Gastrointestinal and Correlative Abdominal Nuclear Medicine Imaging
M. Reza Habibian
MartinChirayu Shah
![]() |
Diverse radionuclide imaging procedures are available for studying the morphology and function of the gastrointestinal (GI) tract.
Although the details of anatomic alterations are demonstrable more reliably by conventional radiographic techniques, such as computed tomography (CT), ultrasound (US), and magnetic resonance imaging (MRI), radionuclide scintigraphy remains a unique noninvasive modality with quantitative capability for the evaluation of equally important functional disorders of the GI tract.
The recent development, acceptance, and integration of functional and structural images has resulted in redefining the role of scintigraphy in diagnosis of the various disorders of liver, GI tract, and abdominal organs.
Scintigraphic approaches using various radiopharmaceuticals can provide important complementary and supplementary information to CT, US, and MRI for further categorization of liver and other abdominal mass lesions, particularly in difficult clinical situations.
The technical aspects of nuclear medicine GI procedures are well described in standard textbooks; however, several of the currently available and clinically used procedures are reviewed briefly, as follows:
Gastric emptying scintigraphy
Gastrointestinal bleeding scintigraphy
Abdominal scintigraphy for bleeding of Meckel’s diverticulum
Hepatobiliary scintigraphy
Liver–spleen scintigraphy
Hepatic artery perfusion scintigraphy
Peritoneal venous shunt scintigraphy
Correlative abdominal nuclear medicine imaging
Hepatic hemangioma
Focal nodular hyperplasia
Hepatic adenoma
Focal fatty metamorphoses
Hepatoma
OncoScint immunoscintigraphy
Octreotide scintigraphy
MIBG imaging
Abdominal PET imaging
Scintigraphic detection of abdominal abscesses and inflammatory process
Salivary gland scintigraphy
Gastric Emptying Scintigraphy
Gastric emptying scintigraphy is a well-established procedure for the assessment of gastric motility disorder, sometimes called functional obstruction of the stomach.
“Functional obstruction” refers to an abnormality of gastric motility associated with the stomach’s inability to grind solid food into the small particle size necessary for emptying or the stomach’s inadequate contractility to generate enough GI pressure gradient to propel the gastric contents across the pylorus into the duodenum.
The grinding process occurs in the stomach’s antrum, which acts as a grinder to triturate the ingested food through rhythmic contraction at the rate of 30 cycles per minute.
The emptying pattern for solid food is biphasic, initiated by lag phase and followed by continuous emptying, usually in a linear fashion. The lag phase is the time required for the antrum to grind food.
The conventional protocol for gastric emptying scintigraphy involves measuring the rate of transit of radiolabeled liquid or solid food (commonly 300 to 500 μCi of 99mTc sulfur colloid in scrambled eggs in a volume of approximately 125 mL) through the stomach.
Using a modern dual-head camera system, a series of 1-minute dynamic images is obtained in the anterior and posterior projections for at least 90 minutes (the use of infrequent static images rather than continuous dynamic images is discouraged).
The entire 90-minute gastric emptying data can be displayed as a time–activity curve using geometric mean counts from the anterior and posterior projections. The geometric mean is the square root of the product of anterior and posterior projection counts. The curve is corrected for decay; otherwise gastric emptying would be overestimated.
Several quantitative data can be approximated from the time–activity curve:
The time it takes for half of the meal to leave the stomach, or the half-time (T1/2). Using the published data, the T1/2 of 60 ± 30 minutes for solid food and 30 ± 15 for the liquid phase is considered to be within normal limits. It should be noted that the normal value for the T1/2 varies depending on the content of the meal, the patient’s position, the protocol used, and the method by which the T1/2 is calculated. Also, it should be noted that although the T1/2 for emptying is often used clinically, the emptying process is not exponential; instead, it is continuous and linear. Therefore, in a physiologic sense, the use of “T1/2” may not be correct.
The percent of gastric emptying at 90 minutes [(maximum count – minimum counts)/(maximum counts × 100)], which takes into consideration the physiologic parameters of both the lag phase and the rate of linear emptying. A value of <35% is considered abnormal.
The length of the lag phase is approximated by visual inspection of the image as the time before the appearance of radiolabeled meals in the proximal small bowel or time when linear emptying is established on the time–activity curve. The duration of the lag phase may vary; however, a delay of more than 45 minutes is considered abnormal and indicates a grinding-phase abnormality.
The percent of the radiolabeled meal remaining in the stomach at 90 minutes. Retention of greater than 50% represents a delay in gastric emptying.
Gastrointestinal Bleeding
Scintigraphy of the abdominal blood pool following IV administration of a patient’s red blood cells (RBCs) tagged with
technetium-99m (99mTc)-pertechnetate is a well-established diagnostic procedure that is complementary to endoscopy and angiography for the detection of GI bleeding.
technetium-99m (99mTc)-pertechnetate is a well-established diagnostic procedure that is complementary to endoscopy and angiography for the detection of GI bleeding.
The procedure is well tolerated and easy to perform. The images provide a high sensitivity for the detection of a low bleeding rate and allow continuous monitoring of the GI tract for several hours. The detection and localization of bleeding sites has been improved significantly by the adoption of the cine scintigraphic technique and use of the improved in vitro labeling procedure, which results in greater than 98% labeling efficiency.
Bleeding at the rate of 0.40 mL/min is easily detected as a focus of intense activity outside the vascular blood pool. Although contrast angiography provides exquisite anatomic detail, bleeding at a rate greater than 1 mL/min must be present at the time of contrast injection; therefore scintigraphy may be useful in many circumstances, particularly in view of the inherently intermittent nature of GI bleeding.
Abdominal Scintigraphy for Bleeding Of Meckel’S Diverticulum
Abdominal scintigraphy with 99mTc-pertechnetate is the technique of choice for detecting ectopic gastric mucosa as the cause of GI bleeding. The most common site of ectopic mucosa is in a Meckel’s diverticulum.
99mTc-pertechnetate is taken up predominantly by the mucin-producing cells of the gastric mucosa, which allows for visualization not only of gastric but also heterotopic gastric mucosa.
Visualization of Meckel’s diverticulum usually occurs as a focus of intense activity in the right lower quadrant, approximately 20 minutes after the injection of 99mTc-pertechnetate, in concert with gastric visualization with same intensity of uptake.
Although the detection of a Meckel’s diverticulum by 99mTc-pertechnetate depends on the presence of gastric mucosa, occurring in approximately 30% of cases, GI bleeding from a Meckel’s diverticulum also requires the presence of gastric mucosa.
