DW and DCE MR imaging contribute significantly to diagnosis, treatment planning, response assessment, and prognosis in personalized cancer medicine. Nevertheless, the need for further standardization of these techniques needs to be addressed. Whole-body DW MR imaging is an exciting field; however, future studies need to investigate in more depth the biologic significance of the findings depicted, their prognostic relevance, and cost-effectiveness in comparison with MDCT and PET/CT. New MR imaging probes, such as targeted or activatable contrast agents and dynamic nuclear hyperpolarization, show great promise to further improve the care of patients with cancer in the near future.
Key points
- •
Several functional MR imaging techniques are being used to detect biological processes in vivo, for example, to evaluate tissue organization with diffusion-weighted imaging, to assess tumor vascularity with dynamic contrast-enhanced MR imaging or tumor metabolites using magnetic resonance spectroscopy or dynamic nuclear polarization.
- •
The most important strength of functional MR imaging is its capacity for whole-body imaging, to capture whole-tumor heterogeneity in vivo, and the noninvasive assessment of changes over time.
- •
Standardization of these imaging techniques needs to be addressed in the future.
Introduction
The National Cancer Institute defines personalized cancer medicine as “a form of medicine that uses […] specific information about a person’s tumor to help diagnose, plan treatment, find out how well treatment is working, or make a prognosis.” Functional imaging allows visual analysis and quantification of biological processes in vivo, such as tumor metabolism, chemical composition, and blood flow. Its most important strength in comparison with other laboratory-based tests of tumor biology is its capacity for whole-body imaging, to capture whole-tumor heterogeneity in vivo, and the noninvasive assessment of (treatment-related) changes over time. Genetic tumor analysis based on single/few tumor biopsy samples may not reflect intratumoral heterogeneity and phenotypic diversity. A study in primary renal carcinoma and associated metastatic sites revealed that intratumoral heterogeneity can lead to underestimation of the tumor genomic landscape represented from single tumor biopsy samples and thus may contribute to treatment failure.
Multiple functional/molecular imaging technologies are available, with PET/computed tomography (PET/CT), single-photon emission computed tomography (SPECT), and MR imaging being represented in clinical routine. Because these modalities image different biologic processes, they have the potential to be used in conjunction rather than in competition with one another. Irrespective of their field of application, the advantages of MR imaging in comparison with PET and SPECT relate to its high spatial and temporal resolution, the superior soft tissue contrast, the capacity of multiparametric imaging, and the lack of ionizing radiation, which is relevant in vulnerable populations, such as children and women of child-bearing age, but might be less relevant in adult patients with cancer who undergo chemotherapy and/or radiation therapy.
Several functional MR imaging techniques are being used to, for example, evaluate tissue organization with diffusion-weighted imaging (DWI), to assess tumor vascularity with dynamic contrast-enhanced (DCE) MR imaging, and to detect tumor metabolites using magnetic resonance spectroscopy (MRS)/spectroscopic imaging (MRSI) or dynamic nuclear polarization (DNP). In addition, several specific and nonspecific MR contrast agents are clinically applicable or under investigation. On T2*-weighted imaging, hypoxia can be detected based on an increase in the transverse relaxation rate of water caused by the paramagnetic effect of endogenous deoxyhemoglobin using blood oxygen level–dependent (BOLD) MR. This technique has been used for imaging tumor hypoxia and treatment response.
Radiogenomics is another exiting field that aims to correlate cancer-imaging features and genetic data for the evaluation of imaging biomarkers. For example, imaging features extracted from MR imaging have been shown to be correlated with gene expression in breast cancer and glioblastoma. Texture analysis describes mathematical parameters computed from the distribution of pixels and is a noninvasive method of assessing heterogeneity within the tumor. Features derived by texture analysis have, for example, been shown to act as a potential imaging biomarker of tumoral response to neoadjuvant chemotherapy/radiation therapy in rectal cancer.
