Fig. 1
Biocontinuum of adverse early and late effects of the esophagus (with permissions from Rubin and Casarett 1968)
The clinical effects of external beam (EB) radiation therapy (RT) on the esophagus were described prior to the 1960s (Seaman and Ackerman 1957; Engelstad 1934). As trends in therapeutic approaches for non-small cell lung cancer (NSCLC) led toward more intensive therapeutic regimens of RT and chemotherapy, rates of treatment-related acute esophagitis increased. Whereas the rate of grade 3 or higher acute esophagitis was approximately 1 % with sequential chemoradiotherapy (CRT), the rates of esophagitis are higher, around 27–40 %, with concurrent CRT (Byhardt et al. 1998; Dillman et al. 1990; Curran W Jr et al. 2000; Ball et al. 1995; Umsawasdi et al. 1985; Gagel et al. 2007). Higher rates of severe acute esophagitis have been associated with increased incidence of late esophagitis (Ahn et al. 2005), but late manifestations of esophageal injury remain less common than acute toxicity in the era of CRT. In recent reports, death due to late esophageal injury occurs in only 0.4–1 % of patients treated for NSCLC (Qiao et al. 2005; Singh et al. 2003). It is anticipated, however, that the burgeoning role of hypofractionated RT in NSCLC may result in higher rates of late esophagitis due to the administration of higher doses per fraction, based upon accepted principles of radiobiology (Onimaru et al. 2003; Timmerman et al. 2006).
A variety of treatment-related factors may influence the development of late effects of the esophagus after RT. An understanding of these variables will provide a foundation for efforts to limit the incidence of and to manage the symptoms of late RT-induced esophageal injury. In this chapter, we review the current understanding of RT-induced esophagitis, provide dose-volume recommendations for the clinical radiation oncologist, and describe recommendations for future directions. Bio-continuum of adverse early and late effects is shown in Fig. 1.
2 Anatomy and Histology
2.1 Gross Anatomy
The esophagus is a muscular, tubular structure that measures approximately 25 cm in length at extends from the cricoid cartilage, at the level C6 vertebral body level, to the esophagogastric (EG) junction, at the level of the T11 vertebral body level. It is located posterior to the trachea and bronchi in the posterior mediastinum. The esophagus is anterior to the spinal cord and the separation between the esophagus and spinal cord increases toward its gastric interface. The presence of an extensive network of submucosal lymphatics permits the spread of esophageal cancer several centimeters beyond the gross tumor, which is a relatively common event (Czito et al. 2008; Bradley and Mutic 2006). Sakata first demonstrated in 1903 that the submucosal lymphatics drain longitudinally, rather than in a segmental fashion (Sakata 1903). The network of lymphatics within the esophagus results in erratic spread of lymphatic metastases with frequent skip metastases to lymph nodes (van de Ven et al. 1999). Immunohistochemical analyses of surgical specimens for resected esophageal cancers have revealed a 66 % rate of skip metastases (Hosch et al. 2001). It is due to this potential for longitudinal spread that the length of longitudinal surgical margin is a predictor of outcomes after resection of esophageal cancer and that a longitudinal resection margin of 5 cm is recommended (Barbour et al. 2007) (Fig. 2).
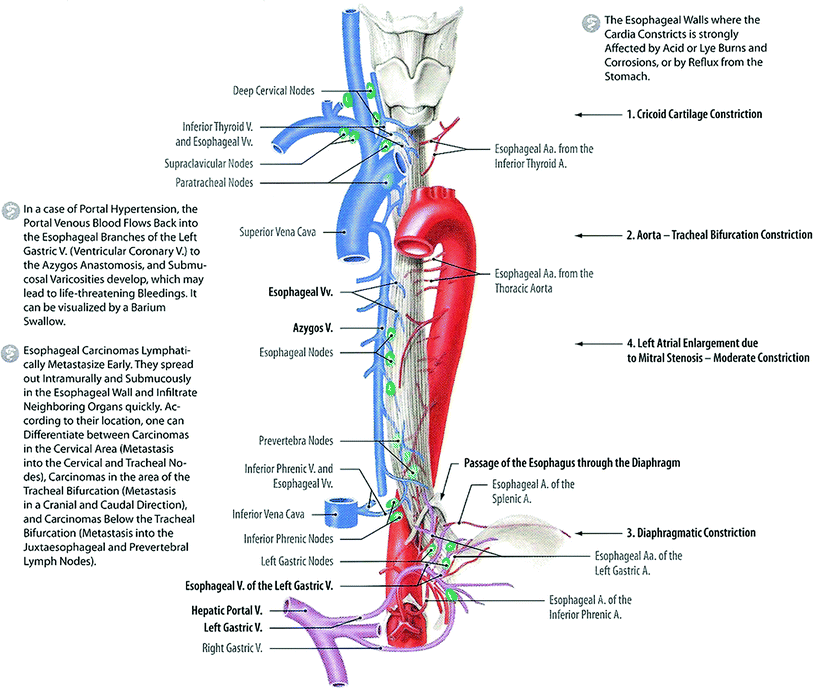
Fig. 2
Anatomy: Esophagus, Blood Supply, Lymphatic and Esophageal Sphincters: ventral view (with permission from Tillman 2007)
The esophagus is generally divided into the cervical, upper thoracic, mid-thoracic, and lower thoracic regions. In the American Joint Committee on Cancer (AJCC) Staging Manual, these regions are defined as: cervical (extending from the inferior border of cricoid to thoracic inlet, at approximately18 cm from upper incisors on endoscopy), upper thoracic (extending from the thoracic inlet to level of the carina, approximately 24 cm from upper incisors), mid-thoracic (extending from the level of the carina to just superior to the EG junction, 32 cm from incisors); and lower thoracic/abdominal, the abdominal portion of the esophagus and the EG junction (40 cm from incisors) (American Joint Committee on Cancer 2002). See Fig. 2 for esophageal anatomy and index distances from upper incisors on endoscopy (Czito et al. 2008). For RT planning, the external border of the esophagus may be defined manually on axial computed tomography (CT) images. In order to obtain an accurate and informative dose-volume histogram, the esophagus should be segmented from its origin at the cricopharyngeus muscle to its termination at the gastroesophageal junction. One recent report suggests that a “correction method” for esophageal segmentation on CT images, based upon physiological principles of the normal esophageal circumference, may improve dosimetric predictions of clinical toxicity outcomes after RT for lung cancer (Kahn et al. 2005), emphasizing the relevance of esophageal anatomy to RT planning.
