First generation
Second generation
Third generation
Carbamazepine
Felbamatea
Clobazam
Phenytoinb,c
Gabapentin
Ezogabinea
Phenobarbitalb
Lamotrigine
Lacosamideb,c
Primidone
Levetiracetamb,c
Perampanel
Valproateb,c
Oxcarbazepine
Pregabalinb
Tiagabine
Rufinamide
Topiramate
Vigabatrina
Zonisamide
Treatment selection of an AED should include considerations regarding efficacy and tolerability as well as side effect profile, pharmacokinetics, and the need for rapid introduction. Although there are comparative trials [12] between the first-generation AEDs that have shown phenytoin and carbamazepine to be superior (combined efficacy and tolerability) to the other older agents, no such comparative trials between newer AEDs have been performed that have demonstrated superiority between the newer agents or between the newer and older agents. There is a general trend away from initial therapy with sedating AEDs (e.g., phenobarbital, primidone) and AEDs that have powerful hepatic enzyme induction (e.g., phenytoin, phenobarbital, carbamazepine) that may result in drug–drug interactions or promote bone mineral density loss. Agents with nonlinear kinetics (e.g., phenytoin) can be more likely to produce toxicity at higher doses and serum levels. Most of the newer second- and third-generation AEDs require much less monitoring of hematologic and metabolic parameters, and titration of these medications can be done based on clinical parameters (i.e., seizure control and lack of dose-related side effects) without a need for frequent monitoring of serum levels of these AEDs.
When a patient has experienced a disabling seizure (i.e., with alteration of consciousness), it is desirable to institute antiepileptic therapy promptly. As mentioned above, in these patients with symptomatic epilepsy, it is not necessary to wait for a second seizure; this is likely to occur without AED therapy. The limits on the ability to introduce an AED rapidly are governed by a number of factors including sedative or cognitive side effects (e.g., phenobarbital, topiramate), the risk of hypersensitivity reactions (e.g., lamotrigine), or pharmacokinetic considerations. It takes five drug half-lives to reach a steady state; an AED with a long half-life like phenobarbital or perampanel (~100 h t 1/2) can take weeks to reach a steady state. Conversely AEDs with short half-lives such as levetiracetam or lacosamide (7–8 h t 1/2) reach steady state levels about 35 h after starting a maintenance dose.
The past popularity of phenytoin was in large part due to the ability to load this agent, either orally or parenterally, providing early effective therapy. To a large degree, levetiracetam has replaced phenytoin for acute therapy. Levetiracetam has a much more favorable pharmacokinetic and side effect profile compared to phenytoin, with no enzyme induction, no drug–drug interactions, few hypersensitivity reactions, linear kinetics, and no significant sedative side effects. There is good level A evidence to support the use of levetiracetam as monotherapy for partial seizures [11]. Available in both oral and parenteral formulations, oral loading with levetiracetam achieves a cMax in 45 min [13] and is well tolerated [14]. The generic formulation of the immediate release formulation has excellent bioavailability compared with the proprietary compound [15]. Table 60.1 lists other AEDs that can be introduced relatively rapidly and are available in parenteral formulations. Lacosamide, a new third-generation AED, can also be introduced rapidly in many patients, and a parenteral formulation is available, although rapid loading is not quite as well tolerated as levetiracetam. Lacosamide has no significant hepatic induction and few drug interactions. In patients requiring two AEDs, the combination of levetiracetam and lacosamide is particularly well tolerated. Gabapentin and pregabalin have identical mechanisms of action; pregabalin is preferred because of its linear kinetics which increases CNS bioavailability at higher doses. Pregabalin is very safe and has no drug interactions. At high doses, particularly if introduced rapidly, some nonspecific CNS side effects can occur with pregabalin; often these are initiation side effects and abate with time.
Carbamazepine, an excellent AED for partial onset seizures, produces autoinduction, requiring slow introduction over several weeks to avoid toxicity. Carbamazepine also is a powerful hepatic enzyme inducer with multiple drug interactions and the potential for rare but serious blood dyscrasias. The congener oxcarbazepine has much less hepatic induction and no epoxide metabolite, allowing somewhat more rapid introduction than carbamazepine, although tolerability is enhanced with slow titration. Hyponatremia, much like SIADH, can occur particularly in the elderly and can be significant (i.e., serum sodium levels below 125 mEq) [16]. The acute use of lamotrigine, an otherwise well-tolerated agent with little sedation and no hepatic induction, is limited by the need for slow introduction over weeks to months to minimize the risk of hypersensitivity reactions which can on occasion be severe (e.g., Stevens–Johnson syndrome). With appropriate slow introduction, the risk of such severe rashes is no greater than phenytoin or carbamazepine [17]. Topiramate and zonisamide need to be introduced over several weeks because of the potential for cognitive side effects, particularly with topiramate.