Introduction
The National Cancer Institute defines personalized cancer medicine as “a form of medicine that uses […] specific information about a person’s tumor to help diagnose, plan treatment, find out how well treatment is working, or make a prognosis.” Functional imaging allows visual analysis and quantification of biological processes in vivo, such as tumor metabolism, chemical composition, and blood flow. Its most important strength in comparison with other laboratory-based tests of tumor biology is its capacity for whole-body imaging, to capture whole-tumor heterogeneity in vivo, and the noninvasive assessment of (treatment-related) changes over time. Genetic tumor analysis based on single/few tumor biopsy samples may not reflect intratumoral heterogeneity and phenotypic diversity. A study in primary renal carcinoma and associated metastatic sites revealed that intratumoral heterogeneity can lead to underestimation of the tumor genomic landscape represented from single tumor biopsy samples and thus may contribute to treatment failure.
Multiple functional/molecular imaging technologies are available, with PET/computed tomography (PET/CT), single-photon emission computed tomography (SPECT), and MR imaging being represented in clinical routine. Because these modalities image different biologic processes, they have the potential to be used in conjunction rather than in competition with one another. Irrespective of their field of application, the advantages of MR imaging in comparison with PET and SPECT relate to its high spatial and temporal resolution, the superior soft tissue contrast, the capacity of multiparametric imaging, and the lack of ionizing radiation, which is relevant in vulnerable populations, such as children and women of child-bearing age, but might be less relevant in adult patients with cancer who undergo chemotherapy and/or radiation therapy.
Several functional MR imaging techniques are being used to, for example, evaluate tissue organization with diffusion-weighted imaging (DWI), to assess tumor vascularity with dynamic contrast-enhanced (DCE) MR imaging, and to detect tumor metabolites using magnetic resonance spectroscopy (MRS)/spectroscopic imaging (MRSI) or dynamic nuclear polarization (DNP). In addition, several specific and nonspecific MR contrast agents are clinically applicable or under investigation. On T2*-weighted imaging, hypoxia can be detected based on an increase in the transverse relaxation rate of water caused by the paramagnetic effect of endogenous deoxyhemoglobin using blood oxygen level–dependent (BOLD) MR. This technique has been used for imaging tumor hypoxia and treatment response.
Radiogenomics is another exiting field that aims to correlate cancer-imaging features and genetic data for the evaluation of imaging biomarkers. For example, imaging features extracted from MR imaging have been shown to be correlated with gene expression in breast cancer and glioblastoma. Texture analysis describes mathematical parameters computed from the distribution of pixels and is a noninvasive method of assessing heterogeneity within the tumor. Features derived by texture analysis have, for example, been shown to act as a potential imaging biomarker of tumoral response to neoadjuvant chemotherapy/radiation therapy in rectal cancer.
Diffusion-weighted imaging
DWI uses the incoherent 3-dimensional motion of water molecules in vivo (Brownian motion) to generate contrast. The degree of water diffusion within intracellular and extracellular fluid and between intracellular and extracellular compartments is impeded by tissue cellularity, intracellular elements, membranes, and macromolecules. The motion of water molecules, for example, in tumor tissue, cytotoxic edema, abscess, and fibrosis is more restricted and displays higher DWI signal intensity. The apparent diffusion coefficient (ADC) is a measure of the magnitude of diffusion and is lower in tissue with restricted diffusion compared with normal parenchyma ( Fig. 1 ). ADC is expressed in units of mm 2 /s.
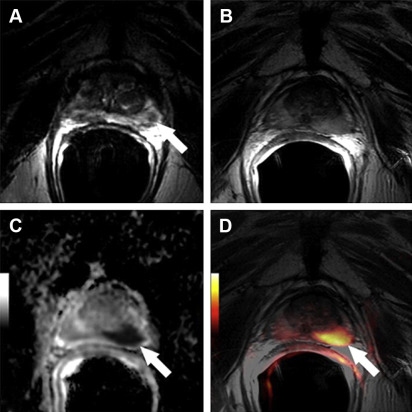
Diffusion-based contrast primarily depends on the selection of b-values (the degree of diffusion weighting that is applied during image acquisition), with improved contrast-to-noise ratio at higher b-values, at the expense of lower signal-to-noise ratios.