Hepatobiliary Scintigraphy
Hepatobiliary scintigraphy or cholescintigraphy, with or without pharmacologic intervention using cholecystokinin (CCK) and morphine, is used primarily for the assessment of diseases affecting the gallbladder, cystic duct, common bile duct, and sphincter of Oddi.
Diagnosis of acute cholecystitis by far is the most common clinical indication for cholescintigraphy.
A dose of 2 to 6 mCi of 99mTc-iminodiacetic acid (IDA) derivative is injected IV and serial images of the abdomen are obtained for 1 hour at 10-minute intervals.
Normal examination exhibits homogeneous liver uptake with uniform washout, resulting in visualization of the gallbladder and bowel within 30 to 60 minutes.
A gallbladder not seen by 60 minutes and not visualized by 20 to 30 minutes after IV injection of 0.05 mg/kg morphine strongly suggests acute cholecystitis.
Postcholecystectomy complications such as bile leak, cystic duct remnant, biliary ductal strictures, and stenosis are among other clinical indications for cholescintigraphy. Hepatobiliary images can be used to differentiate biliary atresia from neonatal hepatitis. Hepatobiliary images are also of value in the evaluation of complications associated with liver transplantation.
As a noninvasive procedure, cholescintigraphy is used for the evaluation of functional hepatobiliary disorder. Chronic acalculous gallbladder and biliary disease as a functional abnormality is diagnosed by the lack of response to CCK as measured by gallbladder ejection fraction in an otherwise normally visualized gallbladder and no abnormality on ultrasound.
The gallbladder ejection fraction is determined by IV infusion of 0.02 μg/kg CCK over a period of 30 minutes. Although a gallbladder normally ejects more than 80% of its contents in response to CCK, an ejection fraction that does not exceed 35% at 20 to 30 minutes postintervention is considered abnormal and represents biliary tract dyskinesis.
It is worth remembering that opioids reduce the gallbladder ejection fraction. Exclusion of opium intake is therefore important in order to avoid a false-positive study.
Liver-Spleen Scintigraphy
Liver–spleen scan using 99mTc sulfur colloid is a simple procedure that is useful in detecting space-occupying lesions and evaluating diffuse liver disease.
Following the intravenous injection of 99mTc–sulfur colloid, the colloid particles are phagocytized by reticuloendothelial cells normally distributed in the liver (Kupffer cells, 85%), spleen (10%), and bone marrow (5%). The usual dose is 2 to 6 mCi.
A normal scan shows homogeneous distribution of radioactivity within a normal-sized liver and spleen with normal contour. The liver has relatively more uptake than the spleen.
Performance of a dynamic study as part of the liver–spleen scan procedure may provide additional information of great value for further definition of liver lesions and may reveal other intra-abdominal abnormalities, such as aortic aneurysm, cystic lesions, collateral circulation, and superior vena cava obstruction. Although the liver blood flow study cannot separate a benign from a malignant liver lesion detected on static images, it can help in identifying the vascular nature of the focal defect.
Space-occupying lesions in the liver or spleen are indicated as areas of reduced or absent radioactivity on the 99mTc–sulfur colloid scan. Space-occupying lesions in the liver may be single, multiple, or even diffuse in distribution. The etiologic origins vary from congenital (cyst), infectious (abscess), trauma (laceration, radiation), degenerative (cirrhosis), benign neoplasm (adenoma, cavernous hemangioma), and malignant neoplasms (primary hepatoma or secondary metastasis).
Contrast-enhanced CT is superior to scintigraphy for the detection of metastatic liver disease with perhaps the exception of endocrine, colon, and ovarian metastasis.
Hepatic Artery Perfusion Scintigraphy
Intra-arterial infusion of chemotherapeutic agents through a catheter placed in the hepatic artery for treatment of hepatic tumor may be more effective than systemic IV chemotherapy because a higher dose of agent can be delivered directly to the tumor with a low incidence of systemic toxicity. A misplaced arterial catheter or a catheter tip displaced from the hepatic artery is not an uncommon occurrence and may be seen in 15% to 40% of cases. Infusion of the chemotherapeutic agent through such a catheter results in visceral misperfusion, with the potential for serious clinical toxicity due to inadvertent perfusion of other organs with a high dose of chemotherapy.
Hepatic arterial perfusion scintigraphy with 99mTc–macroaggregated albumin (MAA) is used to predict the distribution of a subsequently administered chemotherapeutic agent within the liver.
A dose of 1 to 2 mCi of 99mTc-MAA in a volume of 0.2 to 0.5 mL is injected at a slow rate into the catheter. The distribution of MAA particles reflects hepatic artery blood flow to the tumor and liver. Also, any area of undesired extrahepatic perfusion—such as stomach, pancreas, or bowel—may be detected, necessitating readjustment of catheter placement.
Hepatic artery perfusion scintigraphy may also be used to detect liver metastases in areas of the liver that appear normal by CT. Liver metastases, even avascular ones, are perfused from the hepatic artery; therefore a metastasis may appear as focally increased uptake. A focal defect seen by 99mTc–sulfur colloid imaging that shows hyperperfusion by hepatic artery perfusion scan is considered to be indicative of a metastatic lesion.
Peritoneal Venous (Laveen) Shunt Scintigraphy
Insertion of a LaVeen shunt has been shown to be useful in controlling the accumulation of ascites in patients with cirrhosis. The shunt operates by a one-way pressure-activated valve, which helps to reduce ascites by recirculating the ascitic fluid. However, it has a high incidence of spontaneous blockage.
The patency of a peritoneal venous shunt can be studied by injecting 3 to 5 mCi of 99mTc–MAA directly into the fluid accumulated in the abdominal cavity. The appearance of radioactivity in the lung field represents trapping of MAA particles in the precapillary bed of the lung, indicating shunt patency. Lack of lung activity would be conclusive for a nonfunctional (obstructed) shunt.
Correlative Abdominal Nuclear Medicine Imaging Scintigraphy
Hepatic Hemangioma
Cavernous hemangioma is the most common benign tumor of the liver and is found about 20% of the time in autopsy series. Histologically, the mass consists of vascular channels enclosed within the fibroelastic stroma.
99mTc–tagged RBC hepatic blood pool imaging is extremely useful in the identification of hepatic hemangioma.
The classic finding of hepatic hemangioma is represented by decreased perfusion and increased blood pool (perfusion–blood pool mismatch) activity in suspected areas of the liver.