In women of childbearing age, levetiracetam and lamotrigine have good profiles regarding associated major fetal malformations and development. Other AEDs can also be used in this patient population, but phenobarbital and valproate should be avoided unless absolutely necessary.
Acute/Subacute Effects of Radiosurgery on Epileptic Seizures
Treatment with radiosurgery is designed to produce focal injury to the targeted lesion. In the weeks and months after stereotactic radiosurgery, while the maximal effects and benefits of treatment are being accrued, there is local tissue reaction which can include edema and other local tissue effects [18, 19], typically with onset after 3–5 months. Patients with preexisting seizures due to the underlying lesion may have transient worsening of seizures requiring adjustment of AED therapy. Series studying complications of radiosurgery typically include heterogenous patient populations of patients with and without seizure histories and with lesions unlikely to produce seizures (e.g., acoustic neuromas, schwannomas) making assessment of these studies difficult. Patients without a history of previous seizures may develop seizures after radiotherapy of potentially epileptogenic lesions. In one report [20], 21 % of patients undergoing radiosurgery had seizures attributed to the radiosurgery treatment, with 15 % of patients having seizures in the early months after therapy. Interestingly four of six (67 %) patients with treated lesions near the motor cortex experienced seizures, suggesting that lesion location might be predictive of post-radiosurgery seizures. This is a reasonable hypothesis since even untreated lesions located in the frontal and temporal regions, involving neocortical or mesial temporal structures, may have a higher incidence of epileptogenicity.
In patients with preexisting seizure disorders, AEDs should be maintained during the acute and subacute radiotherapy period, with adjustments being made as needed based on seizure recurrence. In patients with new onset seizures shortly after radiotherapy, AEDs should be introduced employing the considerations for selection discussed above. The seizures in some patients may be more difficult to control during this subacute time period after radiotherapy when local tissue reactions are most prominent. As the local reaction subsides, seizures typically are much easier to control or manage. There is no data to support prophylactic treatment with AEDs in patients undergoing radiosurgery who do not have a seizure history; there are no good controlled studies of AED prophylaxis in patients undergoing radiosurgical therapy.
Treatment Implications After Successful Radiosurgery
The obvious major benefit of stereotactic radiosurgery is the ability to avoid a craniotomy and resective surgery. Successful treatment will slow or stop tumor growth or obliterate arteriovenous malformations, eliminating the risk of serious intracranial hemorrhage. But obviously the original lesion, although significantly altered anatomically and pathologically, still remains. What happens to previously symptomatic seizures in these patients? While radiosurgery has been successfully used to treat patients with drug-resistant partial epilepsy due to mesial temporal sclerosis [21, 22], the selection of radiosurgery for treatment of intracranial lesions is typically done independent of considerations of seizure control, specifically to reduce or eliminate risk of hemorrhage from a vascular malformation or to stop tumor growth. Indeed many patients may have not have had seizures or have had seizures that were readily controlled and not drug-resistant prior to radiosurgery. In all these patients, the decision to treat a lesion with radiosurgery is independent of seizure control but is based on these other important considerations.
The success of resective surgical therapy for drug-resistant lesional epilepsy is best with total resection of the epileptogenic lesion. Residual hemosiderin after cavernoma resection, or residual tumor, even if benign and nonprogressive (e.g., DNET, ganglioglioma) can compromise total seizure control or at least limit the ability to discontinue AEDs. It would be reasonable, therefore, to infer that since the underlying lesion is not removed with radiosurgery, the lesion including the associated hemosiderin, although altered, is still potentially epileptogenic. But there is reasonable evidence that radiosurgery may also reduce or eliminate the associated epileptogenicity of the lesion much like total resection. In the trials of treatment of drug-resistant seizures in patients with mesial temporal sclerosis (MTS), the percentage of patient achieving seizure freedom was comparable to results with standard open anterior temporal lobectomy with 77 % of patients in a recent small randomized trial achieving seizure freedom [21] with the higher regimen. As expected, the maximal beneficial effects of radiosurgery are not achieved until >1 year after treatment. The mechanisms of this beneficial effect of radiosurgery are not known but may include neuromodulatory effects or ischemic necrosis of epileptogenic tissue [22]. There are no comparative studies of AED discontinuation in patients successfully treated with anterior temporal lobectomy and stereotactic radiosurgery, but the goal of any surgical procedure for drug-resistant epilepsy, resective or radiosurgical, is to provide seizure control in these patients with previously uncontrolled partial epilepsy. Therefore radiosurgery should best be thought of as adjunctive therapy with AEDs for these patients.
One indication for open resection of a favorably located cavernous malformation is drug-resistant epilepsy, and radiosurgery offers an alternative for lesions in critical locations. A recent report [23] of 49 patients with cavernomas in or near eloquent cortex and drug-resistant epilepsy treated with radiosurgery found 53 % seizure-free with another 20 % achieving a significant reduction, and only 26 % showing little or no improvement. These patients often remained on some medical therapy for their seizures. While these numbers for seizure control are less than would be expected with open resective surgery [24], radiosurgery of cavernomas and arteriovenous malformations in patients with drug-resistant therapy remains an option in patients where open resection poses unacceptable risks [25, 26].