2.2 Histology and the Functional Subunit
The esophageal wall contains the basic histological architecture characteristic of the gastrointestinal tract: mucosa, submucosa, two muscular layers (inner circular layer and outer longitudinal layer), and adventitia (Fig. 3a) (Rubin and Casarett 1968; Junqueira et al. 1094). It lacks serosa, a deficiency that is thought to increase the opportunity for radial extension of tumor from the esophageal wall into the periesophageal tissues. The clinical relevance, however, of the lack of serosal coverage is unclear; it is not known how much a thin serosa would protect against extramural extension for a tumor that has invaded through the muscular wall. The esophageal mucosa is composed of non-keratinized stratified squamous epithelium (Squier and Kremer 2001). The components of the esophagus may be characterized using Rubin and Casarett’s classification of radiosensitivity, which is based on cellular reproductive and functional characteristics (Rubin and Casarett 1968). According to the Rubin and Casarett system, the inner germinal stratum of the esophageal epithelium contains vegetative (Group I) and differentiating (Group II) intermitotic cells, which are considered radiosensitive. The outer germinal stratum, adjacent to the esophageal lumen, is composed of fixed postmitotic cells (Group IV), which do not multiply and are considered radioresistant (Rubin and Casarett 1968; Hall and Giaccia 2006). Although the mucosal epithelium is avascular, it receives nutrients by diffusion from capillaries contained in the lamina propria, which is also the site of venous and lymphatic drainage (Rubin and Casarett 1968). Small mucous glands, whose functions are to protect mucosa and facilitate food transport, are present within the submucosa throughout the esophagus (“esophageal glands”) and within the lamina propria in the distal esophagus near the stomach (“esophageal cardiac glands”) (Junqueira et al. 1094). The muscular components of the esophageal wall, governed by both reflexive and autonomic nervous system mechanisms, are responsible for the peristalsis and sphincter regulation necessary for food transport. When considered as an organ using the Michalowski classification, the esophageal radiosensitivity is in agreement with hierarchical (H-type) tissues, which are early-responding cell lines (Michalowski and Hornsey 1986; Wheldon et al. 1982). The esophagus is comprised of structurally undefined functional subunits (FSUs), in contrast to the lung, which implies that repopulation after RT may result from the migration of clonogenic cells from one FSU to another (Hall and Giaccia 2006) (Fig. 3a, b).
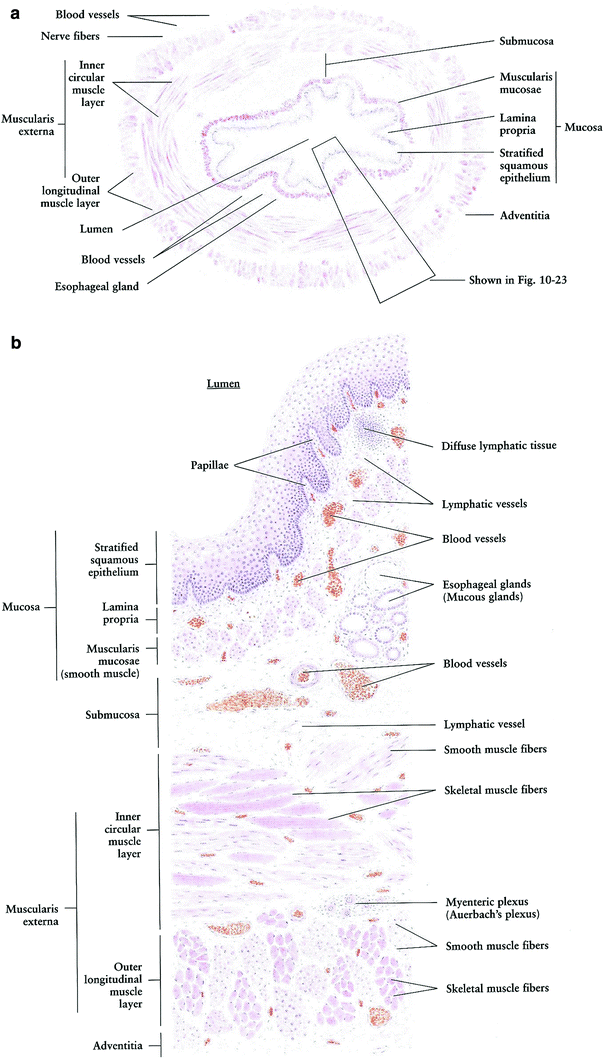
Fig. 3
a Histology: Esophagus cross section, very low magnification. b Histology: Esophagus cross section, low magnification (with permissions from Zhang 1999)
3 Physiology, Biology, and Pathophysiology
A series of molecular mechanisms (Fig. 4) lead to the clinical RT-induced esophageal injury that has been often divided into two phases: the acute phase, associated with mucosal damage, and the late phase, associated with harm to the muscular wall (Fig. 5a, b). There is significant overlap between the two phases, and the strong predictive association between the severity of acute esophagitis and the development of late toxicity (Fig. 5c) (Ahn et al. 2005) suggests a causative relationship. In their 1968 text, Rubin and Casarett wrote, “The radiation-induced responses and lesions in the esophagus and stomach are basically similar in principle and mechanism to those which have been described for the skin and oropharyngeal mucosa” (Rubin and Casarett 1968). This early statement has been confirmed by subsequent literature describing the pathophysiology of the acute and late effects of esophageal irradiation, both in animal models and in humans (Seaman and Ackerman 1957; Engelstad 1934; Rubin and Casarett 1968; Northway et al. 1979; Phillips and Margolis 1972; Phillips and Ross 1974; Novak et al. 1979; Gilette et al. 1998) (Figs. 4 and 5a, b, c).
