Equipment
Point-of-care ultrasound has grown rapidly over the last two decades. Practitioners in virtually all fields of medicine have moved ultrasound image acquisition and interpretation out of imaging suites and to the patient’s bedside in a multitude of clinical settings. Not unexpectedly, the ultrasound equipment market has developed at an astonishing rate, leading to a wide range of choices of ultrasound equipment available to clinicians.
GENERAL CONSIDERATIONS
PORTABILITY
Manufacturers have pushed the boundaries of ultrasound equipment creating a range of sizes. Top-end machines found in radiology suites are still generally larger machines best suited as stationary pieces of equipment (though even these may be moved fairly easily by a single individual). Smaller and lighter machines are now commonly found throughout hospital and outpatient settings. Durable handheld units are used in the prehospital and military combat settings.1,2 Recently, ultrasound devices small enough to fit into the pocket of a clinician’s white coat have been brought to market.
The size of a system should play an important role in purchasing decisions. Cart-based, handheld, and hybrid systems all deserve consideration depending on the clinical environment. Hybrid systems offer a cart from which a handheld component may be removed for easy transport. In general, cart-based systems are higher-end machines offering better imaging and more software options. However, the performance gap between cart-based machines and handheld machines is narrowing.3
The ED and critical care unit generally require some form of cart-based system. Several transducers are necessary for the growing number of applications that emergency and critical care physicians utilize; finding places to set a handheld machine while scanning can be difficult. Cart-based machines have varying amounts of storage space for commonly used adjunct equipment, such as ultrasound gel, transducer sheaths, printers, recording devices, and cleaners.
A removable component is beneficial when other areas of the hospital are covered for “code” situations or when a cart will not fit into the nooks and crannies of a treatment room overflowing with equipment and patients. Handheld-only options may be more appropriate for office-based practices, prehospital providers, and military providers who perform a more limited range of studies in a setting where the importance of small size trumps improved functionality.
POWER
Power is generally not the first consideration for clinicians when purchasing ultrasound equipment. However, the ultrasound machine battery power options and boot-up time may make the difference between a tool that is used regularly in practice and one that sits in the corner collecting dust.
Many companies offer products that are powered both via wall outlets and rechargeable battery packs. Most products with batteries allow for seamless use of the device as it is unplugged, which can be a huge advantage in situations where clinicians move quickly between patients.
The boot-up time of a machine is an important consideration. Ultrasound machines that take more than 30 seconds from power-switch to general use are impractical in the ED and critical care units. Beyond the obvious drawbacks in critical situations, machines with long boot-up times lead to physician aggravation and less use of the machine. No clinician wants to wait for their machine to start while other responsibilities await.
Another important consideration is the power cord itself. Machines in the ED are moved rapidly between patients by many different people with varying levels of concern for the machine. The power cord and its connection to the machine take a lot of abuse when it is run over or pulled from the wall. Devices should have a specific place to store the cord or the ability to retract it completely.
POWER FOR ANCILLARY DEVICES
Most devices provide outlets that are used to power ancillary devices (thermal printers, video recorders, or gel warmers) and some require specialized adapters. Specialized adapters may not be a problem if you plan to store images/video on the hard drive of the machine or other devices such as a USB drive, but realize that if the outlets are too few or too specialized, your options may be limited.
TRANSDUCER CHANGERS
Different ultrasound applications require different transducers so the ability of a clinician to instantly switch between transducers is important. Many cart-based systems have several “active” ports that allow the user to switch between transducers at the push of a button. Machines that only allow one transducer to fit into the machine at any given time have one “active” port, and several “storage” ports where other transducers are held while not in use.
Multiple active ports are essential for full use of an ultrasound machine in an ED or critical care setting. Emergency physicians perform many types of scans and often in rapid succession. Untangling cords and physically changing transducers between patients and scans is frustrating and time consuming. This is particularly evident when clinicians care for a trauma patient and need to seamlessly move between the linear transducer (to assess for a pneumothorax and obtain central venous access) and the small footprint phased array transducer (to assess for hemoperitoneum, hemopericardium and hemothorax).
BASIC KNOBOLOGY
There are many different ultrasound machines, but they all have the same basic controls. Any practitioner with ultrasound experience should be able to operate any machine, no matter where it is found in the hospital or how many knobs are found on the control panel. The next section identifies the basic controls that are present on every ultrasound machine, from a single-transducer device found in a clinician’s pocket to the fully loaded models found in a radiology suite.
CONTROL PANEL
Control panels on ultrasound machines vary widely. Machines with more bells and whistles tend to have more buttons and knobs compared with very portable machines with only the essentials. More complex control panels may intimidate clinicians who are novice to ultrasound. As the graphical user interface of tablet devices and other handheld electronic devices moves in a direction lacking buttons, so too will the portable ultrasound units. Touch screens employing no physical “buttons” will likely become the norm.
