Fig. 37.1
Cone beam angiography with three-dimensional reconstruction of sheep arch anatomy in contralateral projections
Table 37.1
Current indications for preclinical TAVR evaluation
Delivery system evaluation (acute studies: nonpermanent implant) |
Safety of access (femoral versus trans-apical) |
Delivery system |
Profile evaluation (vascular compatibility) |
Aortic arch trackability |
Valve component |
Delivery mechanism |
Retention and anchoring |
Retrieval |
Valve component evaluation (chronic studies: implantable) |
Stent frame |
Material biocompatibility |
Biomechanics (fatigability) |
Tissue valve |
Valve biocompatibility and healing |
Short (post-implant) and long-term performance |
Others: sutures + adhesive components (polymers) |
Experimental Models and Vascular Access
Several routes of implantation of TAVR devices have been reported in humans; however, the transfemoral and trans-apical approaches are the most commonly used. Due to the importance of vascular access to procedural success and clinical outcomes, emerging TAVR concepts are usually tested for safety at the experimental setting. The vascular anatomy in the porcine and ovine models is generally similar to humans. Contrary to humans, both animal models lack a common femoral artery (CFA) and thus, the usual access site is located within the proximal segment of the superficial femoral artery (SFA) or external iliac artery (EIA). Based upon our data, the mean vessel diameters are 5.3 ± 0.5 mm for the SFA and 5.85 ± 0.9 mm for the EI in sheep and slightly smaller in swine (SFA = 5.2 ± 0.5 mm, EIA = 5.63 ± 0.7 mm) (Fig. 37.2a–d). In comparison, the mean human CFA diameters (8.17 ± 1.14 mm) tend to be larger than both animal models [5]. In consequence, these vessel diameter ranges permit the validation of devices within the 22–24 F diameter ranges. Although the basic femoral vessel animal anatomy is similar to humans, the most important difference is the lack of a disease state (i.e., calcification), no significant tortuosity or