Ricardo Yamada • Christopher Hannegan • J. Bayne Selby • Marcelo Guimaraes
Cellular therapy has been used since late 1950s, when transplantation of hematopoietic cells was performed by E. Donnall Thomas1 based on the previous work of Jean Dousset, who identified the first human leukocyte antigen on the surface of cells, leading to a better understanding of histocompatibility and rejection. Since then, cell therapy, in the form of bone marrow transplant, has become a well-established treatment modality for some hematologic disorders. Recently, this therapeutic approach has expanded to other organs and systems, including the central nervous system, liver, pancreas, and heart. This expansion relies on several factors, such as technical improvements in cell harvesting, tracking, delivery, and engraftment.
Cell delivery to the target organ is a key step in the whole process. Bone marrow transplant is done by delivering hematopoietic cells through systemic infusion via a central venous access. Because bone marrow is located within multiple sites, systemic infusion permits widespread cell colonization. However, for a specific target organ, such as brain, heart, or liver, systemic infusion is inadequate because most of the cells will be delivered away from their desired destination, leading to poor engraftment and increased cell loss. In this context, the catheter-based technique is extremely suitable as it provides the capability of selective delivery of transplanting cells to a specific organ in a minimally invasive fashion. Therefore, selective intravascular administration of therapeutic cells, either through arteries or veins, is a growing field yet to be fully explored.
DEVICE/MATERIAL DESCRIPTION
In contrast to other procedures described in this book, here, the embolic agents are live cells capable of altering the targeting organ function. The embolic effect is not the primary goal but rather a secondary result of the cell delivery process. Development of these techniques has expanded the usefulness of minimally invasive procedures beyond structural/anatomic corrections to also comprise specific cellular physiologic changes such as increased production of insulin and improved toxin clearance.
Unfortunately, the source of the “embolic agent” is a major limiting factor for widespread use of cellular therapy, as cell availability is limited by lack of donated organs. Currently, as described in the next sections, the pool of cells used for transplantation is obtained by isolating them from the donated organs of a different person (allogeneic transplant) or from the patient’s organ itself (autotransplant).
To overcome this problem, another potential source for cells is under constant investigation—the so-called stem cells. There are two different types of stem cells: embryonic and adult. The embryonic stem cells are obtained from the inner cell mass of the blastocyst and have the capability of differentiation into any type of cell (pluripotent). On the other hand, adult stem cells are obtained from the bone marrow, adipose tissue, or umbilical cord and have less differentiation capability (multipotent).2 Ethical and religious aspects limit embryonic stem cell research and clinical application, but adult stem cell use is not controversial and might become an unlimited cell source.
PANCREAS
Type 1 Diabetes Mellitus
Clinical Application
According to the World Health Organization’s (WHO) last update on diabetes mellitus (DM), there are more than 34 million people worldwide affected by type 1 DM.3 Those patients have poor quality of life and decreased life expectancy due to inadequate glycemic control, which leads to acute and chronic complications, including severe unawareness of hypoglycemic events, retinopathy, glomerulopathy, and neuropathy. Pathogenesis is based on autoimmune destruction of β-cells within the islet of Langerhans. These cells are responsible for insulin production and are the most predominant pancreatic islet cells, representing up to 75% of islet composition.4
Currently, the mainstay therapy is intensive exogenous insulin administration through multiple daily subcutaneous injections or continuous insulin infusion via an implanted pump. Despite all different insulin regimens available, control of glucose level is not ideal yet, and high incidence of hypoglycemic events is still a major concern. So far, the only treatment that has shown successful results in controlling disease progression without hypoglycemic events is total pancreas transplantation.5,6 However, organ transplant is limited by lack of donors, in addition to associated comorbidities related to surgery and rejection.7
To overcome the unsatisfactory glucose level control with exogenous insulin therapy and surgical complications of whole pancreas transplant, a lot of effort has been put into developing an alternative therapy, which is β islet cell transplantation (ICT). This procedure gained a lot of attention after 2000, when Shapiro et al.8 reported the results of the Edmonton series in which all seven patients were still insulin-independent after a median follow-up of 11.9 months. This result was based on the use of a glucocorticoid-free immunosuppressive regimen. Until that time, only 8.2% of patients were insulin-free 1 year after ICT.9
Most likely, the population that benefits the most from ICT is the group of patients who have labile diabetes and/or severe hypoglycemic events with sustained renal function because whole pancreas transplant alone has higher morbidity and mortality compared to ICT.10 Patients with associated chronic renal insufficiency benefit more with kidney–pancreas transplant.