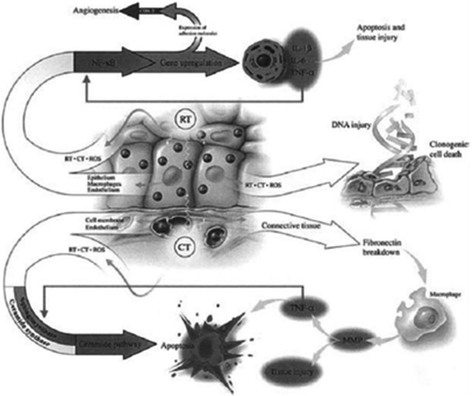
Fig. 4
Radiotherapy (RT) and chemotherapy (CT) generate ROS resulting in direct DNA injury as well as stimulation of secondary mediators leading to apoptosis. Other genes are also up regulated leading to angiogenesis. (Reprinted from Sonis ST et al. 2004; with permission)
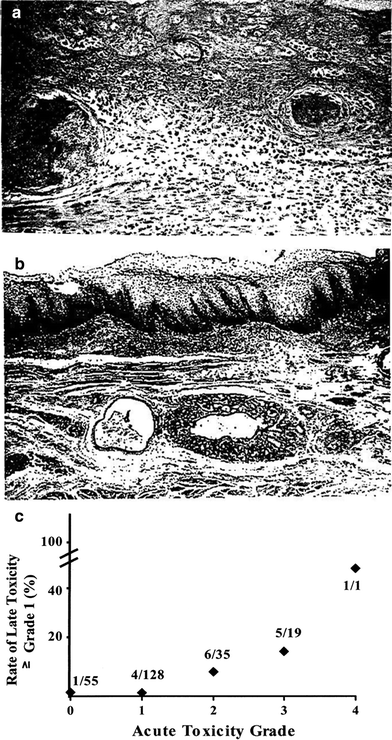
Fig. 5
a Acute: One-year earlier, this patients received 30 Gy to the esophagus for metastatic bone malignancy from breast carcinoma, and 20 days before death she received 30 Gy for sternal and cervical spine metastases. This photomicrograph shows acute necrosis of the esophageal mucosa (upper) and intense submucosal inflammation in which two thin-walled vessels are seen containing fibrin thrombi. High power. b Fibrosis: Postradiation esophageal squamous epithelial hyperplasia and parakeratosis are seen over submucosal fibrosis, distorted and atrophic esophageal mucosal glands, and several small blood vessels with fibrosed walls and narrow lumens. Low power. (with permission from Fajardo 2001). c The severity of acute esophagitis is associated with incidence of late esophageal toxicity for NSCLC patients receiving RT With permission (Ahn et al. 2005)
3.1 Physiology
The principal function of the esophagus is to deliver food from the pharynx to the stomach, and this rapid transfer requires complex coordination to ensure proper timing and anterograde direction. Although swallowing is initiated voluntarily, esophageal motility is largely under automatic control that consists of input from the brainstem and involvement of vagal parasympathetic, efferent nerve fibers and the enteric nervous system. The upper and lower esophageal sphincters must be relaxed at the appropriate time during swallowing, as these structures are closed at rest in order to prevent retrograde movement of digestive contents. Esophageal peristalsis is activated by the stimulatory effects of distention on mechanoreceptors, triggering a vagovagal reflex that is responsive to food bolus volume and temperature (Barrett 2307). The delivery of a food bolus from the oropharynx to the stomach is a complex, coordinated process dependent upon adequate function of multiple autonomic and reflexive processes.
Disruption of esophageal motility is a frequent occurrence after RT. Goldstein et al. used esophagograms to study RT-induced changes. They found motility disorders to be the most common RT-induced problem and reported loss of peristalsis in the irradiation portion of the esophagus, with adjacent areas of rhythmic, but uncoordinated, contraction after an average 50 Gy of RT (Goldstein et al. 1975). Lepke and Libshitz observed similar dysmotility after RT, which they described as developing 4–12 weeks after RT (Lepke and Libshitz 1983). Technetium scintigraphy has also been used to visualize temporarily prolonged esophageal transit times in most patients after RT doses higher than 40 Gy (Lamanna et al. 1985). Impaired esophageal motility may also be observed by manometry (Kaplinsky et al. 1991). The impaired motility may be related to neuronal and/or muscular injury (Coia et al. 1995; Kaplinsky et al. 1991). Due to the tubular geometry of the esophagus, the development of mural thickening or stricture may severely impair the transit of food (Coia et al. 1995; Fajardo et al. 2001). Esophageal dysmotility and stricture is a serious late morbidity for patients after RT.
3.2 Biology (Molecular Mechanisms of RT-Induced Esophageal Injury)
Although the pathologic response of the esophagus to radiation injury has been characterized (Rubin and Casarett 1968), the molecular events responsible for the late effects of radiotherapy are complex and not resolved fully (Stone et al. 2003; Brush et al. 2007; Denham and Hauer-Jensen 2002). The response of normal tissue to RT involves a sequence of steps intended to promote healing, including inflammation, epithelial proliferation, collagen deposition, and remodeling (Denham and Hauer-Jensen 2002). When compared to wound healing after non-RT tissue damage, the injury response is often dysregulated after RT. Whereas the tissue-damage response functions to promote successful wound healing in response to other insults, this response contributes to chronic damage after RT. It is possible that cytokine-mediated damage in response to RT is due to changes in the microenvironment, the influence of cell death on nearby tissues, or DNA damage (Brush et al. 2007). Pro-inflammatory mediators, such as chemokines and cytokines, are expressed in tissues, including the esophagus after RT (Vujaskovic et al. 2007; Brush et al. 2007). The significance of oxidative stress and integrin αv6β-mediated stimulation of TGF-β is supported by preclinical work with amifostine in a rat model. Vujaskovic et al. showed that amifostine reduced acute and late pathologic changes after RT with associated decreases in oxidative stress and levels of integrinαv6β and TGF-β (Vujaskovic et al. 2007). It has been shown that manganese superoxide dismutase gene therapy can ameliorate acute and late esophageal injury via modulation of RT-induced elevation of inflammatory cytokines (Epperly et al. 2001). These animal model studies of radioprotective agents for esophageal injury emphasize the relevance of pro-inflammatory mechanisms in the development of late effects after irradiation of the esophagus. In summary, the late clinical effects of esophageal irradiation appear to be initiated by oxidative stress, mediated by cytokines, and guided by end-pathway damage to the muscle wall and submucosal thickening (Fig. 4).