vessel pathology (i.e., calcifications); the absence of these pathologies mitigates the trackability of the delivery system and renders a smoother delivery in the experimental setting (Fig. 37.2d–e). However, basic safety features can be successfully tested by the use of the experimental models. In addition, the acute and long-term safety and efficacy of large bore closure devices can also be tested at the experimental setting.
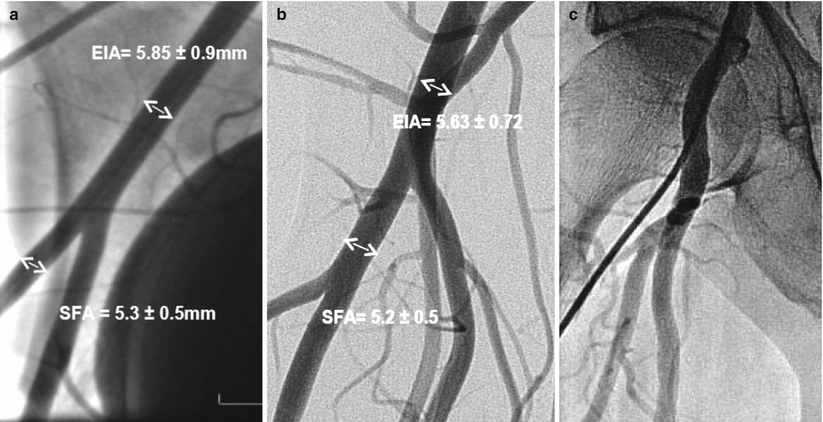
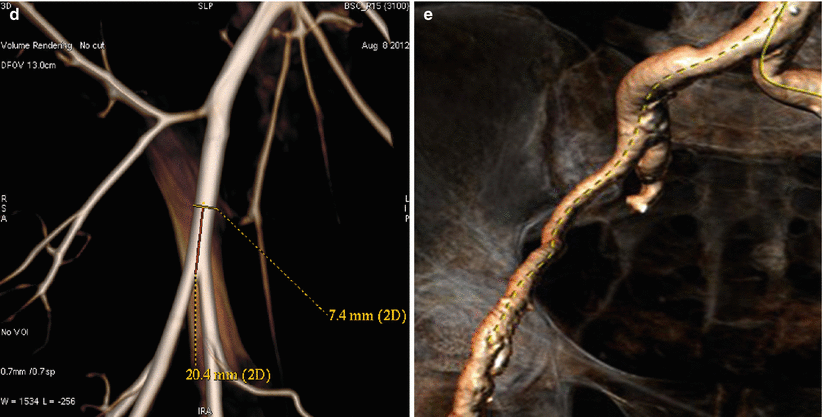
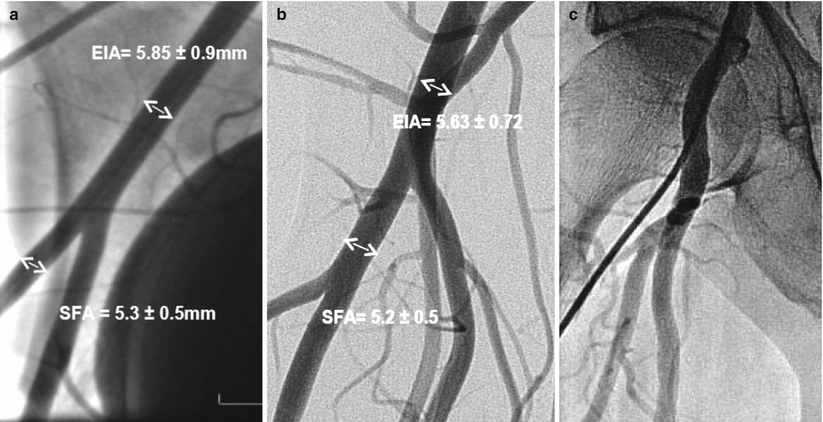
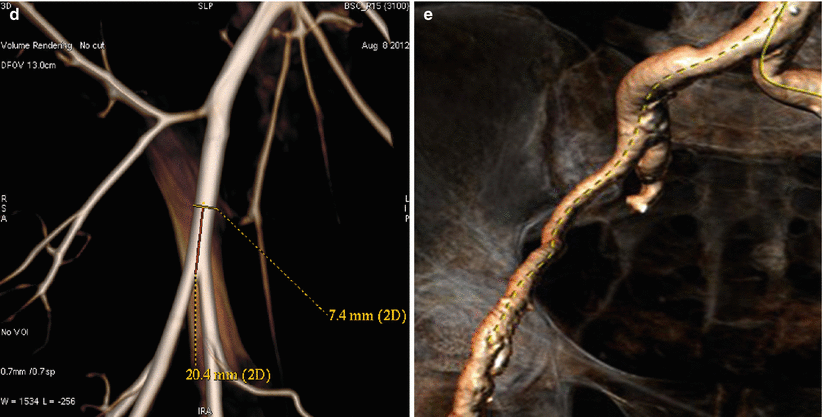
Fig. 37.2
Vascular angiography of ovine (a), porcine (b), and human (c) vascular access. Ovine (cone beam angiography) and human iliofemoral arteries (3D CT) are presented on picture (d) and (e). Average length 60–70 mm and diameter ~6 mm allows testing for devices that are within 22–24 F (Images a, b, and d, courtesy of Skirball Center for Cardiovascular Research. Images c and e, courtesy of American Heart of Poland)
The trans-apical approach can also be successfully evaluated in the preclinical setting. Vascular access is gained through a limited sternotomy or lateral thoracotomy to reach apex of the beating heart [6]. Full sternotomy may be also performed in order to facilitate direct aortic ultrasound imaging. Advanced tools, like MRI, could be potentially used not only for imaging (Fig. 37.3a) but also for procedural guidance (Fig. 37.3b). The porcine heart is an ideal model due to comparable human anatomy and physiology including wall geometry, myocardial wall thickness, ventricular wall motion, and hemodynamics. However, due to the fact that larger hearts are needed in some circumstances, the ovine model may be an acceptable alternative, particularly for displaying thin walled ventricles, similar to end-stage left ventricle remodeling due to CAVS. Similarly to the peripheral vascular approach, the testing of devices utilizing the apical approach (access and closure, Fig. 37.3b) can be performed [7, 8].