A flow study is performed following IV injection of the patient’s RBCs labeled in vitro with 99mTc-pertechnetate. This is followed by serial planar images looking for a hypoperfused area that progressively accumulates RBCs. The specificity of 99mTc–tagged RBC scintigraphy for the diagnosis of cavernous hemangioma is extremely high (approximately 100%), but its sensitivity is high only when the lesion is larger than 2.5 to 3.0 cm. Using single photon emission computed tomography (SPECT), the sensitivity remains high for lesions smaller than 2 cm in diameter. SPECT is superior for detecting hemangiomas that are small, multiple, or adjacent to large vessels, heart, spleen, or the renal blood pool.
Focal Nodular Hyperplasia (FNH)
FNH is a benign hepatic mass and is usually an incidental finding. It is the second most common benign mass after hemangioma and occurs in approximately 3% of the population. Histologically the mass is a hypertrophic nodule consisting of Kupffer cells, hepatocytes, and disorganized bile ducts.
Although practically any space-occupying lesion within the liver appears as a cold area on 99mTc–sulfur colloid scan, a focal nodular hyperplasia, due to the hyperplastic nature of the mass containing all the liver elements, may appear hotter than the surrounding liver tissue. However, in many cases (∼ 70%), the FNH shows uptake equal to that of the liver. On hepatobiliary imaging, the FNH containing hepatocytes and biliary canaliculi takes up the agent and appears as a normal scan or as an area of focal increased uptake.
FNH can have a higher uptake of fluorine-18 (18F)-FDG than the surrounding liver tissue; as such, it may be mistaken for a hypermetabolic focus of metastasis, particularly in patients with a known primary cancer. However, this is a very rare occurrence; as a rule, FNH is not an FDG-avid lesion.
Hepatic Adenoma
Hepatic adenoma typically occurs in women using oral contraceptives or hormone therapy; it is usually asymptomatic. However, it may rupture or bleed, causing right-upper-quadrant pain. Rarely, rupture may lead to hemorrhagic shock. On histologic examination, hepatic adenoma appears to be composed of monoclonal hepatocytes devoid of bile duct and Kupffer cells.
Hepatic adenoma usually appears photopenic on 99mTc–sulfur colloid scan. However, it may be isodense, but never hotter than the liver. Hepatic adenoma, having no biliary radicals, also appears photopenic on IDA hepatobiliary images.
Focal Fatty Metamorphosis
Focal fatty metamorphosis (FFM) of the liver is a reversible disorder that may be seen in obese patients, diabetics, users of steroids, or alcoholics. FFM may be detected as a vague focal
mass on ultrasound or CT. Differentiation from hepatoma or metastatic lesions may be difficult by anatomic studies. Focal fatty metamorphosis in the liver contains Kupffer cells in the perisinoidal space; therefore any area that appears as a focal mass on CT or ultrasound but appears normal or as an area of slightly nonuniform uptake of 99mTc–sulfur colloid most likely represents a minimally infiltrated fatty liver and not malignancy. However, biopsy may be needed if the diagnosis is still in doubt.
mass on ultrasound or CT. Differentiation from hepatoma or metastatic lesions may be difficult by anatomic studies. Focal fatty metamorphosis in the liver contains Kupffer cells in the perisinoidal space; therefore any area that appears as a focal mass on CT or ultrasound but appears normal or as an area of slightly nonuniform uptake of 99mTc–sulfur colloid most likely represents a minimally infiltrated fatty liver and not malignancy. However, biopsy may be needed if the diagnosis is still in doubt.
Hepatoma
Hepatocellular carcinoma is usually diagnosed by anatomic study, and there is no need for the functional images. However, gallium-67 (67Ga)-SPECT imaging may be used in the evaluation of an indeterminate liver mass suspected for hepatoma, particularly in a patient with an elevated alpha-fetoprotein level. Typically, a hepatoma concentrates 67Ga and can be detected with a sensitivity of 70% to 90%. However, uptake is not specific, as other lesions—such as lymphoma, metastatic melanoma, infection, and even macroregenerating nodule of cirrhosis—may take up 67Ga.
OncoScint Immunoscintigraphy
OncoScint, a radiolabeled monoclonal antibody, may outperform contrast-enhanced CT in the detection of colon and ovarian cancer metastasized outside the liver (node). The positive predictive value for detection of colon and ovarian cancer is high (83% and 90%, respectively); however, a negative scan does not exclude malignancy.
Available OncoScint is an indium-111 (111In) radiolabeled monoclonal antibody directed against the antigen found in ovarian and colon cancer. A patient with elevated carcinoembryonic antigen (CEA) and a previous history of ovarian or colorectal cancer is a candidate for OncoScint scintigraphy.
The usual dose is 1 mg of antibody labeled with 5 mCi 111In for intravenous administration. A whole-body scintigram is usually acquired at 48 to 72 hours. Interpretation of an OncoScint scan requires close correlation with CT or MRI images.
Normal distribution of OncoScint includes liver, spleen, bone marrow, blood pool, and genitalia. Nonspecific uptake may be seen in the kidneys, bladder, breasts, nipples, and bowel. Further discussion of immunoscintigraphy may be found in Chapter 8.
Octreotide Scintigraphy
Octreotide, a small peptide of eight amino acids, is an analog of the endogenously secreted neuropeptide hormone somatostatin.
Somatostatin is found in various tumors and nontumoral tissue, with the highest concentration in the central nervous system, endocrine pancreas, and GI tract.
111In-labeled octreotide (Octreoscan) can be used to visualize any tumor bearing the somatostatin receptors. The list includes pituitary tumor, endocrine pancreatic tumor (gastrinoma, insulinoma, and glucagonoma), carcinoid, paraganglioma, medullary thyroid carcinoma, and Merkel cell carcinoma of the skin. Other disorders—such as pheochromocytoma, neuroblastoma, small cell lung cancer, breast cancer, malignant lymphoma, even granulomatous disease and Graves’ disease—may be visualized by Octreoscan. Nevertheless, Octreoscan is most effective in the detection and evaluation of carcinoid, islet cell tumor of the pancreas, and abdominal metastatic disease from small cell carcinoma of the lung.
The administered dose of 111In-octreotide is 6 mCi. Whole-body scan is acquired at 4 and 24 hours with optional 24-hour SPECT images. Whole-body scanning is especially useful for the detection of metastases or multiple tumors that may be seen in patients with paraganglioma or multiple endocrine neoplasms.
The normal distribution of Octreoscan includes liver, spleen, kidneys, and bladder. Images provide specific functional information; however, scintigram images complement rather than compete with anatomic studies such as CT or MRI.