Labile diabetes is defined as inconsistent glucose levels that follow no predictable pattern, interfering with a patient’s quality of life. To quantify that, a lability index (LI) was created based on glucose level measurements during a period of 4 weeks.11 A severe hypoglycemic event is defined as a hypoglycemic episode that requires outside assistance to be treated; a composite hypoglycemic score, called HYPO, was also developed to quantify the frequency, severity, and degree of unawareness of hypoglycemia.11 These measurement systems are important to promote an objective indication of the disease’s severity and thus guide treatment choice.
Similar to whole-organ transplantation, ICT for type 1 DM requires lifelong immunosuppressive therapy, which is associated with frequent and severe side effects. In addition, despite its minimal invasiveness, the procedure carries some risk of serious complications, such as infection, bleeding, and portal vein thrombosis. Therefore, the procedure should be performed only in patients who present severe complications related to poor glycemic control and/or unacceptable quality of life. The patient should understand clearly that exogenous insulin therapy would be exchanged for an immunosuppressive therapy, and so the problems associated with type 1 DM must be severe enough to justify it.
Regardless of the increasing success rate that has been reported,12 ICT is not yet considered a conventional therapy. Issues related to organ availability, cell extraction, delivery and engraftment, and also immunosuppressive regimen remain limiting factors. Hence, according to the American Diabetes Association,10 ICT should be performed only in the setting of controlled research studies in a tertiary care center capable of managing all complex medical situations associated with transplanted patients.
According to the last Collaborative Islet Transplant Registry (CITR) annual report, insulin independence can be achieved in up to 80% to 90% of patients, especially in the presence of favorable predictive factors, such as lower baseline insulin requirement and age older than 35 years.12 Unfortunately, sustained insulin independence decreases over time, and only 10% of patients remain insulin-independent 5 years after transplant.12 Graft functionality is determined by any detection of serum C-peptide by local assay or stimulated serum C-peptide level greater than or equal to 3 ng/mL. Therefore, transplanted islet cells are categorized as fully functional (detectable C-peptide level and insulin independence), partially functional (insulin dependence but detectable C-peptide level), or nonfunctional (no detectable C-peptide).
Despite the low long-term insulin-independence rate, persistent C-peptide level greater than or equal to 3 ng/mL can be achieved in around 80% of patients even 5 years after transplant,12 meaning that a significant number of patients will have at least a partially functioning graft. This has been proven clinically significant by the drop in both hypoglycemic score and lability index13 seen also among insulin-dependent patients, representing improvement of glycemic control, which is the main goal of ICT. In addition, studies have demonstrated that ICT leads to stabilization and even some improvement of the long-term type 1 DM complications, including retinopathy,14 glomerulopathy,15 and neuropathy.16
Technique