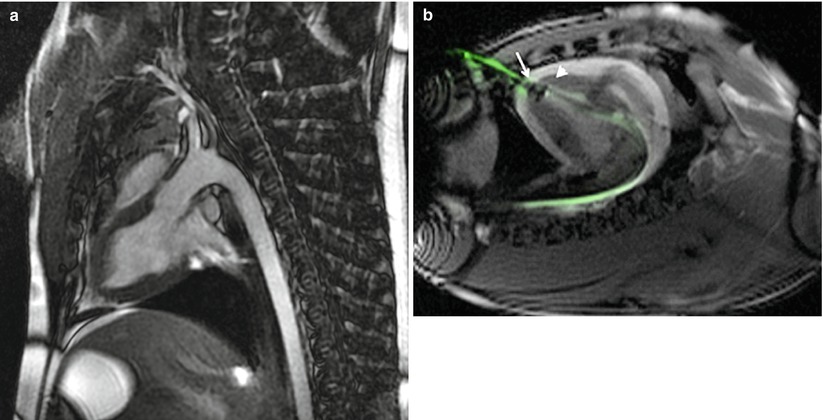
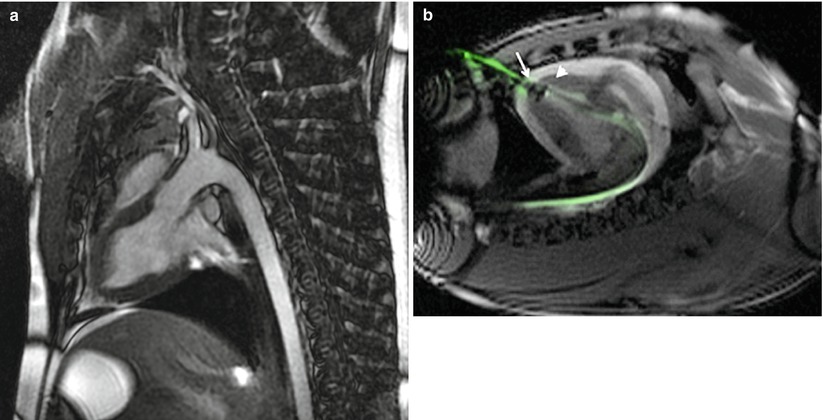
Fig. 37.3
MRI of aortic TAVR route (Image a, courtesy of American Heart of Poland) and guided placement of apical closure (arrow) displays the apex device in swine (arrowhead) (Image b, courtesy of I.M. Barbash [4])
Aortic Arch Anatomy and Delivery System Trackability
One of the most important components during the validation process of TAVR devices is the testing of delivery system tracking throughout the aortic arch. This is a particularly important feature as some neurovascular complications with TAVR are associated with interaction of the device to a diseased and highly calcific aorta. Although, in general terms, the basic anatomic landmarks are present in all large experimental models, major differences occur in the ascending and descending aortae compared to humans (Figs. 37.3a and 37.4a–d). Experimental models display a less angulated arch compared to humans, making it easier for devices to track throughout the process of valve delivery. In addition, the short length of the ascending aorta, a common feature of cloven-footed animals (calves, sheep, goats), gives way to the aortic bifurcation a short way downstream of the aortic valve. The decreased angulation of aortic arch makes the wire placement through the aortic valve and valve system positioning non-coaxial to annulus long axis. Consequently, these anatomical features make the transcatheter valve delivery technically challenging.
