Fig. 59.1
Intracranial monitoring with grids and strips
Based on the results of the neuroimaging studies, patients are divided into those with and without space-occupying lesions. A space-occupying lesion is generally a tumor, a vascular anomaly, or a well-defined developmental abnormality such as a hamartoma or focal cortical dysplasia.
Resective surgery could proceed without phase III investigation if an interictal or ictal EEG or both demonstrated abnormal activity in the same area as the space-occupying lesion, and there is no contradictory localizing information from other tests. In these cases, the resection includes the lesion and is extended to surrounding cortex to an extent determined by results of intraoperative electrocorticography, pathological evidence of normal cytoarchitectonic margins, or both. If the resection area is adjacent to or in important functional cortex, intraoperative mapping/monitoring can be used. For patients with space-occupying lesions who do not fit these criteria, phase III is done to provide EEG localization as well as extraoperative functional mapping as needed.
Patients without space-occupying lesions fall into two categories: those with suspected neocortical epilepsy or those with temporal epilepsy. In patients with mesial temporal lobe epilepsy, if there is concordance with the information from phases I–II, resective surgery could proceed without phase III investigations. If no area of resection is defined or further functional information is required, then intracranial recording (phase III) is considered necessary for localization of epileptogenic area and functional mapping.
After the evaluation is complete, patients are determined to be eligible for resective surgery or not. Ineligible patients for resective surgery are considered for surgery that disconnect and prevent propagation of epileptic discharges such as multiple subpial transections [10] or vagus nerve stimulation [11].
Novel techniques include radiosurgery [12] and magnetic resonance-guided laser-induced thermal lesioning protocols of epileptogenic foci [13].
Medial Temporal Lobe Epilepsy
Background
The temporal lobe was postulated as a site for onset of seizures in the late 1800s [14]. The behavioral and electroencephalographic characteristics of these seizures were becoming increasingly understood by the middle of the last century [15–18] enough that subtotal anterior temporal resections—to include the mesial structures (inferior amygdala, hippocampus, and parahippocampus), as well as anterolateral neocortex—began to be performed for intractable temporal seizures [19, 20]. Surgeons found the mesial structures often were sclerosed—but the cause was uncertain (and remains incompletely understood [18]).
The syndrome of medial temporal lobe epilepsy (MTLE) is now well described, allowing it to be generally distinguished from seizures beginning outside the temporal lobe, and it remains important to aim to differentiate them from seizures arising in the lateral temporal lobe [21]. Febrile seizures are a risk factor. Family history of seizures may be reported. Clinically, visceral sensations are the most common auras; the next most common is fear. Electrophysiological features of temporal seizure activity, particularly medial temporal lobe location are observed, both interictal and ictal. On MRI, the most common lesion is hippocampal sclerosis (hippocampal shrinkage and increased T2 signal), but other abnormalities can be seen such as dysplasias (some are microdysplasias and only appreciated pathologically), tumors, and vascular malformations. These lesions can be considered examples of “dual pathology” in the temporal lobe and may suggest a more generous resection than lesionectomy is needed [22].
When an adequate constellation of features is present, they permit a diagnosis of MTLE with enough confidence to consider surgical resection for seizure control. Corroborative testing for localization can include magnetoencephalography, PET, ictal SPECT, magnetic resonance spectroscopy, or invasive monitoring [23]. Neuropsychological testing and testing for language and memory (e.g., WADA, fMRI) can provide further information on function and localization, help plan resections, predict functional reserve after surgery, and establish baselines. The precise “origin” of the seizure(s) is generally not known; but rather the investigations implicate the mesial structures but also more widespread connected regions [21, 24–26].
Procedures
Penfield and Baldwin [19] described the basic resection, beginning with anterolateral temporal cortex, limited posteriorly to the vein of Labbe, and below the Sylvian fissure, but they often individualized this by pathologic findings and electrocorticography with mapping, frequently with the patient conscious. As mentioned above, the mesial structures were removed to the pia overlying the basal cisterns, including uncus, inferior amygdala, and hippocampus [19, 20] (Fig. 59.2).