3.3 Pathophysiology (The Radiation Response of the Esophagus)
3.3.1 Pathologic Response to Radiation Therapy
The acute effects of RT on the esophagus are related primarily to damage of the basal epithelial layer (Phillips and Ross 1974), and late effects are associated with RT-induced changes in the submucosa and muscular tissue of the esophageal wall (Northway et al. 1979; Fajardo et al. 2001). The pathologic changes observed during RT-induced acute esophagitis are similar to findings in acute dermatitis or mucositis (Rubin and Casarett 1968; Fajardo et al. 2001). During the course of therapy, RT limits the proliferation of the basal cell layer, with degenerative changes and failure of cellular renewal. Characteristic morphologic changes include epithelial swelling, focal necrosis of the basal cell layer, and nuclear hyperchromasia. The basal cell layer may be considered the target for acute RT-induced esophagitis, as this is the region of rapid mitosis and multiplication (Fajardo et al. 2001). Dilatation of capillaries is also observed early in the course, with erythema and increased interstitial edema. The destruction of the basal cell layer results in mucosal thinning or ulceration and may culminate in esophageal mucosal denudation (Rubin and Casarett 1968; Squier and Kremer 2001; Fajardo et al. 2001) (Fig. 5a, b and c).
Although regeneration of the esophageal mucosal epithelium starts during RT, this period may also include the start of progression toward fibrosis of the esophageal wall. During the subacute period after RT, subepithelial fibrosis may become apparent. The chronic period may be viewed as a continual progression of pathologic changes observed during the subacute period, with increased thickening of the esophageal wall (Rubin and Casarett 1968; Fajardo L-G 1982). Stricture is the most common delayed sequela of esophageal irradiation (Coia et al. 1995; Fajardo et al. 2001; Fajardo L-G 1982). Morphologic findings at the stricture site include severe submucosal fibrosis, atrophic epithelial layer, and telangiectatic vessels within the lamina propria (Fajardo et al. 2001). Esophageal ulcers may develop as a delayed toxicity after RT and are usually solitary, round lesions with well-defined, raised borders. Ulceration typically involves the lamina propria and/or submucosa, but the muscularis propria is occasionally eroded. Microscopically, late esophageal ulcers characteristically contain a base of necrotic tissue with acute granulation tissue underneath, as well as chronic granulation tissue below. Chronic ulceration is associated with extensive fibrosis, and esophageal ulcers are often thought to be due to RT-induced vascular insufficiency (Fajardo et al. 2001).
Esophageal motility disorders are a significant feature of late esophagitis. Dysmotility after RT has also been attributed to neuronal injury, based upon findings of manometry and dynamic isotope studies (Kaplinsky et al. 1991). However, morphologic evidence of neuronal damage is not commonly found in pathologic specimens after RT (Fajardo et al. 2001), thus suggesting an alternative mechanism. Nevertheless, it is possible that there are neuronal effects that without detectable abnormalities being seen on light microscopy (Fajardo et al. 2001). It has also been suggested that motility changes after RT may be related to muscularis propria damage (Seaman and Ackerman 1957).
3.3.2 Insights from Animal Models
Animal models of esophageal injury after RT have provided important clues to the pathogenesis of acute and chronic esophagitis, beginning in 1921 with the experiments by Lacassagne involving radium exposure of the rabbit esophagus (Lacassagne 1921). After a single, large-dose RT fraction, characteristic pathologic changes in the mucosa occur that correspond to acute effects (Engelstad 1934; Phillips and Ross 1974; Lacassagne 1921). Phillips et al., using a mouse model, observed vacuolization and absence of mitoses within the basal level and thinning of the squamous surface by the third day after RT, followed by areas of basal cell proliferation during the second week, and regeneration of the mucosal lining by the end of the third week (Phillips and Ross 1974). These findings have been confirmed by pathologic studies of acute esophagitis in humans (Seaman and Ackerman 1957; Mascarenhas et al. 1989). In a more recent study, designed to evaluate amifostine in a rat model of RT injury, Vujaskovic et al. administered a single fraction of 9 Gy and observed increased esophageal mucosal thickness within 5 days of irradiation. They reported decreased acute pathologic radiation response in rats receiving amifostine (Vujaskovic et al. 2007).
Animal models have also contributed to our understanding of late pathologic changes of the esophagus after RT. Because the opossum esophagus is comprised of a muscular wall with architecture similar to the human esophagus, this has been used as a model of late RT-induced pathologic change. After a single dose of 22.5 Gy to the opossum, Northway et al. reported necrosis of the muscularis propria and deep musculature, as well as the presence of inflammatory cells surrounding the mesenteric plexus (Northway et al. 1979). These findings have been confirmed in humans (Fajardo et al. 2001; Fajardo L-G 1982; Papazian et al. 1983) and implicate the muscular component of the esophagus as the relevant target for late esophageal injury after RT. Northway et al. also observed peristalsis abnormalities and impaired esophageal sphincter function in opossum 1–8 months after RT (Northway et al. 1979).
Michalowski and Hornsey demonstrated a dose/length effect for ulcerative esophagitis after RT, reporting that the mean effective dose for single-fraction RT decreased only slightly, from 24.5 to 22 Gy, when length of irradiated esophagus was doubled in a mouse model (Michalowski and Hornsey 1986). This weak volume effect suggests that the esophagus is a “parallel” organ. In a recent study of RT-induced esophageal injury in rodents, a single fraction of 9 Gy resulted in damage to the tunica muscularis, higher submucosal deposition of collagen, and increased presence of macrophages. These findings were associated with oxidative stress and elevation of TGF-β levels (Vujaskovic et al. 2007).