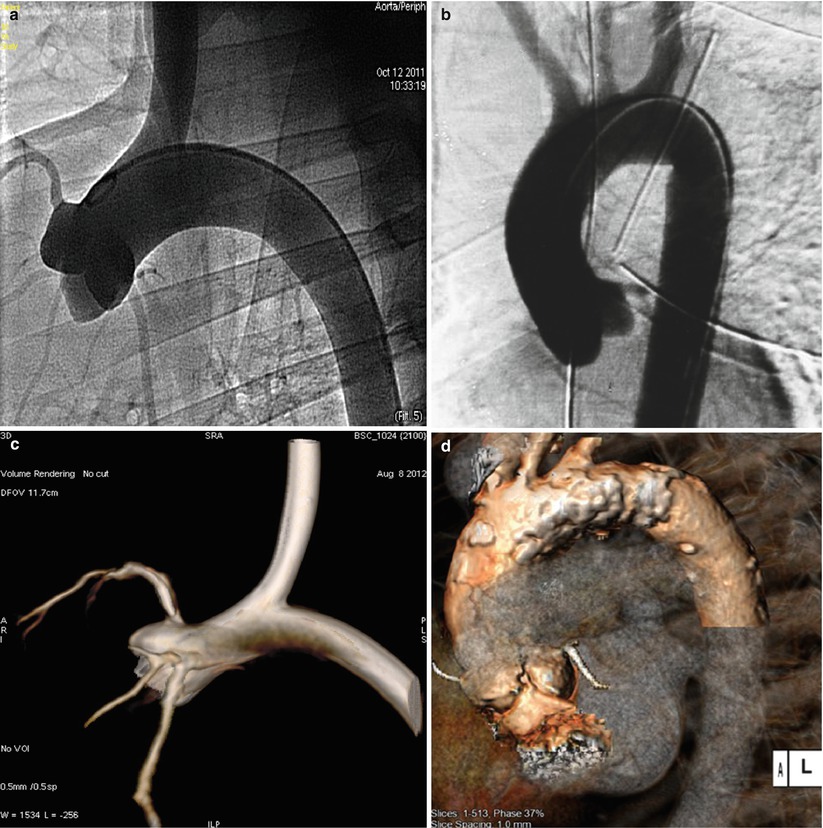
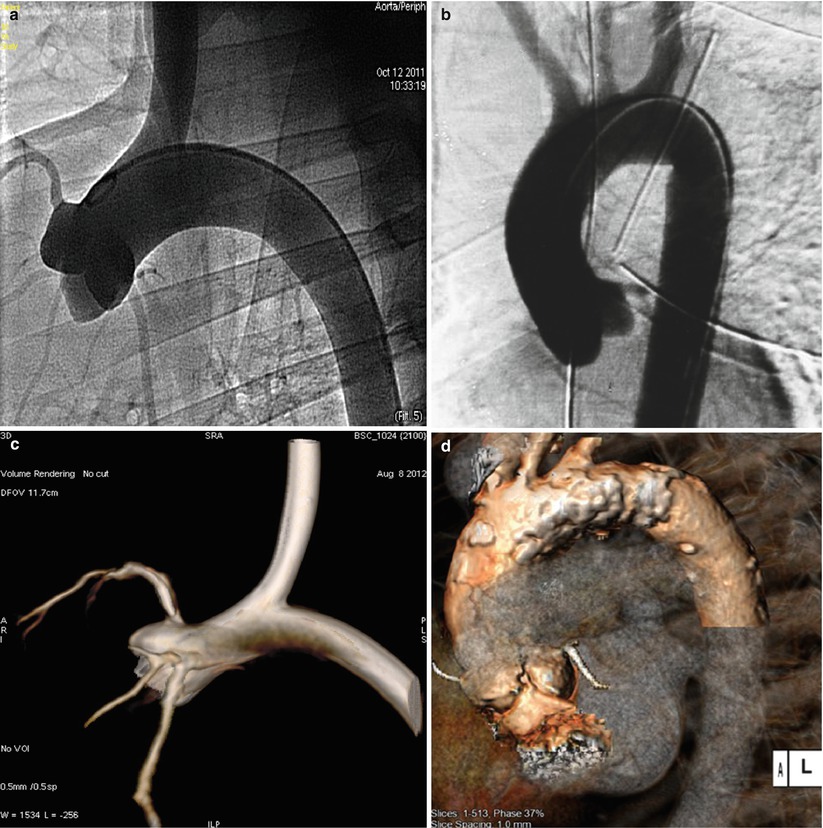
Fig. 37.4
Delivery system trackability and anatomic resemblance (A-ovine, C-human). Images (a) and (b) conventional vascular angiography. Image (c) cone beam angiography with three-dimensional reconstruction. Image (d) CT with three-dimensional reconstruction (Images b and d courtesy of American Heart of Poland)
An important difference in experimental models is the anatomical variants of the large vessels derived from the aorta compared to humans. In sheep, both carotid arteries arise from brachiocephalic trunk, whereas in swine the anatomy of aortic arch is similar to human with the brachiocephalic trunk and common carotid artery arising from the aortic arch (Figs. 37.1 and 37.4). This feature is particularly important in the testing of embolic protection devices.
