Type of cancer
5-year survival (%)
kV X-rays
MV X-rays
Hodgkin’s disease
30–35
70–75
Ca. of the cervix
35–45
55–65
Ca. of the prostate
5–15
55–60
Ca. of the nasopharynx
20–25
45–50
Ca. of the bladder
0–5
25–35
Ca. of the ovary
15–20
50–60
Retinoblastoma
30–40
80–85
Seminoma of the testis
65–70
90–95
Embryonal ca. of the testis
20–25
55–70
Ca. of the tonsil
25–30
40–50
Among various types of charged particles, protons and carbon ions have been most widely employed for cancer therapy in the world. The energetic ion beam deposits much of its energy at the end of its range, resulting in what is called the Bragg peak (Fig. 1.1), so-named after Sir William Henry Bragg, a British physicist. He reported this phenomenon in 1904 [6]. Realizing the advantage of the Bragg peak, Robert R. Wilson (1914–2000) published his seminal paper on the rationale of using accelerated protons and heavier ions for human cancers in 1946 [7]. This was the first proposal to apply charged particles for medical use. He participated in the development of the atomic bomb in Los Alamos during World War II. After the war, he returned to Berkeley to look for peaceful atomic-energy projects, and he wrote a historic paper on the potential benefit of high-energy protons in cancer therapy. He later became the first director of the Fermi Laboratory, where clinical research of fast neutron therapy was conducted on more than 3,100 patients. Compared to conventional photon treatment, charged particle beams appeared to promise higher cure rates with smaller complications as they could deliver sufficient doses precisely, while lowering unwanted doses to normal tissues adjacent to the tumor. Wilson also hypothesized that carbon ions might be superior to proton beams.
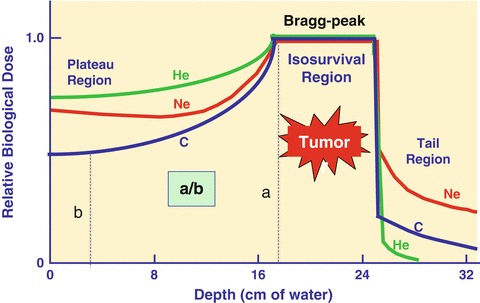
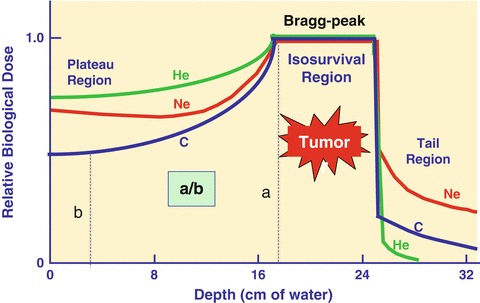
Fig. 1.1
Depth dose distributions of various ion beams. When the same biological endpoint is used, carbon-ion beams have the largest peak to plateau ratio (a/b) in biological effect as compared to other ion beams
Prior to Wilson’s proposal, Ernest Orlando Lawrence (1901–1958) discovered a method of accelerating particles to very high energy without the use of high voltage, which was actually the development of the cyclotron in 1929. The first model of Lawrence’s cyclotron was made of brass, wire, and sealing wax and was only 4 in. in diameter—it could literally be held in one hand. A photograph of Lawrence holding the first cyclotron in his hand appeared on the front cover of “Time” newsmagazine. Subsequently, higher-energy cyclotrons 11, 27, and 37 in. in diameter were constructed at Berkeley.
In 1938, the world’s first particle therapy was conducted on 24 patients with a single fraction of fast neutrons generated by the historic 37-inch cyclotron. This treatment was judged as successful, and then from 1938 to 1943 a total of 226 patients were treated with fractionated fast neutrons generated by a 60-inch cyclotron. Together with the clinical effects of fast neutron therapy, however, long-term side effects on normal tissues deemed were too high, and in 1948 Dr. Stone concluded that fast neutrons should not be used for cancer therapy [8].
A British group, however, evaluated fast neutron therapy again, and in 1965 Mary Catterall at Hammersmith Hospital in London once more began this therapy. By 1969, it was shown that for certain tumors, better local control could be achieved with neutron irradiation. Encouraged by these results, many other institutes in Europe, the USA, and Japan began neutron therapy research in the 1970s. However, the demand for fast neutron therapy decreased thereafter, and most of the institutions abandoned this therapy. Fast neutron therapy is currently conducted at only a few facilities for the treatment of selected tumors.