The result of an Octreoscan can be used to select patients with neuroendocrine tumors who may benefit from Octreotide treatment.
MIBG (Meta-Iodo-Benzyl-Guanidine) Imaging
Radiolabeled MIBG has been used successfully for visualizing benign and malignant pheochromocytoma, adrenal medullary hyperplasia, and other tumors such as carcinoid, neuroblastoma, and paravertebral paraganglioma.
MIBG performs better than octreotide for differentiating pheochromocytoma and abdominal metastases from medullary thyroid carcinoma.
MIBG is a small molecule that is an analog of guanethidine. It resembles norepinephrine structurally and is taken up by adrenergic tissue. Many medications interfere with MIBG uptake, including tricyclic antidepressants, over-the-counter cold medications (containing phenylephrine, pseudoephedrine, and ephedrine), certain antipsychotics, and cocaine among others. The tumor uptake is not inhibited by adrenergic blocking agents other than labetalol.
Normal distribution of MIBG following IV injection includes the salivary gland, liver, spleen, and urinary bladder. Organs that are visualized less frequently and less intensely include myocardium, kidneys, and normal adrenal glands. Thyroid is not typically visualized as it is blocked by necessary pretreatment with saturated solution of potassium iodine (SSKI).
MIBG image is generally obtained at 48 to 72 hours following IV injection of 1 mCi of iodine-131 (131I)-MIBG or at 24 hours after injection of 10 mCi 123I-MIBG. 131I-MIBG has also been employed as a therapeutic agent for some endocrine tumors. MIBG imaging is reviewed in more detail, along with case presentations, in Chapters 1 and 8.
Abdominal PET Imaging
Oncologic positron emission tomography (PET) imaging and combined PET-CT imaging are the most recent advancements in nuclear medicine. PET imaging typically is a survey covering the neck through the pelvis. When it is combined with CT, the preselected regional anatomy becomes the focus of evaluation.
In oncologic PET imaging, 18F-labeled flurodeoxyglucose (18F-FDG) is the most commonly used radiopharmaceutical at present. The rationale behind the use of 18F-FDG for the imaging of malignant tissue is based on the observation that
malignant cells have an elevated rate of glycolytic activity; therefore they exhibit an increased uptake of glucose relative to the normal surrounding cells.
malignant cells have an elevated rate of glycolytic activity; therefore they exhibit an increased uptake of glucose relative to the normal surrounding cells.
18F-FDG has a half-life of 110 minutes. The usual dose is approximately 10 mCi, which is injected intravenously 1 hour prior to scanning.
Evaluation of the abdomen on PET CT requires a thorough familiarity with the normal distribution of 18F-FDG. Physiologic uptake is seen in the kidneys, urinary collecting system, bowel, and stomach wall. Colonic activity, particularly in the cecum and rectosigmoid, may be intense. Liver uptake is moderate, and the spleen has less uptake than the liver. Skeletal muscles may also demonstrate intense uptake if they were being used during the uptake. Physiologic brown fat uptake may also be seen in paraspinal and perirenal regions. Physiologic ovarian uptake in premenopausal women should not be confused with malignancy.
18F-FDG PET has been used successfully in the assessment of colorectal cancer, metastatic disease of the liver, and evaluation of ovarian cancer (see Chapter 8).
Scintigraphic Detection of Abdominal Abscesses
Abdominal abscesses may be detected by abdominal scintigraphy using WBCs labeled with indium-111 (111In-WBC), 99mTc-hexamethylpropyleneamine oxime (HMPAO), or a monoclonal antigranulocyte antibody labeled with 99mTc-pertechnetate. Utilization of 67Ga-citrate is limited in the evaluation of abdominal abscesses owing to its normal excretory bowel pathway, which may obscure an infected area within the abdominal cavity.
The scintigraphic technique can be supplementary or complementary to CT or ultrasound. Differentiating infected from noninfected abdominal fluid collections is not possible using anatomically oriented modalities, but WBC scintigraphy would be expected to be positive only in the infected sites.
The 111In-WBC scan images are usually obtained 24 hours after reinjection of the patient’s WBCs labeled with approximately 500 to 700 μCi of 111In-oxime. For evaluation of inflammatory bowel disease, an early image at 1 and 4 hours is necessary. Whole-body images are important, particularly when localizing signs of abdominal abscess are not present. The scan may show the source of infection to be outside the abdomen. Even when abdominal abscess is present, whole-body scintigraphy may reveal extra-abdominal foci.
A normal scan demonstrates distribution of radioactivity in the liver, spleen, and bone marrow (reticuloendothelial system). Activity outside of these compartments is abnormal or requires a physiologic or technical explanation. A sensitivity of 85% to 95% for the detection of infection and inflammatory processes has been reported for 111In-labeled–WBC scintigraphy. Controversy exists as to the effects of antibiotic therapy and chronicity of infection on the sensitivity of this technique.
HMPAO is a lipophilic complex that can penetrate the membranes of WBCs and permit labeling with 99mTc. The distribution of HMPAO-labeled WBCs is similar to that of 111In-labeled WBCs except that there is significant excretion of activity into the GI and genitourinary tracts.
In imaging with 99mTc-HMPAO labeling, bowel activity does not appear until 2 hours after the reinjection of labeled WBCs; therefore whole-body scanning should be performed at 1 hour and followed by another set of images at 4 hours.
99mTc-HMPAO-labeled–WBC images are useful, particularly in the diagnosis of inflammatory bowel disease. Activity rapidly localizes in the involved bowel within 1 hour and provides good-quality images. Segmental involvement of the bowel, skipped areas, and small bowel versus large bowel involvement may easily be demonstrated, often allowing differentiation of Crohn’s disease from ulcerative colitis, and can accurately determine the extent of the disease.
Monoclonal antigranulocyte antibody–labeled 99mTc-pertechnetate (NeutroSpec) has been used for the early diagnosis of appendicitis. In this procedure, 15 mCi of radiopharmaceutical is injected intravenously, which binds to the surface antigen of granulocytes. Activity is distributed in the blood pool, reticuloendothelial system, and urinary excretory organs. The scan done at 2 hours shows liver, spleen, kidney, and bone marrow activity. A positive scan is usually seen within 30 to 60 minutes. It should be noted that Netruspect is currently not available, as its use was suspended by the FDA because of safety concerns.
Salivary Gland Scintigraphy
Radionuclide scanning with 99mTc-pertechnetate is a simple, noninvasive method for the evaluation of salivary gland function.