Acute Evaluation of Valve Delivery and Deployment
For experimental models, the aortic root, valve architecture, and its components (leaflets, sinutubular junction, and coronary ostia annulus) present identical morphological features compared to humans (Figs. 37.1, 37.4, and 37.5). Due to its size and anatomical configuration, the sheep model is the most common model used for TAVR testing today. In this model, the aortic annulus size is comparable to humans except at the level of the sinutubular junction, where it is significantly bigger. In the ovine, the coronary ostia are located usually lower when compared to human and the right ostium is higher than the left. Basing on our experience the average distance from the annulus to right coronary ostium is 9.2 ± 1.9 and 8.4 ± 1.7 mm to the left coronary ostium. In the clinical setting this origins are considered low and at increased risk of coronary occlusion [9]; however, in the preclinical setting this is a very unusual event.
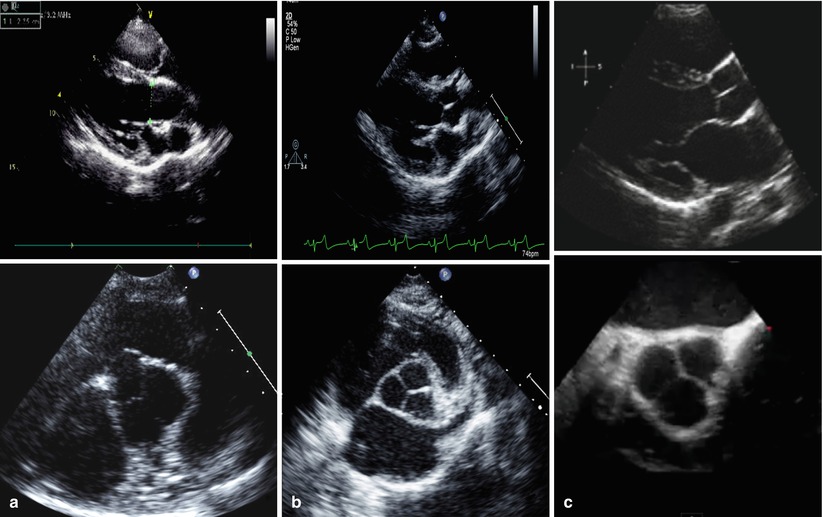
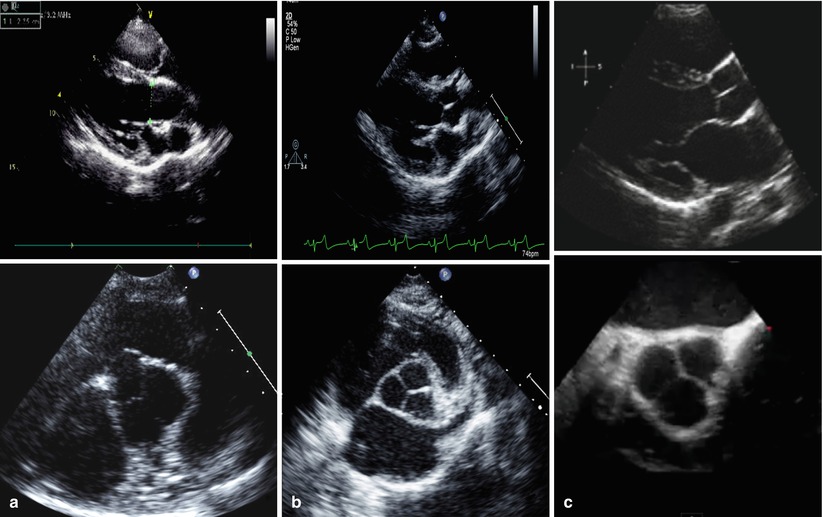
Fig. 37.5
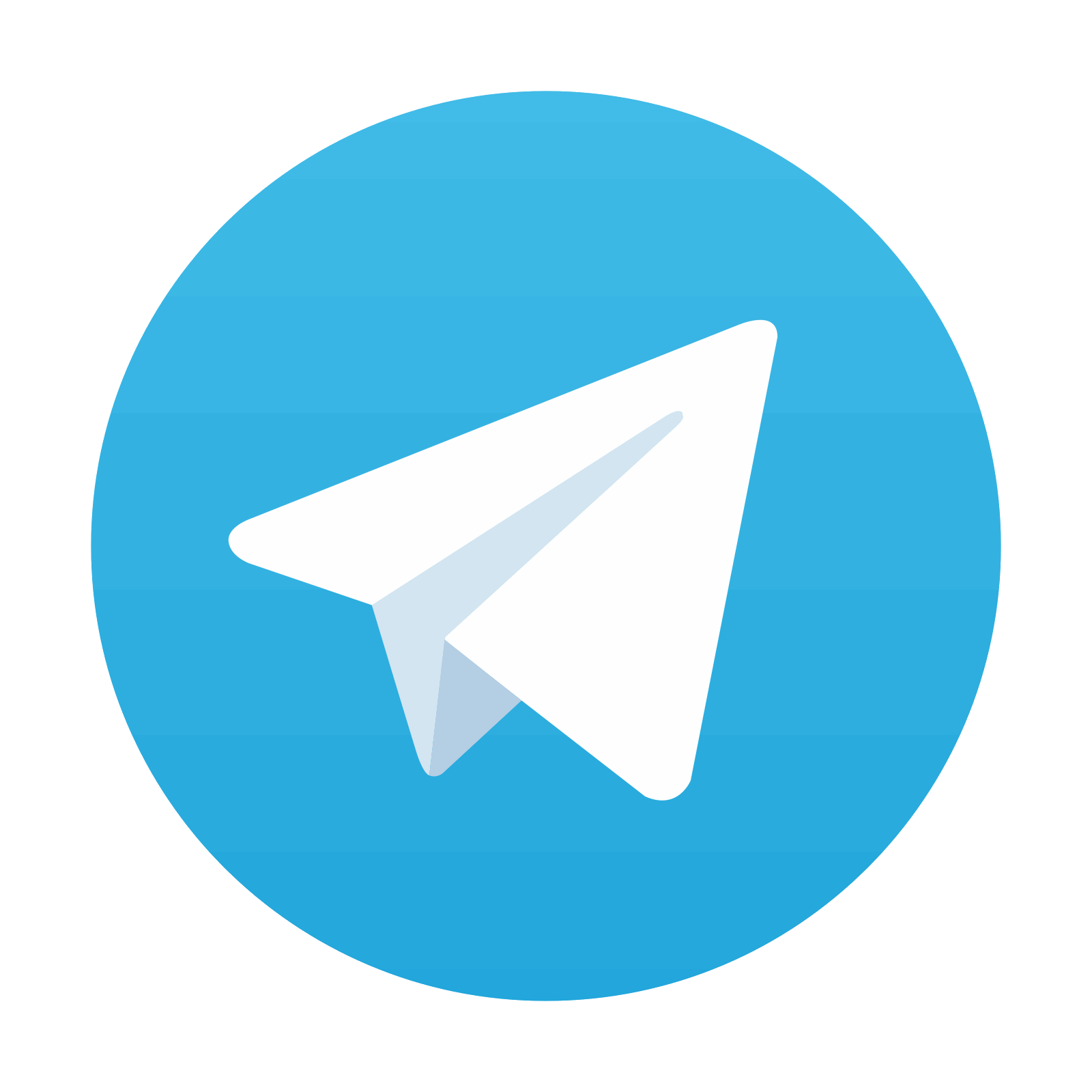
Comparative heart and valvular anatomy in long (top) and short axis (bottom) in echocardiography: (a) sheep, (b) swine, (c) human
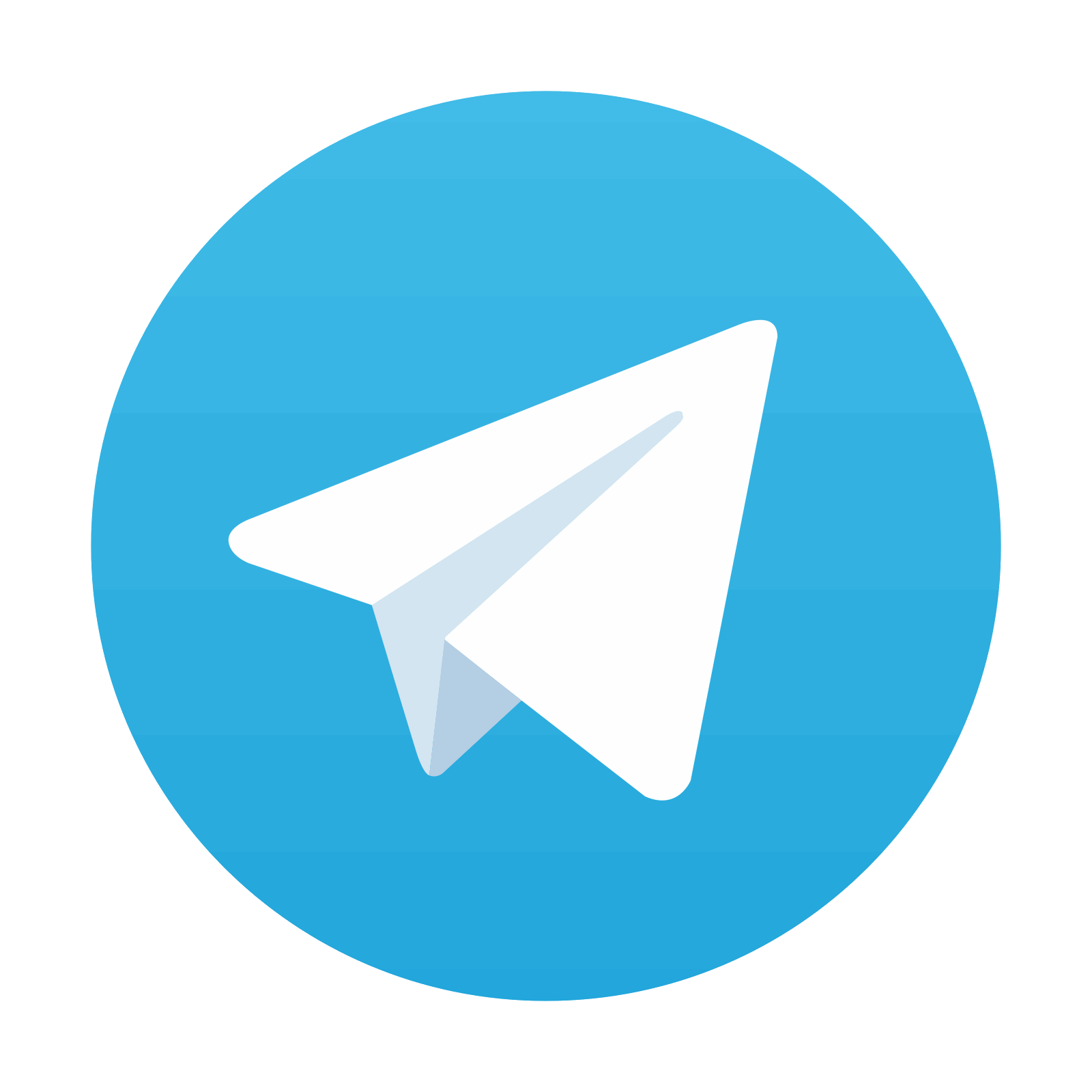
Stay updated, free articles. Join our Telegram channel

Full access? Get Clinical Tree
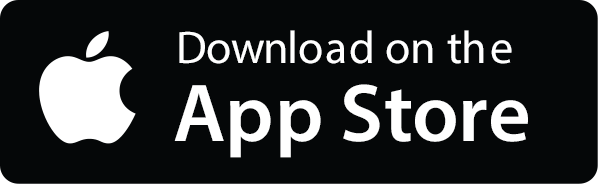
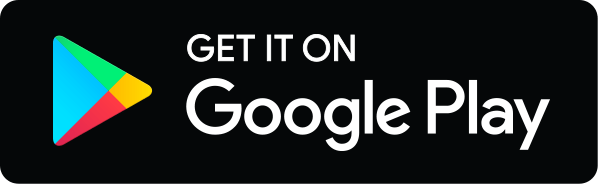