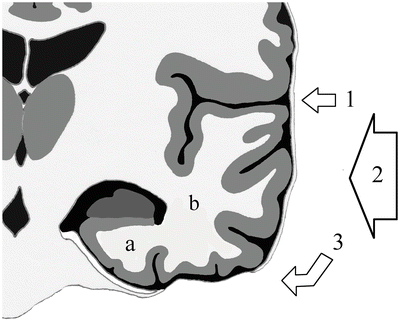
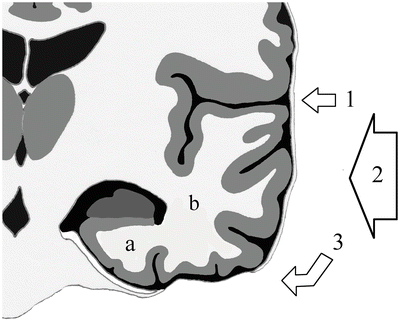
Fig. 59.2
Schematic of cross section of the temporal lobe demonstrating the medial temporal lobe (a) and lateral temporal lobe (b). Temporal resections for epilepsy may focus on lateral temporal cortex, medial temporal structures, or resection of both. Specific approaches through or “around” the lateral temporal structures, to approach the medial structures more specifically, include: transsylvian (1), transtemporal (2), and subtemporal (3)
Over the past 60 years, one of the interests in the surgical procedure for MTLE has been to decrease the extent of tissue removal aiming to minimize any deficit while maintaining the benefit of surgery. Also, in this endeavor, there was often a goal to better identify the structure(s) whose removal is critical for success at relieving seizures. This conceivably could lead to increasing surgical success rate. Study of modifications of subtotal anterior temporal resections continues today. However, no modification is known to be most efficacious for seizure control, nor is there consensus on the structure(s) to be removed for surgical cure of seizures [27]. This is reflected in the fact that the basic procedure described by Penfield and Baldwin [19] and Falconer [28] has a good success rate (comparable to the range of techniques described), favorable side effect profile and remains a standard contemporary surgical treatment [29]. A major determinant in developing operative variations in the temporal lobe was the location of temporal neural tissue critical to language function, generally felt to be largely in the superior and more posterior temporal lobe. The operation of Penfield and Baldwin [30] and Falconer [20] frequently used lateral tailoring during awake craniotomy to avoid this language cortex. Ojemann et al. [31] emphasized and further developed this issue.
For surgery under general anesthesia, more standardized or measured resections were developed: the lateral neocortex was resected approximately 5–6 cm from the temporal pole on the nondominant cortex (slightly less on the dominant side) with mesial structures removed [32–34]. Although such margins if electrically mapped could include some positive language sites, experience indicates including some of these sites with standardized resections is still safe for language outcome [29, 32, 35, 36]. These “standard” lateral resection margins have continued to decrease in recent decades. Frequently the superior temporal gyrus resection is minimized [32, 37], while lateral resections of the other lateral temporal gyri (both dominant and nondominant) have been considered at 4.5 or 3.5 cm [29, 37, 38] or even smaller at about 2 cm [39]—although our understanding of the effects of these variations remains incompletely understood. Other approaches have been described, including: the approach of Niemeyer [40] who described a lateral approach through the lateral temporal cortex into the ventricle, thus permitting resection of mesial structures; transsylvian approaches for mesial excision [25, 41]; and subtemporal approaches [42, 43] (Fig. 59.2).
The development of intraoperative neuronavigation has lead to further refinement of the surgical approach [44] (Fig. 59.2).
In addition to these frequently used open surgical approaches, stereotactic approaches have also been evolving for lesioning of mesial structures. Radiofrequency lesions of mesial structures have been described to result in success rates for seizure control similar to that of open surgery, but experience is limited [45]. These centers have also examined the question of which structure must be lesioned to obtain seizure control. Although there was a general relationship between lesioning of mesial structures and outcome, they did not find a significant correlation between lesion of particular mesial structures (e.g., hippocampus, amygdala, entorhinal cortex) and outcome [46]. Recently, successful magnetic resonance-guided stereotactic laser-induced thermal lesioning of mesial structures for epilepsy has been described in a case report [13].