The procedure is performed under moderate sedation, and preevaluation is focused on any conditions that increase sedation risk, such as airway compromise and cardiopulmonary dysfunction. If the patient has an increased risk for moderate sedation, general anesthesia should be considered. Blood workup includes serum creatinine, as the use of iodine contrast dye is necessary and these patients may have some degree of renal dysfunction. Platelets and prothrombin time are also needed because bleeding is the major procedure-related complication. For antibiotic prophylaxis, cefazolin sodium 1 mg intravenously 1 hour before the procedure and every 6 hours for 24 hours after the transplant has been typically used. Sulfamethoxazole/trimethoprim 400/80 mg is given orally twice a week for 24 weeks to prevent Pneumocystis pneumonia and valganciclovir 450 mg is prescribed orally once a day for 12 weeks to prevent cytomegalovirus infection.17
Under sterile technique and after local anesthesia, a 21-gauge Chiba needle is advanced into the liver parenchyma under fluoroscopic guidance at the level of the right midaxillary line, avoiding transpleural approach. The needle is advanced horizontally and with slight cephalic angulation (10 to 15 degrees). The abdominal midline should not be crossed. The needle is then slowly retracted while small amounts of contrast are injected, searching for portal vein branches. After finding a branch, a more forceful hand contrast injection is performed to confirm needle location within the portal system (Fig. 43.1). Use of ultrasound-guided puncture has been advocated as a safer approach, with less needle passages through the liver parenchyma and single-wall vein puncture.18 Ideally, a second- or third-order branch should be punctured to decrease the risk of bleeding, as the access to the portal system is upsized to accommodate a larger catheter. After confirming the needle location within the portal system, a 0.018-in wire is advanced into the main portal vein under fluoroscopic guidance and a 4-Fr catheter is introduced. After access is secured, heparin is given intravenously according to the patient’s weight. Baseline portal vein pressure is measured. Pressure above 20 mm Hg is a contraindication for cell infusion due to increased risk of portal vein thrombosis. Next, portography is performed through a power injector and a side-hole catheter, infusing 6 mL of iodine contrast per second and a total volume of 30 mL. Portal vein anatomy is delineated, confirming its patency and hepatopetal flow (Fig. 43.2).
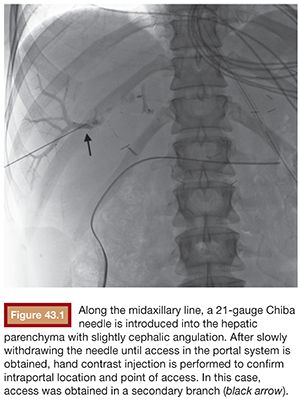
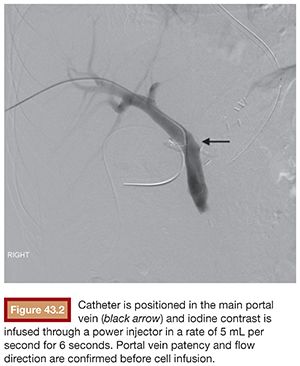
A catheter with at least 700-mm inner diameter is recommended for infusion to avoid cell damage from possible shear forces or increased pressure; therefore, a 4-Fr catheter is more than appropriate.19 The catheter tip is positioned beyond portal vein bifurcation to allow cell distribution in both hepatic lobes (Fig. 43.2). Harvested cell administration by gravity flow is preferred over direct syringe infusion because administration by gravity allows a safety mechanism of natural flow reduction that parallels any increase of portal vein pressure, avoiding precipitous pressure rise.19 Direct syringe infusion seems to be associated with an increased risk of portal vein thrombosis. To avoid that, bag method infusion uses a closed gravity feeding bag system, consisting of a transfer bag and a rinse bag connected via sterile tubing (Fig. 43.3). During infusion, portal vein pressure is measured periodically, and an increase of more than double the baseline or above 22 mm Hg for more than 10 minutes should prompt interruption of the infusion due to increased risk of portal vein thrombosis. After infusion, a final portal vein pressure and portography are obtained.