4 Clinical Syndromes (Endpoints)
For patients receiving RT for thoracic malignancies, acute esophagitis is a common treatment-related toxicity. After 2 weeks of daily standard irradiation to portals that encompass the esophagus, dysphagia, and odynophagia are commonly reported. Morbidity may be severe enough to create treatment interruptions due to consequential dehydration and weight loss. Rates of severe (≥ grade 3) acute esophagitis increased from 1 % with sequential chemotherapy and RT for NSCLC (Byhardt et al. 1998; Dillman et al. 1990) to 15–46 % with concurrent chemoradiation treatment strategies (Byhardt et al. 1998; Curran et al. 2000; Choy et al. 1998) (Fig. 6, Tables 1 and 2).
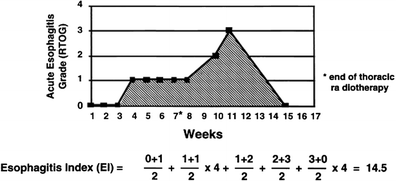
Table 1
Late effect of normal tissues for the esophagus. LENT SOMA
Grade 1
|
Grade 2
|
Grade 3
|
Grade 4
|
|
---|---|---|---|---|
Subjective
|
||||
Dysphagia
|
Difficulty eating solid foods
|
Difficulty eating soft foods
|
Can take liquids only
|
Totally unable to swallow
|
Pain
|
Occasional & minimal
|
Intermittent & tolerable
|
Persistent & intense
|
Refractory & excruciating
|
Objective
|
||||
Weight loss from time of treatment
|
≥5–10 %
|
>10–20 %
|
>20–30 %
|
>30 %
|
Stricture
|
>2/3 normal diameter with dilatation
|
>1/3–2/3 normal diameter with dilatation
|
<1/3 normal diameter
|
Complete obstruction
|
Ulceration
|
Superficial ≤1 cm2
|
Superficial >1 cm2
|
Deep ulcer
|
Perforation, fistulae
|
Bleeding (melena or hematemesis)
|
Occult
|
Occasional, normal Hb
|
Intermittent, 10–20 % decrease in Hb
|
Persistent, >20 % decrease in Hb
|
Anemia
|
Fatigue
|
Exhaustion
|
||
Management
|
||||
Dysphagia/Stricture
|
Diet modification or antacids
|
Diet modification and occasional dilatation
|
Temporary NG tube or regular dilatation
|
Parenteral feeding, prosthesis, gastrostomy or permanent NG tube
|
Weight loss
|
Diet modification
|
Nutritional supplements
|
Tube feeding
|
Surgical bypass, PEG
|
Pain/Ulceration
|
Occasional non-narcotic
|
Regular non-narcotic
|
Regular narcotic
|
Surgical intervention
|
Bleeding
|
Iron therapy
|
Occasional transfusion
|
Frequent transfusions
|
Surgical intervention
|
Analytic
|
||||
Barium esophagram
|
Assessment of esophageal lumen, stricture, dilatation
|
|||
Endoscopy
|
Assessment of esophageal lumen, mucosal integrity, ulceration
|
|||
CT
|
Assessment of esophageal wall thickness, lumen, stricture, dilatation
|
|||
MRI
|
Assessment of esophageal wall thickness, lumen, stricture, dilatation
|
|||
Ultrasonography
|
Assessment of esophageal wall thickness, lumen, stricture, dilatation
|
|||
Mobility esophagram
|
Assessment of motility of bolus and peristalsis
|
|||
Electromyogram
|
Assessment of motility of bolus and peristalsis
|
Table 2
Endpoints for late esophageal toxicity may be broadly divided into categories of subclinical versus clinical, and focal versus global, with corresponding examples as shown
Focal
|
Global
|
|
---|---|---|
Subclinical
|
1. Endoscopically detected mucosal changes (e.g., telangiectasias, ulcer, bleeding)
|
1. Asymptomatic dysmotility on swallowing study
|
2. CT-defined thickening
|
2. Weight loss
|
|
3. Stricture observed on endoscopy or swallowing study
|
||
Clinical
|
1. Bleeding/ulceration
|
1. Dysphagia
|
2. Stricture
|
2. Weight loss
|
Late esophagitis, which is less commonly observed than acute esophagitis, develops in <10 % of NSCLC patients receiving contemporary chemoradiotherapy (Byhardt et al. 1998). It is possible that late esophagitis will increase in frequency as hypofractionated RT becomes more prevalent for NSCLC treatment (Onimaru et al. 2003; Timmerman et al. 2006). Rates of late esophagitis are high among patients who receive large fractions of intraluminal brachytherapy in addition to external beam RT and chemotherapy, with a 12–17 % rate of fistulas and 24 % rate of strictures (Gaspar et al. 1997; Sharma et al. 2000). The late effects of RT on the esophagus generally manifest as dysphagia and odynophagia, which may be associated with stricture formation due to fibrosis of the muscular wall. Defects in esophageal motility are characteristic of late RT damage and may be observed on barium swallow (Lepke and Libshitz 1983; Goldstein et al. 1975), scintigraphy (Lamanna et al. 1985), or manometry (Coia et al. 1995; Kaplinsky et al. 1991). Strictures may develop 3 or more months after RT, with a median time of ≈6 months (O’Rourke et al. 1988). Barium swallow may demonstrate esophageal stricture, and endoscopy allows both visualization and potential for dilatation (Wax et al. 1997; Swaroop et al. 1994; Siersema 2008). In order to evaluate treatment-related esophagitis, several approaches have been utilized for standardized assessment of both acute and late findings.
The RTOG/EORTC developed the Subjective Objective Management and Analytic (SOMA) scale was published in 1995, as a product of the Late Effects of Normal Tissues (LENT) Conference, and provides a standard, consensus-based instrument for scoring late esophagitis (No Authors Listed 1995) (Table 1a and b).