Outcomes
Outcomes in terms of seizure control, for these variations on this procedure, have been described by many groups and have been notably similar. In surveys, Engel et al. [47] reported seizure freedom before 1985 at 56 % (2,336 pts) and 1986–1990 at 68 % (3,579 pts) (1 year or more follow-up). Wiebe et al. [48] performed a randomized trial of epilepsy surgery (subtotal anterior temporal lobectomy) compared to medical treatment and reported that at 1 year 58 % of patients in the surgical group were free of disabling seizures compared to 8 % in the medical group (Class I evidence for significant benefit). Engel et al. [36], in 2003, surveyed the surgical literature since 1990 and included 24 papers with retrospective descriptive data and reported 67 % of 1,952 patients were free of disabling seizures—emphasizing the similarity of this result to the evidence from the Class I study [48]. Seizure outcomes across the different methods of partial temporal resection, as mentioned above, continue to appear similar [27].
The critical structure(s) for excision for successful treatment of seizures remains uncertain despite many years of attention. Although most evidence implicates the mesial structures (hippocampus, inferior amygdala, parahippocampus), the correlation between their removal and outcome is not tight [27] (for review see Schramm et al. [27]). For instance, resections that retained the hippocampus could provide seizure control [49], but not as great as when the hippocampus was also removed. Jack [50] reported that seizure outcome did not seem related to the extent of removal of any particular mesial structure, but to some damage to all based on post-op MRIs. Also based on post-op MRIs, Siegel [51] reported with transsylvian mesial resections that seizure outcome appeared more related to the extent of removal of the parahippocampal gyrus than the amygdala or hippocampus. Wyler et al. [52], in a randomized study, reported that seizure outcome was significantly better with surgery aimed at hippocampal removal to the level of the posterior midbrain (69 % seizure-free at 1 year) compared to mesial resections only to the anterior midbrain (38 % at 1 year). Bonilha et al. [53], from post-op MRIs, reported a significant correlation between the extent of hippocampal removal and outcome and further improvement when combined with entorhinal cortex removal. Schramm et al. [54] in a randomized study, reported that 2.5 cm vs. a 3.5 cm hippocampal removal was not associated with difference in seizure outcome (74 % vs. 73 % free of disabling seizures) (included post-op MRI confirmation of significantly differing resection volumes).
It has been suggested the variety of resections utilized in the anterior temporal lobe for MTLE may be effective by “severing a critical proportion of the connections” [55], necessary to support a seizure. Although this circuitry is likely concentrated medially in the temporal lobe, its more widespread and possibly variable nature [56] may be why 20–40 % of surgically treated patients are not cured by surgery and a consistently critical structure for removal has not been identified. It should also be noted that long-term outcomes need to be considered and have been reported to decrease gradually at longer post-op periods [57], but further longer-term data is needed. Reported success rates of reoperation for continuing or recurrent seizures are variable but most commonly below 50 %. Patient selection appears to be important in this situation [58].
Since the effectiveness for seizures is similar for the different resective approaches, focus on other outcomes has also been of major interest. Wiebe [48] in their randomized study demonstrated a significant improvement in quality of life (QOL) in the surgical arm. Engel et al. [36] reviewed and described that improved QOL measures were primarily related to freedom from disabling seizures post-op. Other evaluations have included neuropsychological testing and this remains a major area of interest today. In a recent review Sherman et al. [59] describe decreases in verbal memory and naming after left-sided surgery as being the most consistent change after temporal resections (different resection types included), but with patients usually not self-reporting changes. Loss of visual memory was less common but similar after surgery on either side.