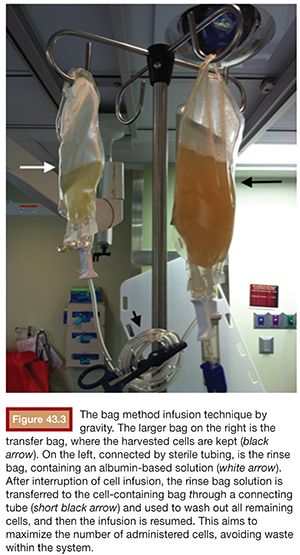
Despite the use of low-profile systems (4-Fr), embolization of the parenchymal track is performed at the end of the procedure as it is believed to substantially decrease the risk of postprocedure bleeding.20 Under fluoroscopic guidance, the catheter is slowly withdrawn from the portal vein. Proper catheter location within the parenchymal track is confirmed with gentle hand injection of a small amount of iodine contrast. Embolization of the track can then be performed with different types of embolic agents, including gelfoam, coils, and N-butyl cyanoacrylate. The ideal embolic agent should promote a complete seal of the track with accurate deployment, avoiding intravascular embolization. Embolization is recommended for at least 5 to 7 cm of hepatic parenchyma to prevent postprocedure bleeding from the liver surface.21
After the procedure is terminated, the patient should be admitted to an intensive care unit, with close monitoring of vital signs and complete blood cell count measurement every 6 hours. If bleeding is not initially suspected, to prevent portal vein thrombosis, intravenous heparin infusion is started, aiming a thromboplastin time of 50 to 60 seconds. After 48 hours, intravenous heparin is switched to subcutaneous low-molecular-weight heparin for 1 week.
Chronic Pancreatitis
Clinical Application
Chronic pancreatitis (CP) is an important disabling entity that leads to significant detriment of life quality, mainly due to severe abdominal pain and opioid abuse. CP has a reported incidence of 3.5 to 10 per 100,000 people per year, and incapacitating pain is present in nearly 90% of these patients.22 The primary treatment goal is pain alleviation, which can be associated with pancreatic duct dilation or not. When ductal dilation is present, endoscopic or surgical drainage is indicated. However, when dilation of the pancreatic duct is absent or drainage fails, total pancreatectomy (TP) should be considered.
In patients with established diabetes, the decision to proceed with pancreatic resection is more convenient because surgery is not adding a new comorbidity. On the other hand, nondiabetic patients submitted to pancreatectomy will have an incidence greater than 50% of postoperative diabetes, usually associated with more difficult glucose control and severe hypoglycemic events.23,24
To overcome this problem, islet cell autotransplantation (IAT) was first introduced in 1977 in the University of Michigan.25 Since then, other centers in the United States have been performing this type of transplant successfully. In this process, the resected organ is sent to a cell laboratory, where a collagenase-based digestion process is started by pancreatic duct cannulation and enzymatic infusion. This will separate islet cells from the exocrine pancreas and connective tissue. Once islet cells are isolated, they are placed in a bag with albumin solution and antibiotic, and they are ready to be transplanted.
In the initially described technique, the patient (under general anesthesia) and the surgical team wait in the operating room (OR) while the cells are harvested. This process usually takes around 4 hours. More recently, we started performing the cell infusion at the interventional radiology (IR) angiography suite with the goal to maximize cost and efficiency. Soon after the pancreas is resected, the cells get processed at the cell therapy center while the patient’s abdomen is closed. Alternatively, the patient may be sent to the intensive care unit for a few minutes in case there is any delay in pancreatic islet cell separation and preparation process26 (Fig. 43.4).