The parotid gland is imaged following IV injection of 10 to 15 mCi of 99mTc-pertechnetate. Serial images are obtained in the anterior and both lateral projections within the first 20 minutes. The scan is followed by a washout image after oral stimulation with lemon juice.
The scan is evaluated for symmetrical gland accumulation, drainage, and areas of increased or decreased uptake that may suggest neoplasm, inflammation, or avascular lesions.
A washout image is very important in the detection of Warthin’s tumor, which does not communicate with the ductal system and therefore cannot excrete its accumulated activity even after lemon juice, resulting in a focus of persistently increased activity.
Case 5.1
History: A 71-year-old man with anorexia, early satiety, and a history of poorly controlled diabetes is referred for a gastric emptying study in order to evaluate the possibility of gastric outlet obstruction or diabetic gastroparesis.
A solid food gastric emptying study was performed following the ingestion of a sample food of scrambled eggs containing 400 μCi of 99mTc–sulfur colloid (Fig. 5.1).
Findings: Figure 5.1 displays the gastric emptying curve over a period of 90 minutes generated from geometric mean counts obtained from the anterior and posterior images outlining the stomach as an area of interest.
There is delayed gastric emptying with prolongation of the gastric emptying T1/2 exceeding 90 minutes, with 58.8% of radiolabeled meal remaining in the stomach at 90 minutes. There is an apparent 45-minute lag phase before the curve assumes a linear emptying pattern (arrow).
Discussion: Gastric emptying of solid foods, as depicted in Figure 5.1, is a biphasic pattern that initiates with a lag phase and is followed by a continuous linear pattern of emptying.
Delay in gastric emptying may be due to gastric outlet obstruction—commonly seen in patients with chronic duodenal ulcer, carcinoma of the stomach, gastric polyps, and hypertrophic pyloric stenosis—which may be associated with narrowing of the gastric lumen. These disorders should be studied and excluded by contrast radiographic examination or upper GI endoscopy.
Delay in gastric emptying may be functional, and etiologies vary from diabetes, vagotomy, collagen vascular disease, hypothyroidism, celiac disease, progressive systemic sclerosis, brain tumor, electrolyte abnormalities, and effect of medications. Delayed gastric emptying of functional origin, as measured by radionuclide gastric emptying and depicted on the time–activity curve, usually reflects the combination of prolongation of both the lag phase and continuous linear emptying process. However, delayed gastric emptying may be solely due to a prolonged lag phase, as seen in certain diseases such as diabetic gastroenteropathy and morbid obesity.
Prolongation of the lag phase reflects the functional abnormality of the gastric antrum and represents the stomach’s inability to grind food in a normal timely fashion. Drugs such as erythromycin improve the gastric emptying rate by shortening the lag phase.
Quantification of the gastric emptying rate and the lag phase are of particular clinical importance in follow-up and evaluation of the effectiveness of treatment. Response to treatment can be judged based on the quantitative results of the gastric emptying study.
In evaluating the gastric emptying curve, it is not unusual to observe an initial transient increase in the count rate. This is due to a reduction in the self-absorption of radiation in food. As the grinding process continues, food triturates into small particles of reduced thickness; therefore there will be fewer barriers to self-absorption and attenuation of gamma rays, resulting in more photons reaching the detector and causing the observed transient increase in count rate.
Accelerated gastric emptying may also be encountered. Duodenal ulcer, Zollinger-Ellison syndrome, hyperthyroidism, postvagotomy dumping syndrome, and medications such as erythromycin have been noted to be associated with accelerated gastric emptying.
Diagnosis: Delayed gastric emptying consistent with diabetic gastroparesis.
Case 5.2
History: This patient is an 84-year-old man who underwent cystectomy and ileal conduit for bladder cancer 2 weeks earlier. His history is pertinent for partial colectomy 2 years previously for colon cancer. He is now referred for a 99mTc–tagged RBC abdominal scintigram for the detection of a bleeding site in the setting of painless passage of bloody stool over the preceding 5 days.
Findings: Images from the 99mTc–tagged RBC abdominal scintigram (Fig. 5.2 A) show a focus of radioactivity in the right lower quadrant with rapid changes in configuration and migration to the left upper quadrant. This finding is most consistent with GI bleed originating in the terminal ileum.
Discussion: The patient’s bleeding site was confirmed angiographically (Fig. 5.2 B) as a focus of active extravasation from the distal branches of the SMA. He was subsequently taken to the operating room and found to have bled from the anastomotic site that had been repaired.
The detection of a focus of radiolabeled RBCs outside the normal area of expected vascular blood pool which moves within the bowel in either the antegrade or retrograde direction are the two important criteria for the diagnosis of GI bleeding.
Although RBC scintigraphy is a sensitive technique for the detection of a GI bleed, localization of the bleeding site is as important as detection. There are inconsistent reports in the literature as to the accuracy of scintigraphy for detecting and localizing a bleeding site. Variables to be considered for these inconsistencies are the rate of bleeding (massive versus minimal), pattern of bleeding (active versus intermittent), location (large bowel versus small bowel), length of study (60 minutes versus imaging over a longer time period), labeling procedures (in vitro versus in vivo), and the imaging technique (static images versus rapid dynamic imaging with movie display mode).
The current state-of-the-art in RBC scintigraphy is to use the high labeling efficiency (98%) of the in vitro technique and acquisition of the images in a rapid cine scintigraphic mode. The combination of these two techniques provides an overall
improvement in the quality of the blood pool image and the test’s accuracy.
improvement in the quality of the blood pool image and the test’s accuracy.
An abdominal scintigram obtained with 99mTc-tagged RBCs enables the detection of a bleeding of a rate as low as 0.1 to 0.4 mL/min; with angiography, the bleeding rate must be at least 0.5 mL/min to be detectable. However, clinical studies indicate that, in the majority of patients, the bleeding can be detected angiographically when the rate is greater than 1 mL/min.
More than 80% of the patients with active bleeding have a positive scan within the first 90 minutes of the study. If the initial image is negative, the patient can be reimaged at an interval up to 24 hours. Some patients who are not actively bleeding during the time of early imaging may bleed several hours later, resulting in a positive delayed scan. Up to 60% of patients with a negative RBC scan of 90 minutes will have a positive scan on delayed images at 4 to 24 hours. A delayed positive scan is commonly due to migration of blood through the small bowel and accumulation in the colon. Intense colonic activity on the delayed image where the early image is normal should be considered evidence of proximal GI hemorrhage and should not be mistaken for large bowel bleeding. This error in localization occurs because extravasated blood originating from the stomach, duodenum, ileum, or jejunum migrates rapidly to the colon and may pool there. Blood in the bowel is an irritant and has a cathartic effect that causes rapid movement of blood both antegrade and retrograde.