Advantages in neuropsychological outcomes after more selective resections compared to resections with generous lateral removal have been reported [60–62], but this was not seen in other studies [63, 64]. Further complicating the situation, there may be different effects of different procedures in the different hemispheres. For example, Helmstaedter et al. [39] describe how left resections were worse for verbal memory with selective amygdalohippocampectomy being more deleterious than an anterior neocortical temporal resection plus mesial resection. Right resections were worse for figural memory, but on this side the anterior temporal resection plus mesial resection was worse than the selective mesial resection. Tanriverdi et al. [65] described that verbal memory decreases more with left-sided selective amygdalohippocampectomies compared to more standard left temporal resections. On the right, nonverbal memory decreased especially after the standard temporal resections compared to the selective resection.
There is a general agreement that an important effect on neuropsychology after resections involves the functionality of the resected tissue; for example, there are higher risks to memory associated with resections that include a normal-appearing hippocampus vs. sclerotic [66]. However, this remains an evolving area—partly because of ongoing interest in defining meaningful behavioral results that may vary between surgical methods described above and radiosurgery [12].
Complications can occur with any of these resective procedures, for example, diplopia from irritation of cranial nerve III or IV, a stroke from injury of the arteries or veins in the basal cisterns or insula, and injury to the basal ganglia superior to the amygdala (for further discussion, see [29, 38,67]). Postoperative dysphasia can occur but is usually transient [29]. Lastly an upper quadrantanopsia is common from involvement of visual fibers variably sweeping around the temporal horn [68].
Hypothalamic Hamartoma
Hypothalamus hamartomas (HH) are rare, congenital heterotopic lesions in the region of the third ventricle and tuber cinereum that are intrinsically epileptogenic when closely connected to the mammillary bodies [69]. The prevalence is 1 in 50,000–100,000 [70].
Patients classically present with isolated fits of ictal laughter (gelastic epilepsy) or a combination of gelastic and other types of seizures during the first years of life that are often particularly drug resistant from the onset [71].
Although there are several classifications available for these lesions, two clinical syndromes have been recognized: precocious puberty and intractable epilepsy including gelastic seizures. A significant number of children have both problems. The natural history is unfavorable in the majority of patients because of behavioral symptoms and mental decline; these include oppositional defiant disorder (83.3 %), attention-deficit/hyperactivity disorder (75 %), high rates of conduct disorder (33.3 %), speech retardation/learning impairment (33.3 %), and anxiety and mood disorders (16.7 %). Significant rates of aggression have been noted with 58 % of the seizure patients meeting criteria for the affective subtype of aggression and 30.5 % having the predatory aggression subtype [72].
Typically, medium/large sessile lesions within the third ventricle are more likely to lead to the seizure disorder [73] than pedunculated lesions, which hang inferiorly from the tuber cinereum and are more likely to result in central precocious puberty. Resection of pedunculated lesions causing precocious puberty reverses the hormonal abnormalities.
The first successful and safe removal of an HH was reported by Paillas et al. in [74], but the interest for the surgical cure of this specific group of patients was primarily developed in the 1990s. The association between the HHs and epilepsy was obscured by the fairly generalized spike and wave EEG patterns usually observed. Consequently, patients underwent temporal and frontal resections without seizure relief. The emphasis changed, however, due to the information gleaned from ictal single-photon emission computed tomography (iSPECT) and ictal depth recordings from the lesion itself [75] implicating the epileptogenicity of the hamartoma. High-quality magnetic resonance (MR) imaging has strongly facilitated the diagnosis of HH in cases of severe epilepsy in children. Delalande classified HHs into four types: Type I has a horizontal implantation plane inferior to the floor of the third ventricle; Type II within the third ventricle, with a vertical insertion plane; Type III is a combination of Types I and II; and Type IV includes all giant hamartomas [76].
Delalande et al. [76, 77] and Choi et al. [78] have emphasized that the goal of surgery is to resect and/or disconnect the hamartoma from the adjacent hypothalamus and to preserve the mammillary bodies (which may be malpositioned and deformed), mammillothalamic tracts, tuber cinereum, and hypothalamic nuclei. The spread of seizures occurs in part via the mammillary body. The surgery should ideally be performed before the onset of secondary generalized epilepsy [70, 79, 80]. Larger lesions might require more than one disconnection procedure, and some might require a multistep surgical approach.