The durability testing manufacturers undertake should be considered. Machines with more buttons and knobs may also have more cracks and crevices through which fluids may enter and disrupt function. Another minor question relates to the difference between trackballs and track pads. Trackballs may work more quickly and precisely than a track pad (especially with gelladen gloves). However, they also may become clogged with gel or other substances necessitating removal and cleaning.
ACOUSTIC POWER
The acoustic power (also called output power) relates to the amplitude of sound waves produced by the transducer and helps determine the brightness and quality of the image. Increasing the acoustic power results in higher transmitted amplitudes and stronger returning echoes. Greater acoustic power may improve image quality by increasing the contrast between light and dark areas on the display. However, if the power is too high, lateral and longitudinal resolution will decrease.
Acoustic power is directly related to intensity. The intensity of the ultrasound beam, meaning the amount of energy in a given area, determines the bioeffects of ultrasound. As the intensity increases, the amount of heat produced in the tissue increases, which potentially could cause tissue injury. While no studies have provided concrete evidence that diagnostic ultrasound has deleterious effects on tissues, including fetal tissue, practitioners using ultrasound work by the ALARA (As Low As Reasonably Achievable) principle, meaning we use the lowest possible power setting necessary for creation of the appropriate image.4 Potential bioeffects are especially important when scanning pregnant women and the eye. The obstetrics and ocular presets on a given machine appropriately adjust the power output to FDA-approved levels for these tissue types.
Most machines do allow the clinician to adjust the power. However, the controls for acoustic power are generally not found on the primary control panel on portable machines. With some more basic machines, the power is only adjusted by toggling the presets.
GAIN
The primary control clinicians use to adjust brightness is gain. When an echo returns from the body to the transducer, it does so within an amplitude range. The ultrasound device translates that amplitude range to a brightness, which is displayed on the monitor. The overall gain allows the clinician to adjust the brightness of all returning echoes, thereby adjusting brightness over the entire screen. Care should be taken not to over-gain images. Despite the perception of many novice ultrasound operators that brighter is better, increased gain can lead to loss of subtle findings.
Both acoustic power and gain change the brightness of the image. Power changes the brightness by changing the strength of sound entering the body, thereby increasing the strength of returning echoes. Gain changes the brightness by adjusting the amplification of the electronic signals after the echoes have returned to the transducer (Figure 2-1). Therefore, when an image is not bright enough, the user should first adjust the gain to improve the image.
Figure 2-1. Gain—over, under, correct. (A) (Over) The image has too much gain applied to the image. Compared with image C, echoes are found where there should be none. (B) (Under) The image is under-gained. The periphery of the image is very dark, potentially making an accurate diagnosis very difficult. (C) (Correct) Appropriately gained. (Courtesy of Ultrasonix)
TIME GAIN COMPENSATION
Time gain compensation (TGC) allows the clinician to adjust the brightness of the image at different depths. To understand TGC, one must understand attenuation. Attenuation is the progressive weakening of the ultrasound beam as it passes through tissue. Attenuation occurs due to absorption, reflection, and scattering of sound energy away from the transducer. If the ultrasound device were to display the actual amplitude of returning echoes, the image would become progressively darker from superficial to deep. Accordingly, ultrasound devices are built to compensate for attenuation by increasing the brightness that is displayed for structures that are deeper in the body in order to create an image with the same echogenicity from top to bottom.
Ultrasound devices often make this adjustment with slight inaccuracies because structures attenuate sound at different rates. As an example, the very bright image that is typically displayed posterior to a bladder in a transabdominal pelvic window occurs because sound does not weaken much as it passes through fluid such as urine. Echoes therefore return with a much higher amplitude than sound waves that pass through soft tissue. The ultrasound machine interprets that to mean that there are stronger reflectors posterior to the bladder and display them much more brightly.
Clinicians can adjust the TGC to correct for inaccurate assumptions made by the device (Figure 2-2). The simplest method of adjustment is two knobs: one dedicated to the near field and the other dedicated to the far field. More complex machines have a series of sliding levers that correspond the various depths on the display. Users are thus able to adjust the gain more smoothly through the image. Newer technology now allows some machines to evaluate and better self-correct image brightness at the push of a button.
Figure 2-2. TGC—near field, far field. (A) The near field is under-gained. (B) The far field is under-gained. Compare with Figure 1c—an appropriately gained image that is uniformally “bright” from top to bottom. (Courtesy of Zonare)
DEPTH
The most frequently used button or knob on any ultrasound machine is depth. The depth function adjusts how far into the body the machine images. There are two important reasons to optimize the depth. First, the size of the display is finite and imaging to a greater depth means structures are made smaller to present more on the display. If the deeper structures are not important to the user, that area of the display is simply “wasted real-estate.” When the depth is reduced, less area is displayed making the presented structures relatively larger on-screen (Figure 2-3).