Figure 5.2 C represents a delayed 21-hour image of an abdominal blood pool scintigram in a patient with intermittent bleeding. Intense colonic activity is due to pooling of the blood originating from the patient’s jejunal arteriovenous malformation detected angiographically. Lack of radioactivity in the stomach and renal collecting system helps to exclude unbound 99mTc (free technetium) as the cause of colonic activity.
Overall, detection and localization of bleeding in the large bowel is easier than in the small bowel. The well-known
anatomic location of the cecum, flexures, and transverse colon facilitates the localization of bleeding, making it more identifiable than bleeding within the highly peristaltic and moving small bowel. On Figure 5.2 D, the GI bleed within the transverse colon is easily recognizable, with the blood moving in both directions.
anatomic location of the cecum, flexures, and transverse colon facilitates the localization of bleeding, making it more identifiable than bleeding within the highly peristaltic and moving small bowel. On Figure 5.2 D, the GI bleed within the transverse colon is easily recognizable, with the blood moving in both directions.
Selection of patients for noninvasive RBC scintigraphy versus other invasive diagnostic procedures for providing the most favorable outcome is based on the clinical presentation. Upper GI endoscopy is usually used with an accuracy of about 90% for the diagnosis of upper GI bleeding commonly caused by gastric ulcer, duodenal ulcer, gastric erosion, and varices.
Colonoscopy and sigmoidoscopy may disclose the source of lower GI bleeding commonly caused by neoplasm, polyps, diverticula, and angiodysplasia in 60% to 77% of cases. However, owing to the intermediate nature of GI bleeding, endoscopy and angiography may fail to identify the sources of bleeding. Colonic examination may be very difficult when active, perfuse bleeding is present.
99mTc–tagged RBC scintigraphy can play a major role in triaging and screening the patient for GI bleed. Patients who are actively bleeding and are bleeding intermittently are good candidates for scintigraphic evaluation.
A positive scan can not only make the diagnosis but also identify patients who would benefit from angiography. When the scan is not positive, it is unlikely that angiography will demonstrate a bleeding site. In addition, a positive scan can be helpful in directing the angiographic approach to a specified vascular territory.
A scan can also be used to predict the need for surgery versus medical therapy. Patients who show extensive extravasation of labeled RBCs in the first hours of the study, with the intensity of the extravasated blood pooling being greater than pooling in the liver, usually find their way to the operating room.
Diagnosis: Active small bowel bleeding from the postsurgical anastomotic site in the terminal ileum.
Case 5.3
History: This 80-year-old male with multiple medical problems–including coronary artery disease, COPD, obstructive uropathy, and bilateral above-knee amputation–presents with GI bleeding requiring eight units of packed RBCs over the previous 24 hours. He was referred for a 99mTc-tagged RBC abdominal scintigram for detection of the bleeding site.
Findings: Sequential dynamic images of the abdomen over a period of 60 minutes (Fig. 5.3 A) show abnormal distribution of the labeled RBCs in the epigastrium outlining the duodenal loop (GP 1 -15 M), seen repeatedly throughout the hours of examination with some changes in configuration and intensity. On the second group of images (GP 15-30 M), another collection of labeled RBCs appears in the left lower quadrant, which persists throughout the sequence with some changes in intensity and configuration. A large area of stable and nonmobile activity is also present in the midabdomen.
Discussion: Diagnosis of GI bleed in duodenum and sigmoid colon was made based on the observation of activity outside the normally expected vascular blood pool with changes in shape, location, and intensity, even though minimal. The patient was subsequently taken to the operating room, and bleeding from a duodenal ulcer as well as sigmoid colon diverticula was noted.
Application of the rapid sequence cine scintigraphic technique in this case facilitated not only the early detection of bleeding in the duodenum but also bleeding from another site in the sigmoid colon. Otherwise, the sigmoid bleeding might have been mistaken for the phenomenon of rapid movement of blood originating from the duodenum and pooling in the sigmoid.
In RBC abdominal scintigraphy, a variety of abnormalities may be detected incidentally. The large fusiform area of intense but stable activity in the midabdomen seen in Figure 5.3 A. A represents a large abdominal aortic aneurysm. The activity there is stable and does not change in location, configuration, or intensity and does not represent bleeding.
Figure 5.3 B represents a selected image of an abdominal blood pool in a 47-year-old patient with hepatic cirrhosis who presents with hemoptysis and guaiac-positive stool. The image is notable for markedly reduced hepatic blood pool activity consistent with this patient’s known hepatic cirrhosis and associated reduced portal blood flow. There is a region of increased activity (arrow) within the midabdomen medial to an enlarged spleen. This activity, which did not migrate or change in configuration or intensity on subsequent serial images, does not fulfill the criteria for acute GI bleeding. Further evaluation of this area by ultrasound (Fig. 5.3 C) reveals multiple dilated mesenteric veins secondary to portal hypertension; pooling
of tagged RBCs within these varicose veins accounts for the scintigraphic abnormality.
of tagged RBCs within these varicose veins accounts for the scintigraphic abnormality.
On an abdominal blood pool scintigram, in addition to the well-known lesion of the hepatic cavernous hemangioma, increased activity may be identified in an accessory spleen, ectopic kidney, vascular graft, and pseudo-aneurysm. Increased activity in these structures should not be mistaken for GI bleed, as these areas are stationary and do not demonstrate changes in their appearance.
As in most imaging procedures, recognition of physiologic and anatomic variants is important for the accurate interpretation of blood pool scintigraphy. There are several normal structures visualized on the 99mTc-RBC scintigram that might easily be confused with bleeding. In addition to the aorta, inferior vena cava, and iliac vessels, the smaller vascular structures–such as the portal and splenic veins–are often visualized and may be mistaken for bleeding in the transverse colon, duodenum, or stomach. Occasionally, the superior mesenteric vein and inferior epigastric vein are visualized. Vessel visualization usually is more pronounced on the early images and later fades slightly. Also, the lack of migration of radioactivity should help in their differentiation from bleeding. If no movement occurs, no active GI bleeding is present.
A vascular blush not uncommonly appears in the left lower quadrant and represents the mesenteric vascular bed; it should not be erroneously diagnosed as GI bleeding. Uterine blush also may be seen as a result of uterine hyperemia. A penile blood pool overlying the lower abdomen may be mistaken for bleeding. Figure 5.3 D shows intense focal and linear uptake over the left lower pelvis (arrow) in a 65-year-old man with history of duodenal ulcer and melena, mimicking sigmoid bleeding. Error in the diagnosis was avoided by repeating the images following a change in penile position.