The microsurgical resection in this critical area is attended by a significant risk of oculomotor palsy, hemiparesis, hypothalamic deficit, and/or visual field defect, and many epilepsy surgeons have therefore abandoned this type of approach [81]. Resections have often been subtotal and only partially effective in controlling seizures, but still associated with significant complications.
There are three typical microsurgical approaches:
Comparing the three approaches, the transcallosal anterior interforniceal offers the best chance of seizure freedom with less morbidity than the pterional route. Concerns regarding the impairment of short-term memory in children, who are cognitively intact, have promoted the exploration of surgical alternatives, e.g., endoscopic disconnection, radiofrequency obliteration [83–85], or magnetic resonance stereotactic laser ablation [13], and also nonsurgical therapies such as radiosurgery [86].
Surgical Techniques
There is literature describing the success rate of resection of just the lesion (lesionectomy) vs. lesion resection plus surrounding tissue (that may demonstrate electrographic abnormalities). Although lesionectomy is quite successful for seizure control, the excision of adjacent irritated cortex likely can provide further benefit—particularly in patients with a longer history of seizures [90–92]. Disconnective procedures are based on the concept of interrupting the spreading of the epileptic discharge in the brain, by isolating the primary epileptogenic zone. This is the basic concept for hemispherectomy, multilobar disconnection, hypothalamic hamartoma disconnection, corpus callosotomy, and multiple subpial transections.
Hemispherectomy and Hemispherotomy
Dandy [93] first reported the anatomical hemispherectomy in 1928 for the treatment of gliomas. In 1938, McKenzie [94] first reported its use in the treatment of epilepsy. Anatomical hemispherectomy was popular until 1966, but became more selectively utilized because of postoperative complications, which could include superficial cerebral hemosiderosis and a significant incidence of hydrocephalus [95, 96]. A major factor leading to the development of hemosiderosis was the large cavity created following removal of the hemisphere. Hence, various surgical modifications have evolved as alternatives, attempting to minimize the removal of brain but maximize the disconnection of white matter.
One of the most popular techniques, the functional hemispherectomy, was described by Rasmussen [97] in 1983, who modified the technique of the anatomical hemispherectomy to remove part of the central and temporal regions while disconnecting the rest. In the early 1990s, a new era in functional hemispherectomy was initiated with the introduction of the hemispherotomy, with the aim of removing as little brain as possible, and two different approaches were described almost simultaneously by Delalande [98] and Villemure [99, 100]. Since the first description of those techniques, a number of modifications have been made to reduce the number of complications [70]. The hemispherotomy techniques described include the vertical parasagittal hemispherotomy by Delalande and associates [98], the periinsular hemispherotomy by Villemure and Mascott [100–102], and the transsylvian keyhole hemispherotomy by Schramm et al. [103].
Other Disconnective Techniques
Daniel et al. [104] reported the posterior quadrantectomy for patients with refractory temporo-parieto-occipital epilepsy. Three variants were utilized in this series, namely, (1) anatomical posterior quadrantectomy, which entails the removal of the temporal, parietal, and occipital lobe; (2) functional posterior quadrantectomy, where an extended temporal lobectomy is performed and then parieto-occipital lobe is disconnected; and (3) periinsular posterior quadrantectomy; in this surgery no lobe is resected, and the entire temporo-parieto-occipital is disconnected but remains viable because of the preservation of vessels supplying or draining the disconnected lobes [105]. They reported excellent results. Chabardes et al. [106] published a series of 47 patients with temporal disconnection procedures guided by neuronavigation. Outcomes and complications are within the range of that expected with resective procedures described in a prior section.