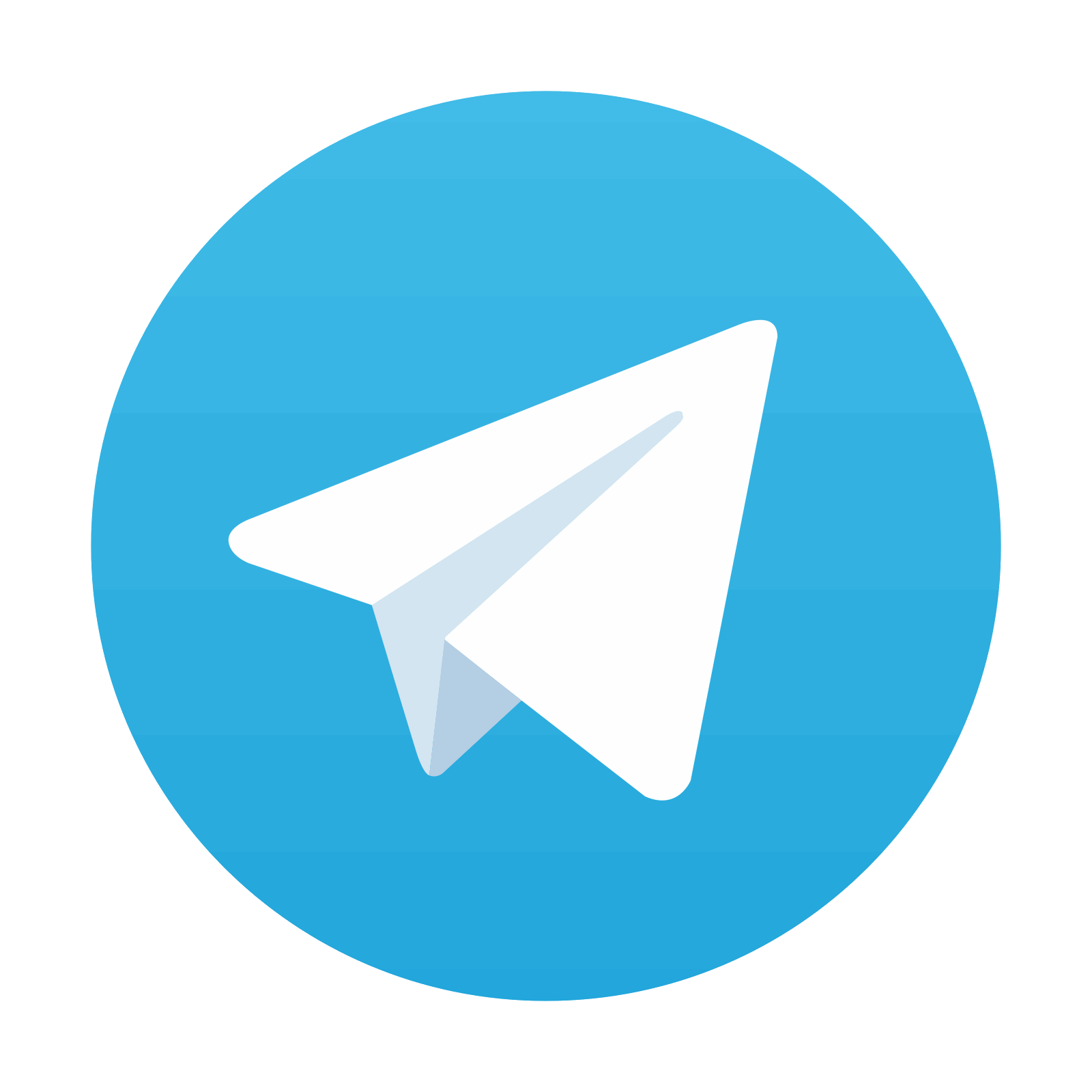
Stay updated, free articles. Join our Telegram channel

Full access? Get Clinical Tree
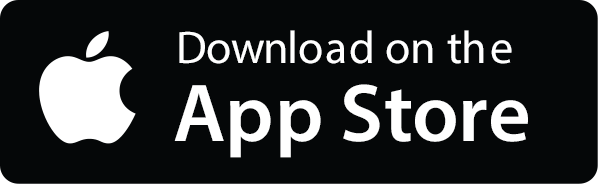
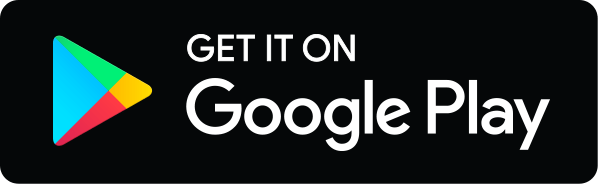