Figure 2-3. Depth—deep, correct. (A) Note the depth is set too deep, wasting valuable space on the display. (B) The depth is set correctly providing a balance between all important structures, while utilizing the entire display. (Courtesy of Zonare)
Second, adjusting the depth changes the amount of time the machine listens for returning echoes. If the depth is increased, the machine listens longer to collect data before displaying information reducing the displayed frame rate. Decreasing the frame rate may diminish the temporal resolution making the stream of images displayed less smooth to the eye, which may have a negative effect on diagnostic accuracy and procedure guidance.
The depth refresh time refers to how long it takes a machine to create a new image after the clinician adjusts the depth knob. Most machines will refresh the image almost seamlessly, but some machines may have a noticeable delay. While it may not be clinically relevant, a slow refresh rate can be frustrating to the user.
ZOOM
Most ultrasound machines offer a zoom function. The zoom function magnifies one section of the display. Importantly, the resolution remains the same, meaning the number of pixels does not change. Rather, they are magnified to create the larger image. The zoom function is most useful when the clinician wants to focus on deeper structures (Figure 2-4).
Figure 2-4. Zoom. (A) A sector of the image is selected prior to the zoom function activation. (B) The zoom function is activated, effectively magnifying the hepatic vein and surrounding structures. (Courtesy of Zonare)
Zoom and depth work through entirely different mechanisms and, whenever possible, the depth should be altered first to optimize the image. The zoom function magnifies the original data that are collected (postprocessing). The depth function actually changes the way the image is acquired (preprocessing). Decreasing the depth allows the machine to dedicate more pixels to a smaller area, thereby improving the resolution. Only use zoom when deeper structures need to be magnified.
FREEZE
The freeze button holds an image on the display. Most devices keep the last several seconds of images in memory (referred to as a “cine loop”), so when clinicians freeze the display, they are allowed to move through those saved images. The exact number of images saved in current memory varies with the device.
MEASUREMENTS AND CALCULATIONS
The ability to measure structures is very important for any machine in any clinical setting. Almost all ultrasound devices produce electronic calipers that allow the clinician to make accurate measurements. Many machines also offer packages that will use the measurements made to make clinically important calculations such as area, volume, crown-rump length, biparietal diameter, cardiac output, and others.
ADVANCED KNOBOLOGY
M-MODE
M-mode (motion-mode) ultrasound is used to graph the movement of structures within the body. The clinician focuses the machine on a narrow area of returning echoes. The machine maps the returning echoes on the y-axis with time graphed on the x-axis (Figures 2-5 and 2-6). Cardiologists use M-mode ultrasound to precisely evaluate the movement of heart valves. Common uses in emergency settings include evaluation for pneumothorax and quantifying fetal heart tones.
Figure 2-5. M-mode cardiac. The cursor has been placed over the mitral valve. The graph below demonstrates the motion of the mitral valve at the cursor over time. (Courtesy of GE Medical)
Figure 2-6. M-mode fetal heart. The M-mode line is placed through the fetal heart in this image. The movement of the heart is charted against time and can be seen at approximately 3.4 cm on the resulting graph. The movement calculates to 164 bpm. (Courtesy of Zonare)
DOPPLER
Doppler ultrasound uses the frequency shift created by the reflection of sound off a moving body to observe and describe that movement. Doppler shift is the change from the original frequency that occurs when the sound reflects off a moving structure. The amount of shift relates to the velocity of that structure. The simplest form of Doppler ultrasound audibilizes the Doppler shift. Palm-sized machines that “whoosh” in time with the pulsating flow of blood through peripheral vessels or the fetal heart are used in many areas of the hospital. Diagnostic ultrasound devices offer more advanced Doppler that visually displays Doppler shift.
COLOR FLOW AND POWER DOPPLER
Bidirectional Doppler is probably the most recognizable form of Doppler ultrasound. Doppler shift is detected and movement toward and away from the transducer is displayed in different colors—generally in red and blue (but not limited to those colors). The color image is placed on the backdrop of the gray scale image so flow can be assessed related to the surrounding anatomy (Figure 2-7).