The current version of the National Cancer Institute (NCI) Common Toxicity Criteria (CTC) for Adverse Events (AE), CTC version 4.0 (CTCAEv4.0), and the immediately prior version (CTCAEv3.0), differ from previous versions by relying more strongly upon patient symptoms. Prior criteria, including the RTOG scale and the CTCv2.0, were based more strongly upon the clinician’s assessment of the patient’s symptoms, dietary changes, analgesic requirement, weight loss, and need for IV fluids and/or non-oral nutritional supplementation. The CTCAE v4.0 criteria, which are designed for both acute and late effects can be found online. Late effects of esophageal RT may also be scored using the CTCAEv4.0 GI stricture criteria. The CTCAEv4.0 is currently considered the standard instrument for evaluating both acute and chronic esophagitis. The scale is meant to incorporate symptoms due to gastroesophageal reflux, but esophagitis symptoms attributable to infection (most commonly candidiasis) must be excluded when determining a score. Toxicity scores apply to only one point in time, without information regarding the duration of suffering. For patients receiving CRT for thoracic malignancies, symptoms of acute esophagitis develop after the second week of RT and increase to peak severity during the treatment course (Wei et al. 2006). Acute esophagitis symptoms commonly resolve within 2–3 weeks after RT, but late symptoms of esophageal damage may develop 3–8 months afterwards. The most common presentation of late esophagitis is solid food dysphagia due to focal esophageal stricture. In the study by Ahn et al., the median time to onset of late esophageal toxicity was 5 months (maximum, 40 months) (Ahn et al. 2005). In addition to stricture formation, deficits in esophageal motility are also observed frequently, with occurrence typically within 1–3 months after RT alone or within 1 week from the start of concurrent CRT (Coia et al. 1995; Goldstein et al. 1975).
It is challenging to score the severity of acute esophagitis as the severity of symptoms varies over time. Is 1 day of severe symptoms ‘better or worse’ than a week of moderate symptoms? The Esophagitis Index has been suggested as a reasonable manner to generate a single quantity (area under the curve) to reflect the severity and duration of symptoms (Fig. 6) (Werner-Wasik et al. 2000, 2002). The potential advantage of this approach is that it quantifies the degree of toxicity over time, but its calculation requires the collection of toxicity scores at specific points in time (Werner-Wasik et al. 2000).
A variety of endpoints that can be used to describe late esophageal injury are presented in Table 2.
4.1 Detection: Endoscopy
In the assessment of RT-induced esophagitis, endoscopy, and imaging studies provide important information. During the acute phase, endoscopic findings of esophagitis include mucosal erythema, erosion or ulceration (Mascarenhas et al. 1989; Hirota et al. 2001). Hirota et al. performed endoscopic examination during or soon after RT for patients with NSCLC, most treated with concurrent chemoradiotherapy, and found a good correlation between endoscopy-measured esophagitis grade and RTOG toxicity score (rank correlation coefficient = 0.428, p < 0.0001) (Hirota et al. 2001). Endoscopic findings of acute esophagitis from Hirota et al., along with the accompanying score, are displayed in Fig. 7a (Hirota et al. 2001). The agreement between endoscopic appearance of the esophagus during the acute phase and the reported RTOG toxicity score is supportive of the scoring system’s validity (Werner-Wasik et al. 2004). In some instances, however, endoscopic findings of esophagitis may not be confirmed by histology when biopsied (Mascarenhas et al. 1989), producing some false-positive results. Although endoscopy may be useful in the management of esophagitis, endoscopic findings are not a primary determinant of toxicity grade in standard practice. The current CTCAE criteria emphasize patient symptoms of esophagitis, but the description of CTCAEv4.0 Grade 1 esophagitis does include asymptomatic findings on endoscopy or radiography.
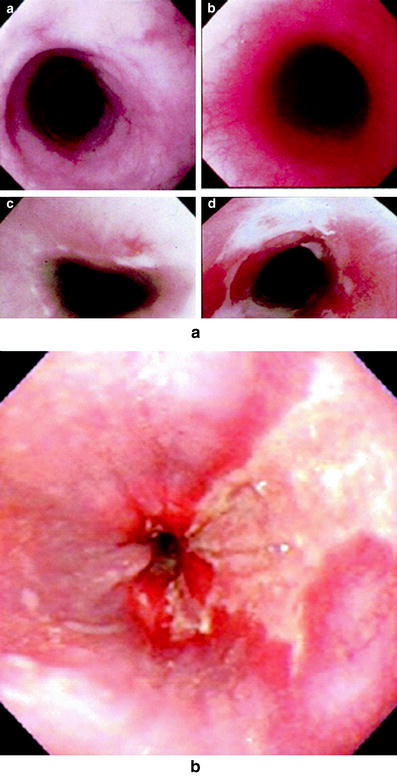
Fig. 7
Endoscopically assessed score used by Hirota et al. 2001 a Grade 0 for normal mucosa. b Grade 1 for mucosa with erythema. c Grade 2 for mucosa with erosion. d Grade 3 for mucosa with thickly-coated ulcer Adapted with permission. Endoscopic appearance of esophageal stricture as a late effect after RT With permission
Although most endoscopic findings of acute esophagitis resolve without the development of chronic effects, some damage that is evident during the acute period may progress to late esophagitis (Hirota et al. 2001). Endoscopy permits the visualization of strictures that occur as a late effect after RT (Fig. 7b) and provides an opportunity for dilatation by bougie or balloon, the standard therapeutic approach for post-RT benign esophageal stricture (Siersema 2008; Raymondi et al. 2008). Many strictures require more than one dilatation, with a reported median number of 2.5 dilatations delivered after a median time of 5 months between procedures (O’Rourke et al. 1988). The likelihood of requiring more than one dilatation for esophageal stricture is higher for complex versus simple strictures, which may be determined by endoscopy. Simple strictures are short, straight, and wide enough to permit passage of a standard-diameter endoscope. Longer (>2 cm), tortuous strictures, through which a standard endoscope may not be advanced, are categorized as complex and are a challenge for endoscopic visualization and dilatation (Siersema 2008; Giever et al. 2008; Lew and Kochman 2002). Endoscopic dilatation is discussed in further detail below in Sect. 8.2 along with other aspects of management of RT-related esophagitis.