In abdominal blood pool imaging, trace amount of activity may be seen in the stomach, kidney, collecting system, and bladder. Visualization of the urinary collecting system is more pronounced on the later images. This artifactual activity is due to the presence of a small amount of unbound 99mTc (free technetium) and should not occur to any significant degree with the currently used improved in vitro RBC labeling.
Diagnosis: Active GI bleed in the duodenum and sigmoid.
Case 5.4
History: A 5-year-old otherwise healthy child presents with an unexplained GI bleed. He undergoes an abdominal radionuclide scintigram following the IV injection of 3 mCi of 99mTc-pertechnetate (Fig. 5.4).
Finding: The anterior projection of the abdomen (Fig. 5.4) shows a focal area of increased uptake (arrow) in the right lower quadrant superior and lateral to the visualized urinary bladder on the images obtained at 20, 25, and 30 minutes.
Discussion: The anatomic location, persistent activity, and synchrony with gastric visualization are consistent with the diagnosis of Meckel’s diverticulum containing gastric mucosa.
Meckel’s diverticulum is a congenital anomaly of the GI tract occurring in 2% to 3% of the population and is the result of incomplete atrophy of the omphalomesenteric duct. It is a true diverticulum composed of all layers of intestinal ileum occurring on the antimesenteric border of the ileum within 100 cm of the ileocecal valve. Heterotopic gastric and pancreatic tissue is seen in approximately 50% of resected Meckel’s diverticula, with gastric mucosa being the most common finding.
In the majority of patients, Meckel’s diverticulum is asymptomatic. Clinical symptoms arise from complications of the diverticulum such as peptic ulceration and hemorrhage, which commonly occur before the patient reaches 10 years of age. Ectopic gastric mucosa produces gastric acid and pepsin, which can cause mucosal ulceration and hemorrhage. Bleeding is brisk and painless.
Bleeding from a Meckel’s diverticulum can be diagnosed by labeled RBC scintigraphy; however, the procedure is less sensitive and less specific than the Meckel’s scan.
A Meckel’s scan is performed following IV injection of 99mTc-pertechnetate. Serial images of the abdomen are obtained over a period of 30 minutes. The mucous cells of the gastric epithelium, when present in the diverticulum, accumulate and excrete the pertechnetate; therefore the diverticulum appears as an area of focal accumulation of radioactivity, usually in the right lower quadrant.
In the diagnosis of Meckel’s diverticulum, attention should be paid to the timing of the appearance of focal uptake. It should be in synchrony with visualization of the stomach.
Ectopic gastric mucosa may also be found in enteric duplication (located on the mesenteric side) and duplicated cysts. Therefore these anomalies should be entertained in the differential diagnosis, although differentiation is not possible by scan. But the distinction is not as important, as all will be treated surgically.
In the pediatric population, the Meckel’s scan has a sensitivity, specificity, and accuracy of 85%, 95%, and 90% respectively. The result would be significantly lower in the adult population, as the prevalence of hypertrophic gastric mucosa declines with age.
A false-positive scan may occur as a result of inflammation and local hyperemia, ulcerative colitis, Crohn’s disease, intussusception, retention of radioactivity in the distal right ureter, and arteriovenous malformation of the bowel. A false-negative scan occurs in the diverticulum that does not contain gastric mucosa, or there may be inadequate tissue to concentrate 99mTc-pertechnetate.
Diagnosis: Meckel’s diverticulum.
Case 5.5
History: A 52-year-old man presents to triage with the complaint of right-upper-quadrant pain and tenderness over the past 3 days. He denies nausea or vomiting. There are no chills or fever. He is referred for a hepatobiliary scan (Fig. 5.5 A).
Findings: There is persistent nonvisualization of the gallbladder up to 3 hours. There is enhanced activity (arrowhead) along the inferior hepatic border, known as the rim sign, which is best seen on the delayed 2-hour image.
Discussion: Persistent nonvisualization of the gallbladder under proper clinical conditions is most consistent with acute cholecystitis. The rim sign, in association with nonvisualization of the gallbladder, is highly specific for acute cholecystitis and was originally described with acute gangrenous chole-cystitis.
Figure 5.5 B shows an ultrasound image of the right upper quadrant demonstrating multiple echogenic foci within a thickened gallbladder wall that cast (dirty) acoustic shadows consistent with gas and emphysematous cholecystitis.
Acute gangrenous cholecystitis may also appear as a large area of photopenia in the gallbladder fossa with or without the rim sign. This appearance usually represents a relatively late and more severe stage of the disease process.
The rim sign has a high positive predictive value (95%) for acute cholecystitis; when it is present, there should be no need for delayed 3- to 4-hour images. Demonstration of the rim sign, even in the presence of a visualized gallbladder, should be considered as indicating a high probability for acute cholecystitis.
The rim sign should not be confused with normal anatomic variants. In patients with a prominent porta hepatis, the portal area appears as a photopenic region surrounded by normal liver tissue mimicking a rim sign. To avoid misinterpretation, ultrasound correlation should be considered for this atypical appearance.
The rim sign may also be seen along the inferior hepatic border in a patient with recent cholecystectomy. This should not be mistaken for inflammation. It is related to surgical manipulation and retraction of the liver during the surgery.
The rim sign is probably related to the prolonged transition and slow clearance of radioactivity from the edematous and hyperemic liver tissue adjacent to the inflamed gallbladder. Theoretically, the sign occurs throughout the hepatobiliary sequences, including scintiangiography, but it is more easily detected on delayed images as the activity of normal liver tissue disappears and the rim sign activity stands out. It is to be noted that high-count, high-intensity images should be obtained so as not to overlook the rim sign.
Diagnosis: Acute gangrenous cholecystitis.
Case 5.6
History: A 62-year-old chronically ill patient on total parenteral nutrition (TPN) complains of right-upper-quadrant pain and tenderness. Gallbladder ultrasound (Fig. 5.6 A) shows a moderately distended gallbladder with sludge. Figure 5.6 B is a hepatobiliary scan done to exclude acute cholecystitis.
Findings: The hepatobiliary images show uniform hepatic uptake of hydroxy iminodiacetic acid (HIDA) radiotracer with excretion into the bile duct and small intestine. There is no visualization of the gallbladder by 60 minutes. Ten minutes after IV injection of 2.5 mg of morphine sulfate, the gallbladder is visualized (arrow).