In general, malignant tumors exhibit higher DWI signal and lower ADC values compared with normal/reactive tissue or benign tumors. DWI has been shown to improve detection and diagnostic accuracy in several primary malignancies; for example, in prostate or endometrial cancer. DWI in conjunction with morphologic MR imaging sequences improves detection of metastatic spread to the peritoneal cavity, in particular in gynecologic malignancies with a reported sensitivity and specificity for the detection of peritoneal implants of 90.0% and 95.5%, respectively.
In several studies, lower ADC values have been associated with a more aggressive tumor. However, DWI signal intensity and ADC values are dependent on histologic characteristics, such as tumor type, tumor grade/differentiation, and extent of necrosis. False negatives may occur particularly in well-differentiated tumors, in cystic or necrotic lesions. Abscess and infection might cause false-positive findings.
Low pretreatment tumor ADC has been found to predict a favorable treatment response; for example, in colorectal and gastric carcinomas. This observation might be explained by the relationship between tumor necrosis and unfavorable patient outcomes.
Successful treatment is generally reflected by decreases in signal intensity on high b value images and corresponding increases in ADC values due to treatment-induced necrosis, edema, or cellular lysis; all of them induce an increase in water diffusion in the extracellular space. However, transient early decreases in ADC values can be seen after treatment.
The development of echoplanar imaging, high gradient amplitudes, multichannel coils, and parallel imaging facilitated DWI to be extended to whole-body imaging ( Fig. 2 ). Whole-body DW MR imaging is an exciting field to image systemic disease, such as multiple myeloma, lymphoma, and leukemia, but also solid tumors with associated metastatic spread, particularly those involving the skeleton. Whole-body DWI can provide complementary information to CT, PET/CT, and SPECT or might be able to replace tests using ionizing radiation. However, published data on staging/restaging accuracy and treatment response assessment are limited. Whole-body DWI for tumor staging has some limitations, especially with regard to the limited anatomic coverage for intravenous contrast-enhanced sequences. Some solutions have been proposed. For example, Klenk and colleagues used ferumoxytol (AMAG Pharmaceuticals, Inc, Waltham, MA) enhanced whole-body DWI for staging of children and young adults with malignant lymphoma and sarcoma in comparison with 18 F-fluorodeoxyglucose (FDG) PET/CT. Ferumoxytol increases the signal intensity on T1-weighted images and decreases the signal intensity on T2-weighted images (hereby improving the contrast between tumor and the reticuloendothelial system). The fusion of ferumoxytol-enhanced whole-body DWI scans with ferumoxytol-enhanced anatomic T1-weighted scans provided diagnostic images very similar to an 18 F-FDG PET/CT scan with equivalent sensitivities, specificities, and staging results of both imaging modalities.
Short-term and midterm test-retest variability of repeated ADC measurements in a healthy population has been reported to be not significant with a mean coefficient of variation of 14%. However, the investigators suggest that treatment effects of less than approximately 27% (1.96 × coefficient of variation) will not be meaningfully detectable. Interestingly, this definition of tumor response is very similar to what was reported in several studies investigating metabolic tumor response by 18 F-FDG PET and which was suggested as a cutoff in the recently introduced PET Response Criteria In Solid Tumors (PERCIST) criteria.
The lack of standardization and the limited published data on interscanner variability hinder the comparison of DWI results between studies. A recent prospective study evaluated the variability of ADC values in various anatomic regions in the upper abdomen measured with systems from different vendors and with different field strengths. The investigators found no significant differences between ADC values measured at 1.5 T and at 3.0 T in any anatomic region. However, in 2 of 7 regions at 1.5 T (left and right liver lobes) and in 4 of 7 regions at 3.0 T (left liver lobe, pancreas, and renal cortex and medulla), intervendor differences were significant.
Dynamic contrast-enhanced MR imaging and MR contrast agents
Perfusion Imaging
Extracellular paramagnetic gadolinium-based contrast agents (EGBCA) distribute nonspecifically in the blood plasma and interstitial space and are administered to reduce the T1 relaxation time of nearby protons, and therewith increase the signal intensity on T1-weighted images. In oncologic imaging, DCE MR imaging uses a bolus injection of EGBCA to acquire multiple serial images as the contrast agent passes through tissue to obtain information on altered blood flow and vascularization of tumors. The perfusion data extracted from DCE MR imaging can be investigated qualitatively (visual), in a semiquantitative or quantitative manner to obtain data on enhancement fraction and permeability, respectively. Most of the pharmacologic models used for the quantitative approach are based on determining the rate of contrast exchange between blood plasma and extracellular space using transfer rate constants, such as K trans (forward volume transfer constant) and k ep (reverse reflux rate constant between extracellular space and plasma).