4.2 Diagnosis: Imaging (Radiology)
Radiological studies may complement endoscopy and clinical evaluation in the assessment of esophagitis after RT. The esophagogram may be used to demonstrate esophageal stricture and dysmotility after RT (Lepke and Libshitz 1983; Goldstein et al. 1975; Ellenhorn et al. 1993). Goldstein et al. observed deficiencies in peristaltic waves on esophagograms in patients who received mediastinal RT (Goldstein et al. 1975). Double-contrast esophagograms may demonstrate the presence of multiple ulcers or granular mucosa as an acute effect of RT, as well as stricture as a late effect after RT, as shown in Fig. 8 (Collazzo et al. 1997). Manometry and technetium transit scintigraphy may also be used to demonstrate abnormal esophageal motility after RT (Kaplinsky et al. 1991; Lamanna et al. 1985). Findings on esophageal manometry after RT that support the diagnosis of esophagitis include low-amplitude, weakened or absent peristaltic contractions (Collazzo et al. 1997). Among 25 patients who received RT, Lamanna et al. observed protracted transit times using technetium transit scintigraphy in nearly all patients after more than 40 Gy, and some patients displayed transit abnormalities at >2 months after RT (Lamanna et al. 1985). During the initial evaluation of esophageal stricture after RT, esophagography may be helpful in defining the location and extent of stricture. Other modalities, such as manometry or scintigraphy, may provide complementary information regarding esophageal motility. In appropriate cases, endoscopy would then be performed, with biopsy or dilatation as appropriate. In summary, radiography can demonstrate RT-induced esophageal injury and complements endoscopy in the evaluation of acute and late esophagitis.
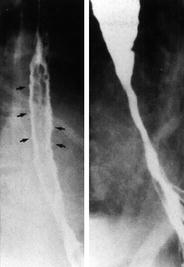
Fig. 8
Double-contrast esophagograms show the presence of multiple, small ulcers in the acute period (left) and the development of stricture at the site of radiation injury as a late effect (right). With permission (Collazzo et al. 1997)
5 Radiation Tolerance (Predicting Radiation-Induced Esophageal Injury)
Rates of late esophageal complications after RT have been associated with RT dose, with evidence of a relatively-steep dose–response curve (Morichau-Beauchant et al. 1983; Phillips and Margolis 1972). Analyses of associations between dosimetric parameters and late esophagitis have provided insight into dose-volume aspects of delayed esophageal injury after RT (Ahn et al. 2005; Qiao et al. 2005; Kahn et al. 2005; Maguire et al. 1999; Rose et al. 2008). In their seminal publication of estimated normal tissue dose limits, Rubin and Emami, Lymen, et al. suggested that the doses for whole-esophagus RT that result in a 5 and a 50 % rate of stricture or perforation at 5 years are 55 and 68 Gy, respectively, in standard fractionation (Emami et al. 1991). In the following section, we review factors associated with risk of radiation-induced esophageal injury (Fig. 9, Tables 3 and 4).
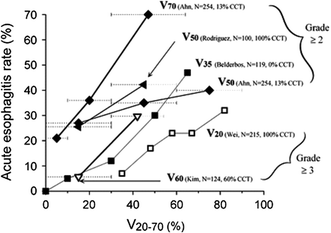
Fig. 9
The QUANTEC review analyzed the associations among dose-volume parameters and risk of acute toxicity. Incidence of acute esophagitis (y-axis) versus Vx (volume receiving more than x Gy). x-Axis values estimated according to range of doses reported. Each curve annotated as follows: Vdose (investigator, number of patients, percentage with concurrent chemotherapy (CCT). Dashed horizontal lines reflect dose ranges ascribed to each data point. Upper x-axis range of greatest data point for V50, are indefinite according to data (light-gray dotted bars). Solid and open symbols represent reported rates of Grade 2 or greater acute esophagitis and Grade 3 or greater acute esophagitis, respectively. Thicker and thinner solid lines represent higher and lower doses of Vx, respectively (i.e., thicker line for V70 and thinner line for V20) Reproduced with permission (Werner-Wasik et al. 2010)
Table 3
Normal tissue tolerance estimates for esophagus, proposed by Emami et al. based upon clinical experience and review of the literature. TD5/5 and TD50/5 represent the dose that results in 5 risk and 50 % risk, respectively, for the selected endpoint of clinical esophageal stricture or perforation at 5 years
Risk
|
Estimated tolerance doses for esophageal irradiation
|
Endpoint
|
||
---|---|---|---|---|
1/3 Volume
|
2/3 Volume
|
3/3 Volume
|
||
TD5/5
|
6000
|
5800
|
5500
|
Clinical stricture/perforation
|
TD50/5
|
7200
|
7000
|
6800
|
Clinical stricture/perforation
|
Table 4
Example dosimetric factors that have been associated with late esophagitis in the medical literature
Author
|
Years
|
N
|
Dosimetric parameter
|
---|---|---|---|
Maguire et al. (1999)
|
1999
|
91
|
Volume tx >50 Gy
|
Surface area tx >50 Gy
|
|||
Length of 100 % circumference tx >50 Gy
|
|||
Length of 100 % circumference tx >60 Gy
|
|||
Maximum % circumference tx >80 Gy
|
|||
Ahn et al. (2005)
|
2005
|
196
|
Length of 75 % circumference tx ≥70 Gy
|
Length of 100 % circumference tx ≥50 Gy
|
|||
Length of 100 % circumference tx ≥55 Gy
|
|||
Maximal percentage of circumference tx ≥70 Gy
|
|||
Kahn et al. (2005)
|
2005
|
236
|
Volume tx ≥60 Gy
|
Qiao et al. (2005)
|
2005
|
208
|
Mean esophageal dose ≥40 Gy
|
Maximal dose point ≥60 Gy
|
5.1 Dose Time Fractionation (Dosimetric Parameters)
Although threshold doses for RT-induced esophageal injury had been described earlier (Roswit 1974), Emami et al. provided the first dose-volume recommendations for esophagitis, using the endpoint of clinical stricture or perforation at 5 years, based upon the authors’ clinical experience and review of the literature (Emami et al. 1991) (Table 3). Subsequently, the widespread availability of three-dimensional RT planning has provided informative data for the prediction of esophagitis. The length of irradiated esophagus has been reported to impact esophagitis, as suggested by animal studies that demonstrated that increasing the length of irradiated esophagus lowers the mean effective dose for ulcerative esophagitis (Michalowski and Hornsey 1986). However, findings in clinical studies are contradictory and do not confirm the presence of such a relationship between length or irradiation esophagus and esophagitis in NSCLC patients (Ball et al. 1995; Werner-Wasik et al. 2000; Werner-Wasik et al. 2004; Choy et al. 1999; Langer 1999). Dosimetric parameters have been associated with both acute and late esophagitis in published reports, and are reviewed below (see Tables 3, 4 and 5).