Corpus Callosotomy
First described in 1940 [107], corpus callosotomy has been reserved for the treatment of generalized epilepsy or partial epilepsy with secondary generalization where the onset cannot be localized. Corpus callosotomy is particularly effective for the drop seizures of Lennox-Gastaut syndrome [108], a severe childhood epilepsy disorder characterized by encephalopathy and multiple, often intractable, seizure types. The drop attack is the most frequently recognizable seizure type in this patient population and is also the most dangerous physically, thus severely limiting quality of life. The diagnosis is confirmed by electroencephalography, for which the classic pattern is a slow 2.5 Hz generalized spike and wave [109]. Seizure improvement ranges between 50 and 81 % with complete callosotomy [110–112]. The complete callosotomy is a palliative procedure reserved for nonverbal patients with severe intractable epilepsy because it often produces a hemisphere disconnection syndrome that can result in disabling symptoms of intermanual conflict [113]. The 2/3 anterior partial callosotomy does not usually have significant cognitive consequences. It is because of the potential for the development of this disconnection syndrome that partial callosotomy is usually undertaken initially, and the section can be completed if there is an inadequate seizure control.
Multiple Subpial Transections
First described by Morrel in 1969, the multiple subpial transections (MST) procedure was designed to treat patients with epileptogenic zones in functionally critical cortical areas where respective surgery could cause unacceptable neurological deficits [114]. The rationale is based on the principle that vertical columns are essential in the propagation of normal cortical activity from cortex to subcortical structures. Therefore, the transection of horizontal cortical-cortical interneuronal connections will interrupt local synchronization of epileptogenic activity and the spread of the epileptic discharges [115].
Although it is a well-known technique, MST is the least frequently performed procedure in epilepsy surgery (on the order of 0.6 %). It is difficult to assess the effectiveness of MST because the majority of the reports used MST in combination with resective surgery [116–118]. The use of MST as a stand-alone procedure has been reported by Schramm [119], Smith [120], and Whisler [121] with a seizure-free outcome of 5 %, 37.5 %, and 63 %, respectively. Tellez-Zenteno et al. [122] in a meta-analysis study concluded that MST has the lowest rate of long-term seizure-free outcome (16 %) among all epilepsy procedures.
Electrical Stimulation for Epilepsy
Advantages of electrical stimulation therapy for epilepsy include reversibility, adjustability and that inaccessible and multiple targets may be influenced. Presumably the stimulation disrupts the abnormal electrical “buildup” of the seizure, but both the mechanism and circuitry remain unclear.
Vagal Nerve Stimulation
Vagal nerve stimulation (VNS) had been known to ameliorate experimental seizure activity since the 1950s [123], and a series of clinical studies supported its utility in the mid- and late 1990s. In patients with primarily focal seizures, after VNS implant, patients were randomized between stimulation that was either “high” (“typical” and felt to be active) or “low” (low frequency and hypothesized to be ineffective). Blinding was not likely complete as some patients and observers may have intuited the group they were in. In the initial randomized study (n = 114), there was a 24.5 % reduction of seizures in the “high” stimulation group at 14 weeks—6 % in control [124]. In the 2nd study (n = 196) after 3 months, the seizure frequency declined 28 % in the “high” stimulation group—vs. 15 % in the “low” [125]. DeGiorgio et al. [126] reported on patients from this latter trial who were followed unblinded with “high” stimulation and reported a 45 % decrease at 1 year. Over 400 patients from early trials [127] were followed, and seizure reductions of 35 % at 1 year, 44 % at 2 years, and 44 % at 3 years were reported. Approval for the device was received for adjunctive treatment for intractable focal seizures in the United States in the late 1990s.
