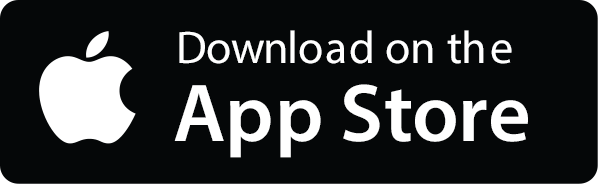
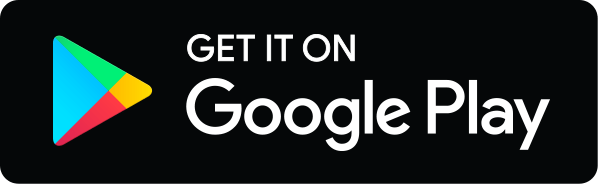