The absence of enhancement is a strong predictor of benignity in several tumors, for example, in breast cancer, whereas the semiquantitative enhancement criterion that suggests malignancy is a rapid initial enhancement ( Fig. 3 ). Quantitative DCE MR imaging also allows differentiation of malignant from benign tumors, as has been shown for example, in adnexal masses. On the other hand, qualitative DCE MR imaging time curve type analysis was found to perform poorly for the differentiation of prostate cancer from healthy prostatic tissue. DCE is currently considered to add relatively little incremental value to the combination of T2-weighted and DWI for the detection of prostate cancer, as reflected in the recently updated Prostate Imaging and Reporting and Data Systems: Version 2 (PIRADS v2.0), which ascribed DCE a minor role in determining the PIRADS Assessment Category when T2-weighted and DWI are of diagnostic quality. The addition of DCE MR imaging to T2-weighted and DWI also did not contribute significant incremental value in the detection of locally recurrent prostate cancer after radiation therapy.
Anatomic tumor size measurements using standard World Health Organization, Response Evaluation Criteria In Solid Tumors (RECIST), and RECIST 1.1 criteria have limitations, particularly in assessing early treatment response and in assessing the effects of molecularly targeted therapies and antiangiogenic strategies that stabilize disease rather than induce fast tumor shrinkage. DCE MR imaging parameters can serve as predictive biomarkers and enable early treatment response assessment in patients who undergo treatment with antiangiogenic drugs and other therapies. However, the clinical application of the potentially powerful biomarkers derived from DCE MR imaging has been limited by the lack of standardization to permit interscanner/interinstitutional comparison of DCE MR imaging studies. Initiatives such as the Quantitative Imaging Biomarker Alliance will help to address these issues in the future.
Hepatobiliary Contrast Agents
Three hepatobiliary contrast agents (HBCAs) have been developed for liver MR imaging: gadoxetic acid (Gd-EOB-DTPA; Eovist [Bayer, USA], Primovist [Bayer, Germany]), gadobenate dimeglumine (Gd-BOPTA; MultiHance [Bracco, Italy]), and mangafodipir trisodium (Mn-DPDP; Teslascan [GE Healthcare, USA]; marketing status: discontinued). Gd-BOPTA and Gd-EOB-DTPA are taken up to varying degrees by functioning hepatocytes via organic anion transporters and are subsequently excreted in the bile ( Fig. 4 ). The relatively stronger hepatic signal intensity and biliary tree enhancement of Gd-EOB-DTPA in comparison with Gd-BOPTA results due to approximately 50% and 3% to 5% of excretion via the bile route, respectively. T1 shortening of the liver and biliary tree results in an increased difference in signal intensity for nonhepatocellular lesions compared with normal liver background. Therefore, HBCAs allow dynamic imaging in the arterial phase (20 s post injection [p.i.]), portal venous phase (60–70 s p.i.) and late venous phase (2–3 min p.i.), as well as liver-specific imaging with regard to a lesion’s hepatocyte function and hepatocyte content during the hepatobiliary phase (20 min p.i.). The results of several studies have shown that MR imaging with HBCA depicts more metastatic lesions in the liver than contrast-enhanced MR imaging with EGBCA and adds diagnostic information and confidence. Gd-BOPTA and Gd-EOB-DTPA have been shown to be equivalent to EGBCA dynamic imaging for lesion characterization. However, the relatively short bolus transit time due to the lower approved dose of Gd-EOB-DTPA (0.025 mmol/kg) in comparison with conventional EGBCA (1.0 mmol/kg) may result in weaker arterial enhancement of liver lesions and impaired lesion characterization. Therefore, the acquisition of the arterial phase needs specific attention and might benefit from modified injection strategies. In addition, acute self-limiting dyspnea was observed significantly more often using gadoxetate disodium compared with gadobenate dimeglumine and might affect arterial-phase MR image quality.