Figure 2-7. Color Doppler. Color Doppler measures the frequency shift and displays it as color over the gray scale image. Note the color scale to the left of the ultrasound image. The blue color at the top of the scale indicates that flow toward the transducer is labeled blue. (Courtesy of GE Medical)
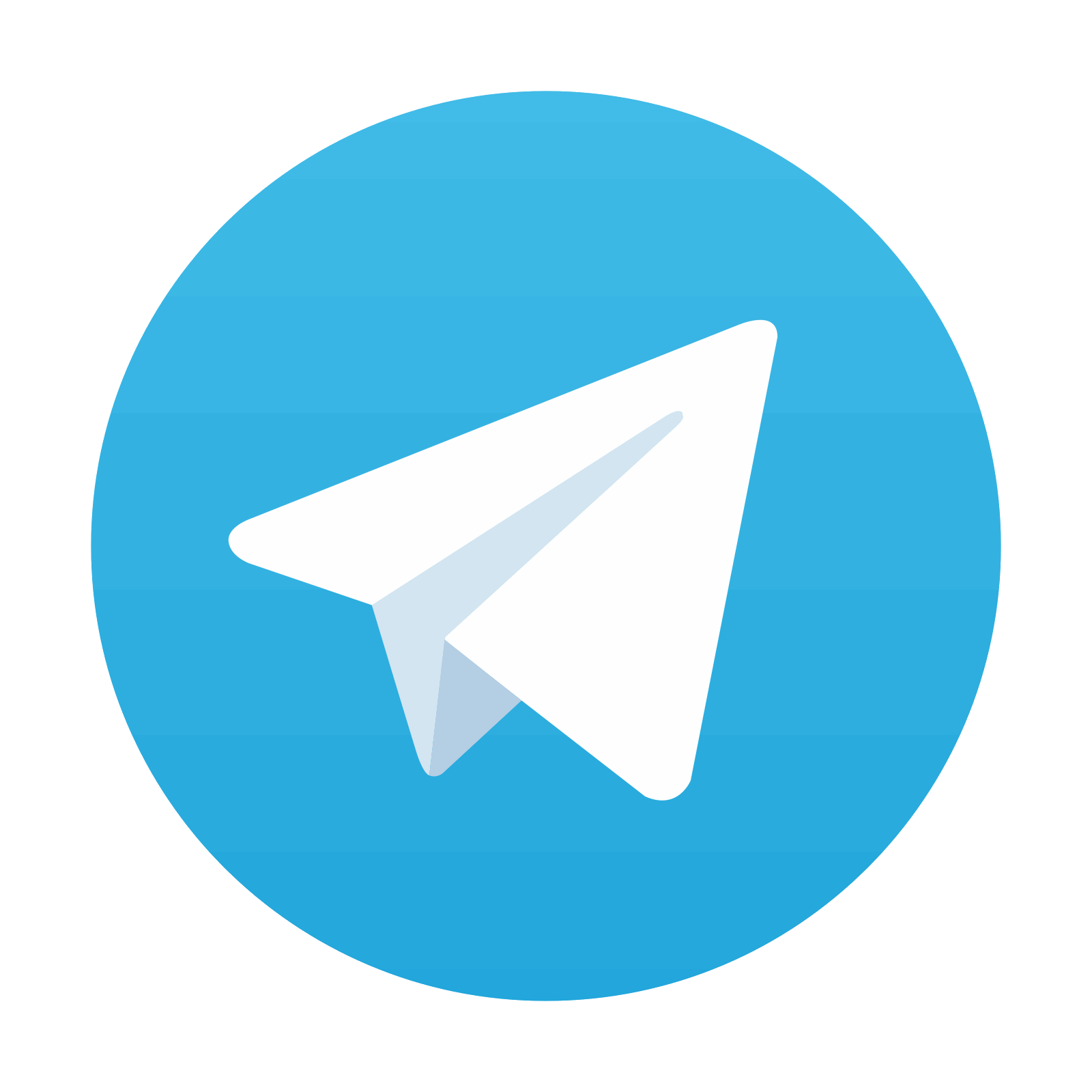
Stay updated, free articles. Join our Telegram channel

Full access? Get Clinical Tree
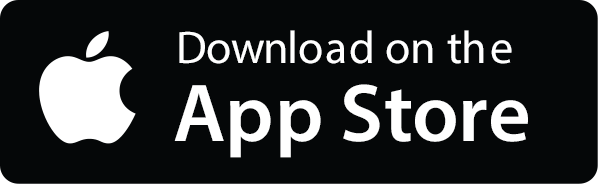
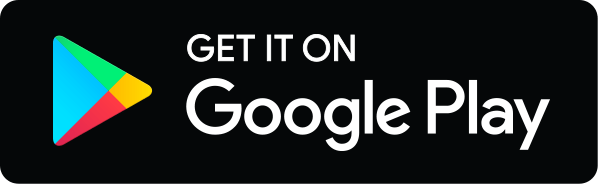