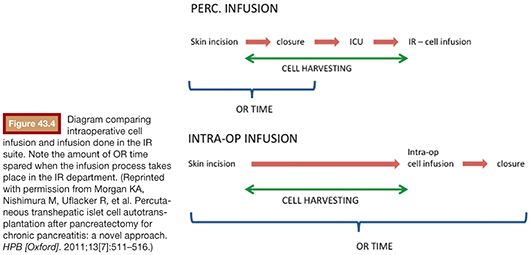
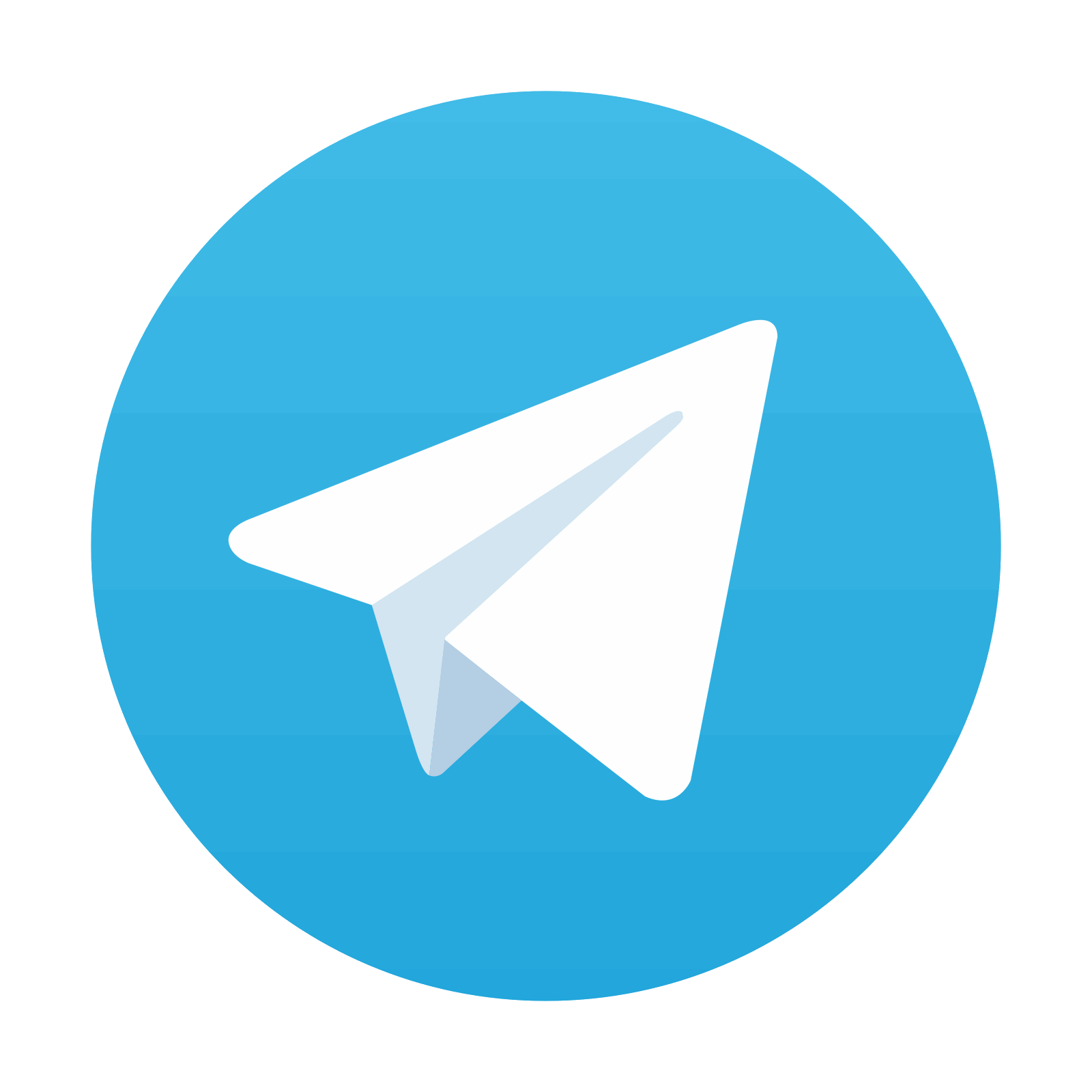
Stay updated, free articles. Join our Telegram channel

Full access? Get Clinical Tree
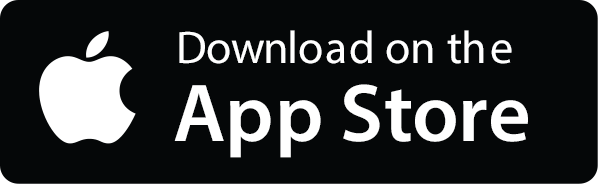
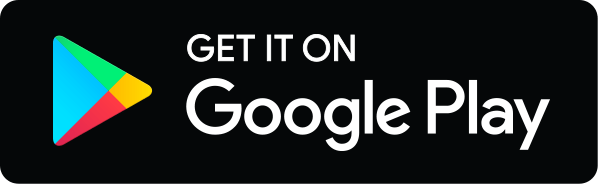