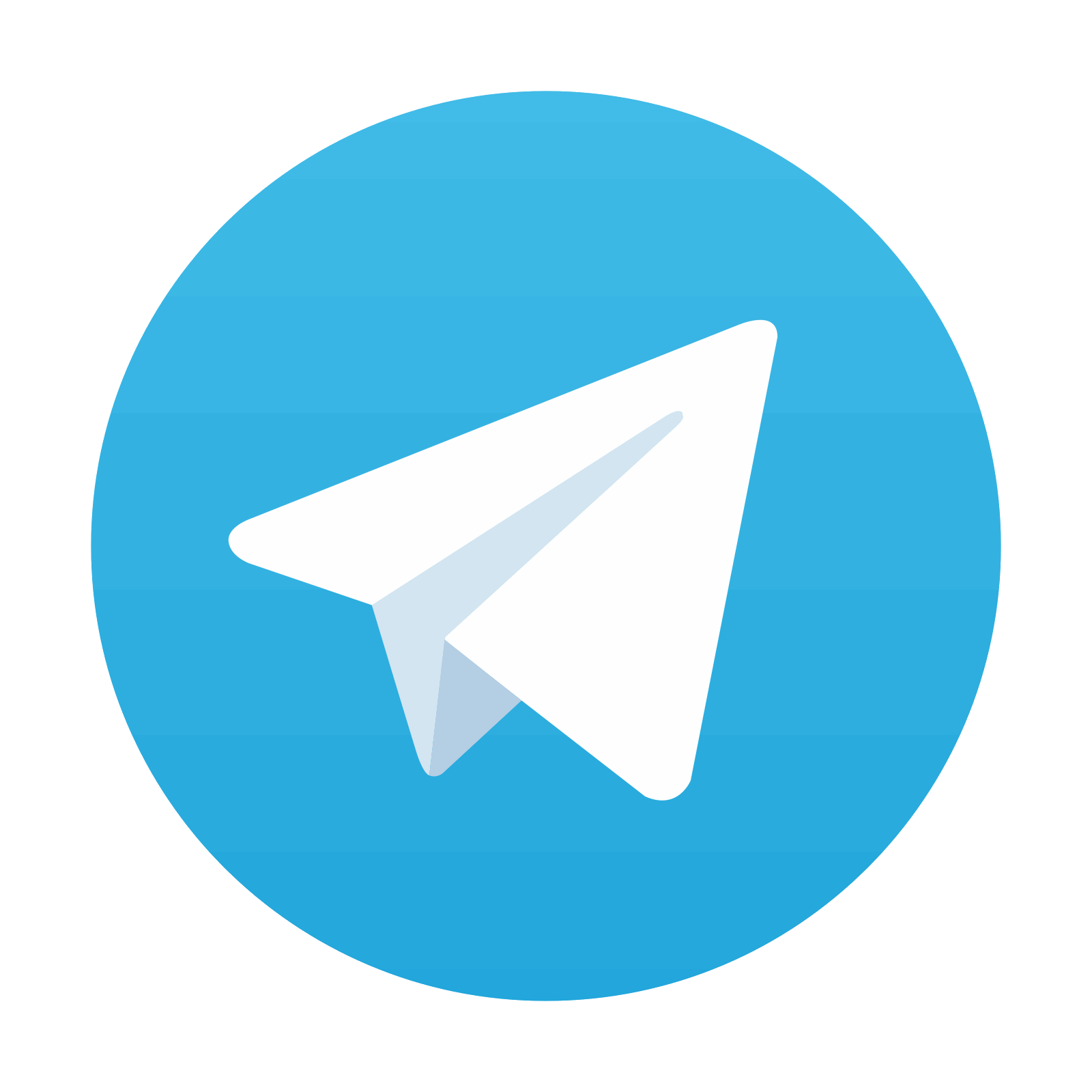
Stay updated, free articles. Join our Telegram channel

Full access? Get Clinical Tree
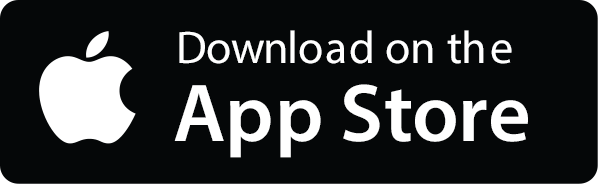
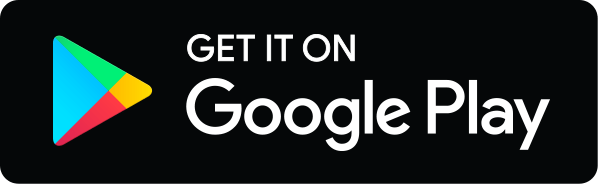