Incidentally, in the same year (1938) of the start of fast neutron therapy, Gerald Kruger, a physicist at the University of Illinois, came up with a new idea for cancer therapy using alpha particles emitted from boron when it captured neutrons. He proposed to saturate the tumor with a boron compound and then exposed it to a neutron beam. The boron capture cross section for thermal neutrons was about 100 times higher than that of other tissue compounds. This method came to be known as “neutron capture therapy.”
1.2.1.1 First Phase of Proton Beam Radiotherapy
In 1947, E. Lawrence completed construction of the 184-inch synchrocyclotron at the University of California (UC) Berkeley, making it possible to accelerate protons, deuterons, and helium nuclei to energies of several hundred MeV/u. Protons and heavier ions, being much more massive than electrons, require bigger accelerators to accelerate them to produce enough kinetic energy to treat deep-seated tumors. For example, a proton is 1,836 times heavier than an electron. E. Lawrence suggested that Cornelius A. Tobias and John H. Lawrence at UC Berkeley jointly use the 184-inch cyclotron to test the scientific validity of Wilson’s ideas [9].
Prior to irradiation of human patients, they decided to deliver deuteron beams to the pituitary of a dog patient with breast cancer in 1954 [10]. The dog’s tumor was ulcerated, bleeding and oozing milk continuously. Within a few days, pituitary irradiation caused a noticeable effect and the bleeding stopped. Within 2 weeks, the secretion of milk dried up completely and the animal had regained some strength. The dog was well and her tumor remained in remission for several months before death due to tumor relapse.
In 1954, encouraged by this favorable effect on the animal tumor, the world’s first human patient with disseminated breast cancer had pituitary irradiation with protons generated from the 184-inch synchrocyclotron [11]. Therapeutic exposure was performed with ion beams for a total of 50 human patients (deuterons, protons, and helium ions). The first human patient with widespread breast cancer was given pituitary proton irradiation, about half of what they actually expected to be an effective dose. She showed almost immediate improvement but eventually died several months later. Although it was impossible to determine who might benefit from the treatment, the earliest optimistic sign was the reduction of both skin temperature and swelling. After pituitary irradiation, more than half of the patients treated exhibited some beneficial effects, although in some there was no effect at all. As for toxicities, the most prominent side effect was the development of diplopia. It was thought that protons and helium ions were most likely spread too wide inside the head due to multiple scattering, and such effect could be minimized if carbon or oxygen beams could be used.
The second series of pituitary irradiation were for patients with acromegaly. Eventually about 700 patients were treated. Unlike with other treatments, acromegaly remained in regression and the growth hormone levels stayed within normal limits for many years [12]. In 1957, the 184-inch synchrocyclotron was modified to accelerate helium nuclei. By the time of the closure of the facility in 1992, a total of 2,054 patients had been treated with helium ions. These initial treatments with protons in the 1950s and 1970s had been mainly aimed at pituitary tumors, as they could be localized by orthogonal plane X-ray films and rigid immobilization of the skull. This was the manner of proton therapy before the invention of computed tomography (CT).
1.2.1.2 Second Phase of Proton Beam Radiotherapy
Soon after the initiation of clinical studies at UC Berkeley, programs of proton therapy also began at other proton facilities. They were originally constructed for nuclear physics research, including Uppsala, Sweden (1957), Cambridge, Massachusetts (1961), Dubna (1967), Moscow (1969), St Petersburg (1975), Chiba (1979), Tsukuba (1983), and Villigen (1984) [13].
Charged particle therapy was only made practical for cancer therapy with the advent of CT scanning in 1973, which could accurately determine the beam path in a patient. In 1974, Suit et al. initiated studies of fractionated RT with protons [14]. In the 1970s and 1980s, however, throughout the world the tumors treated with protons were mostly choroidal melanoma, skull base tumors, and intracranial tumors (Table 1.2). Among them, the largest number of patients treated with proton beams had choroidal melanomas, the first tumor treated safely with a large dose of 60–70 GyE in 4 to 5 fractionations in 1 week. At that time, only at Tsukuba University were deep-seated tumors such as lung, esophagus, liver, uterine cervix, prostate, and head and neck malignancies extensively treated by Tsujii et al. [15].
Table 1.2
Distribution of tumors treated with proton beams in the early phase of its clinical application in the world. This was summarized in May 1993
Tumor sites | USA | Europe | Russia | Japan | Total (%) |
---|---|---|---|---|---|
Ocular melanoma | 1,698 | 2,196 | 355 | 44 | 4,293 (35.1) |
Skull base and upper spine | 3,132 | 15 | 1,678 | 58 |