Table 5
Summary of large published series investigating treatment-related esophagitis in patients with non-small cell lung cancer Reproduced with permission (Werner-Wasik et al. 2010)
Series Institution (Author, Year)
|
Patient number
|
Prescription dose range [Median]a in Gy (special fractionations)
|
% with CCT
|
Endpointb (rate)
|
Univariate significant factors
|
Multivariate significant factors:
|
---|---|---|---|---|---|---|
Duke (Maguire et al. 1999)
|
91
|
64-86 (Collazzo et al. 1997)d (64 % BID, 1.25−1.6 Gy/fx)
|
47
|
Acute grade ≥3 (Gr 3: 11 % Gr 4 and 5: 0 %)
|
None
|
None
|
Any late:d18 % (Gr 1: 9 % Gr 2: 6 % Gr 3: 3 %)
|
V50; A50; Length of 100 % circumference >50 Gy
|
Gender; pre-RT dysphagia; V50; maximum % of circumference >80 Gy
|
||||
Thomas Jefferson (Werner-Wasik et al. 2000)d
|
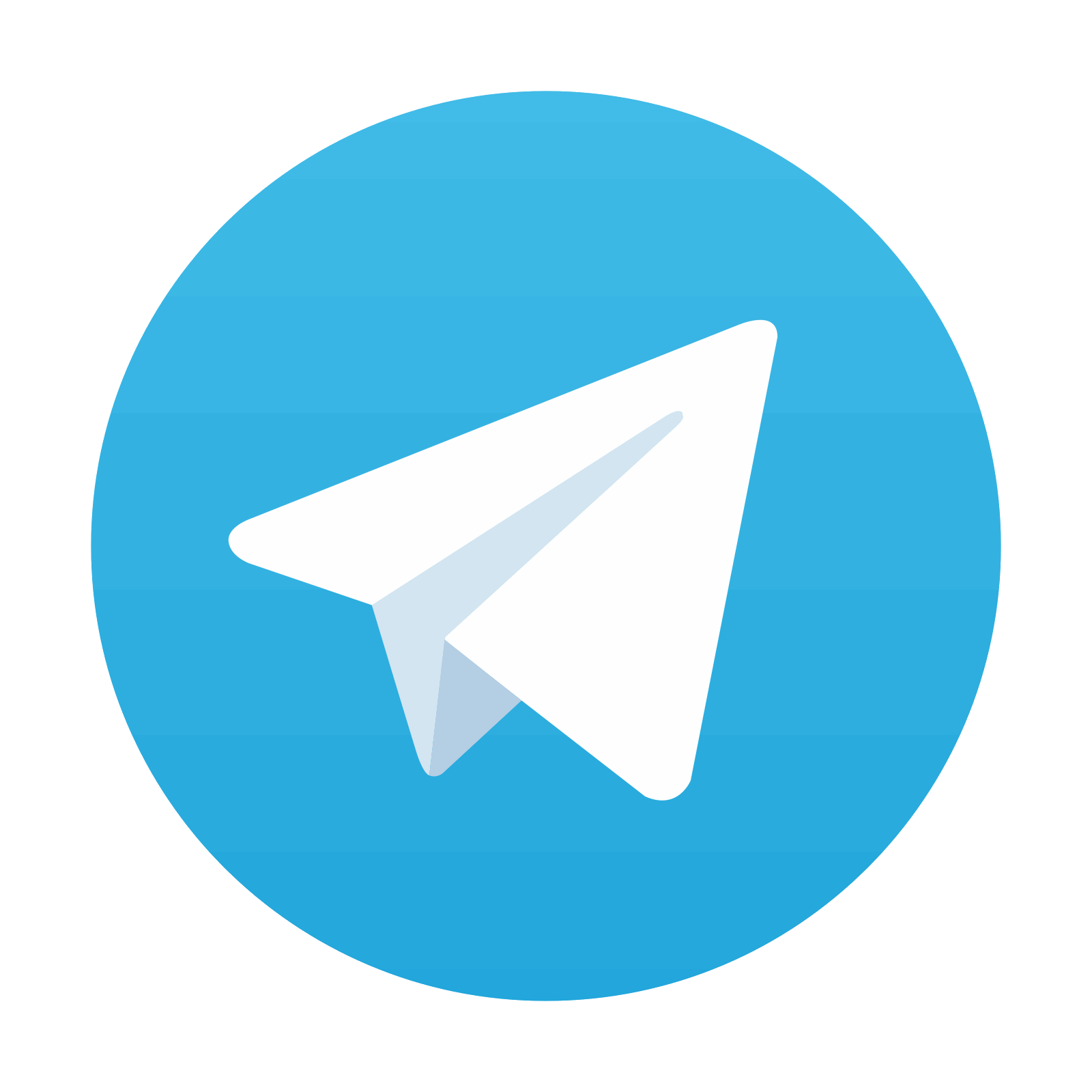
Stay updated, free articles. Join our Telegram channel

Full access? Get Clinical Tree
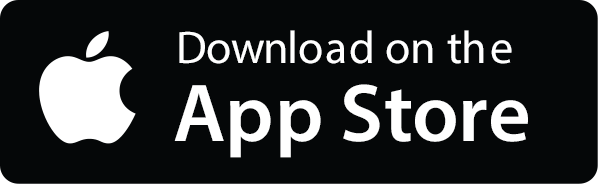
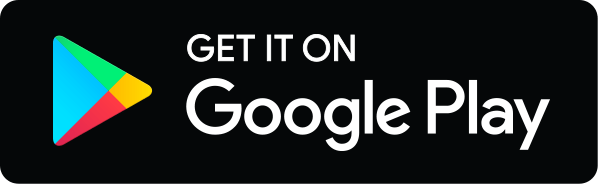