Discussion: Nonvisualization of the gallbladder prior to administration of morphine could be the result of acute cholecystitis, chronic cholecystitis, hyperalimentation, prolonged fasting, physiologic distention of the gallbladder, pancreatitis, or chronic alcoholism.
Visualization of the gallbladder following IV injection of morphine confirms the patency of the cystic duct, excludes cholecystitis, and suggests the diagnosis of a sludge-filled gallbladder with chronic cholecystitis.
Morphine enhances the tone of the sphincter of Oddi and causes increased intraluminal bile duct pressure severe enough to overcome resistance to bile flow into the sludge-filled gallbladder. Therefore there will be a diversion of bile flow into the gallbladder if the cystic duct is patent.
Morphine augmentation cholescintigraphy helps to avoid 3- to 4-hour delayed imaging without compromising the accuracy of the test. There are studies indicating that the results of morphine-augmented procedures are superior to conventional 3- to 4-hour delayed imaging for including or excluding acute cholecystitis.
Morphine should not be injected before 60 minutes into the study. If the gallbladder is not visualized by 60 minutes, morphine is injected and imaging is continued for an additional 30 minutes. Nonvisualization of the gallbladder at that time, in an appropriate clinical setting, is diagnostic for acute cholecystitis. If the gallbladder is not visualized by 60 minutes but is seen by 30 minutes following morphine injection, the diagnosis of abnormal gallbladder function can be made.
A technical note is that a sufficient amount of radiotracer must be present within the hepatobiliary system at the time of morphine injection to allow visualization of the gallbladder. If it is not, then a booster dose may be used.
It is to be noted that there may be a higher frequency of false-positive (nonvisualized gallbladder) diagnoses in a morphine-augmented study in severely ill patients as well as those receiving TPN. Therefore complementary ultrasound should be used liberally. In this group of patients, the greatest value of the ultrasound lies in the very high negative predictive value of the procedure.
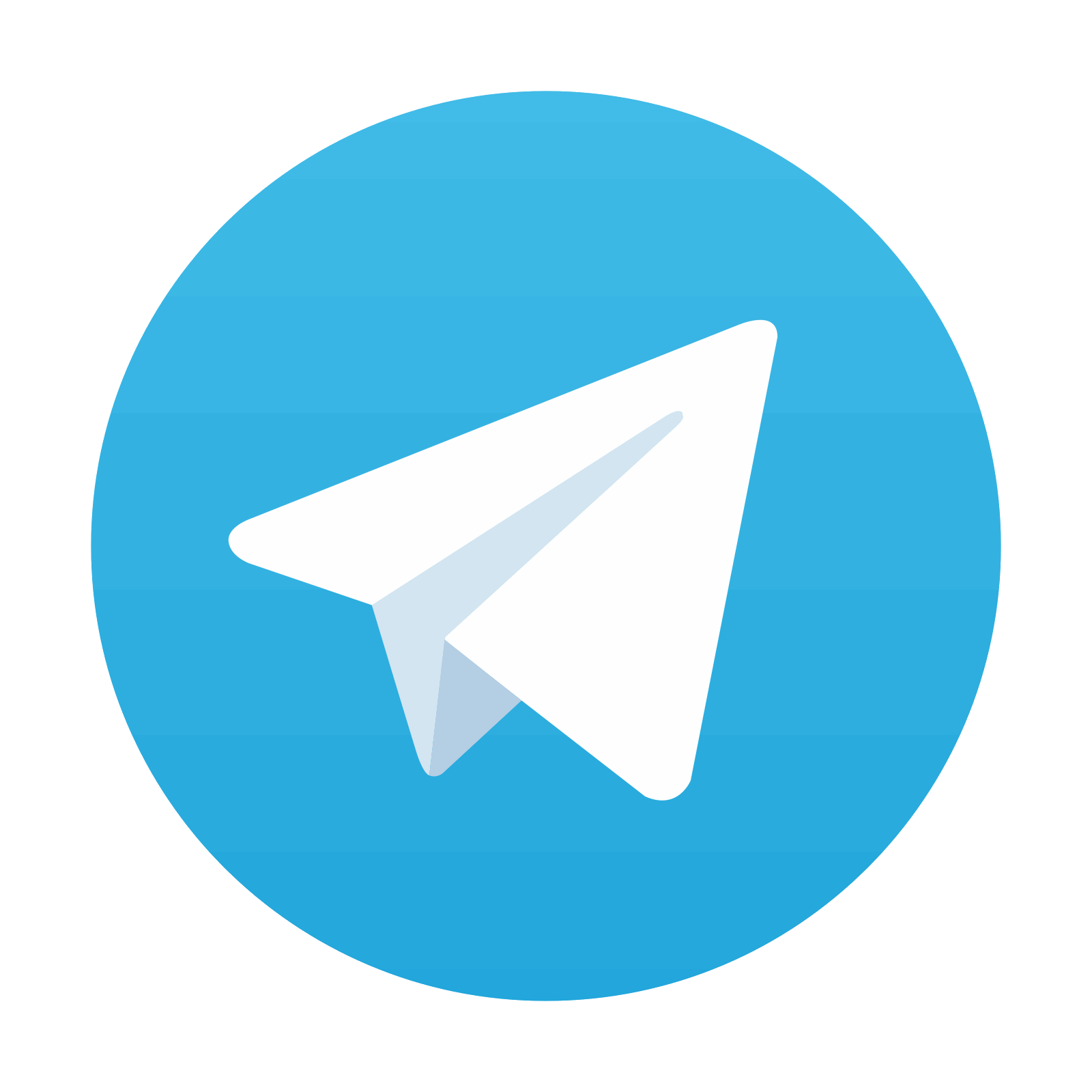
Stay updated, free articles. Join our Telegram channel

Full access? Get Clinical Tree
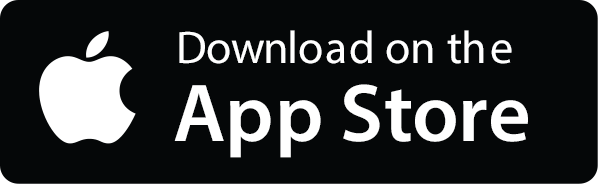
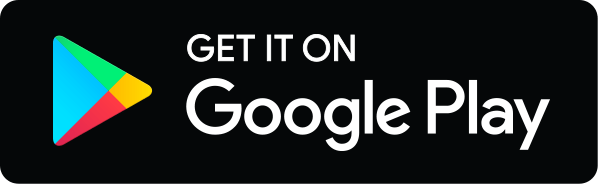