1 Brain and Extra-axial Lesions
Computed tomography (CT) can provide rapid multiplanar imaging of the brain, meninges, and skull. The use of dynamic rapid data acquisition combined with bolus injection of iodinated contrast agents allows for assessment of blood perfusion rates of normal and abnormal brain tissue, as well as the generation of high-resolution CT arteriograms and venograms. CT has proven to be a powerful imaging modality in the evaluation of (1) neoplasms of the central nervous system, meninges, calvarium, and skull base; (2) traumatic lesions; (3) intracranial hemorrhage; (4) ischemia and infarction, particularly using CT perfusion studies; (5) infectious and noninfectious diseases; and (6) metabolic disorders.
The appearance of brain tissue depends on the milliampere second (mAs) and kilovolts peak (kVp) used, as well as the age of the patient imaged. Myelination of the brain begins in the fifth fetal month and progresses rapidly during the first 2 years of life. The degree of myelination affects the appearance of the brain parenchyma on CT. In adults, the cerebral cortex has intermediate attenuation that is slightly higher relative to normal white matter. For infants younger than 6 months, differentiation of white matter relative to gray matter is limited secondary to the immature myelination of brain tissue. Myelination proceeds in a predictable and characteristic pattern with regard to locations and timing. These changes are more optimally seen with magnetic resonance imaging (MRI) than with CT.
Various pathologic processes can affect the attenuation properties of the involved tissue or organ. For example, intraparenchymal hemorrhage can have variable appearances in the brain depending on the age of the hematoma, oxidation states of the iron in hemoglobin, hematocrit, protein concentration, clot formation and retraction, location, and size. Oxyhemoglobin in a hyperacute blood clot has ferrous iron. After a few hours during the acute phase of the hematoma, the oxyhemoglobin loses its oxygen to form deoxyhemoglobin. Deoxyhemoglobin also has ferrous iron, although it has unpaired electrons. Later in the early subacute phase of the hematoma, deoxyhemoglobin becomes oxidized to the ferric state, methemoglobin. Initially, red blood cells in the clot are intact. In the late subacute phase, breakdown of the membranes of the red blood cells results in extracellular methemoglobin. In the chronic phase, methemoglobin becomes further oxidized and broken down by macrophages into hemosiderin.
The CT features of subdural hematomas are variable, although the appearances can progress in patterns similar to intraparenchymal hematomas. Acute subdural hematomas often have low-intermediate attenuation.
Other lesions that can result in zones of low attenuation are dermoids (intact or ruptured), teratomas, lipomas, and cystic structures with low protein concentration or cholesterol.
Nonhemorrhagic pathologic processes tend to decrease the attenuation of the involved tissues. Such processes include ischemia, infarction, inflammation, infection, demyelination, dysmyelination, metabolic or toxic encephalopathy, trauma, neoplasms, gliosis, radiation injury, and encephalomalacic changes. Exceptions to this phenomenon include neoplasms with high nuclear to cytoplasmic ratios (e.g., medulloblastomas and small cell lymphomas), fluid collections with high protein or mineral concentrations (e.g., colloid cysts and craniopharyngiomas), and lesions with fine or clumplike calcifications (e.g., ependymomas, meningiomas, and metabolic disorders such as hypoparathyroidism and Fahr disease).
Of the various human body tissues, the brain is the least tolerant of ischemia. A lack of sufficient blood flow to the brain for approximately 5 seconds results in loss of consciousness and for several minutes can result in irreversible cerebral ischemia and infarction. For normal brain function, cerebral blood flow (CBF) must be maintained at a constant rate to deliver oxygen and glucose as well as to remove CO2 and metabolic waste products. Maintenance of CBF is critical for neuronal function. With arterial occlusion, the loss of normal neuronal electrical activity occurs within seconds after arterial occlusion. Cellular death is dependent on the duration and magnitude of ischemia, the metabolic vulnerability of specific anatomical sites, and the oxygen content of blood. CT is important in the detection of cerebral infarction and for the presence of associated hemorrhage, which may preclude the use of thrombolytic therapy. Another clinical application of CT for stroke is a technique referred to as CT perfusion, which utilizes rapid infusion of intravenous (IV) iodinated contrast, and will be discussed at the end of this section.
Areas where there is a breakdown of the blood–brain barrier from pathologic disorders can be evaluated with iodine-based IV contrast agents. Leakage of these agents through the blood–brain barrier results in contrast enhancement localized to the involved pathologic regions. Contrast-enhanced CT images are an important portion of most imaging examinations of the head. In addition to pathologically altered intracranial tissues, contrast-enhancement can be normally seen in veins, the choroid plexus, the pituitary gland, and pineal gland. For this book, the attenuation of the various entities will be described as low, intermediate, high, or mixed and whether there is contrast enhancement or not.
Intracranial lesions are typically classified as being extra- or intra-axial. Extra-axial lesions arise from the skull, meninges, or tissues other than the brain parenchyma. They are characterized as being within epidural, subdural, or subarachnoid spaces or compartments. Lesions involving the meninges can be further categorized as involving the dura mater (e.g., with benign postoperative dural fibrosis) or involving the leptomeninges (pia and arachnoid). Abnormalities of the meninges are often best seen after the IV administration of contrast material. Dural enhancement usually has a linear configuration, whereas pathology involving the leptomeninges appears as enhancement within the sulci and basilar cisterns. Enhancement of the leptomeninges is usually related to significant pathology, such as neoplastic or inflammatory diseases.
Intra-axial lesions are located in the brain parenchyma or brainstem. Differential diagnoses of extra-axial and intra-axial masslike lesions are presented in Tables 1.2 , 1.3 , 1.4 , and 1.5 . Intra-axial masslike lesions are presented according to location: supra- versus infratentorial. Infratentorial neoplasms are more common in children and adolescents than adults. During childhood, intra-axial tumors such as astrocytomas, medulloblastomas, ependymomas, and brainstem gliomas are the most common neoplasms. In adults; metastatic lesions and hemangioblastomas are the most common intra-axial infratentorial tumors, and acoustic schwannomas and meningiomas are common extra-axial infratentorial neoplasms. Infratentorial lesions are discussed in Tables 1.4 and 1.5 .
Multidetector CT is an excellent imaging modality for evaluation of the skull base, orbits, nasopharynx, oropharynx, and floor of the mouth because of its multiplanar imaging capabilities and high spatial resolution. CT is a useful method for imaging the location and extent of osseous lesions at the skull base, such as metastatic tumors, myelomas, chordomas, and chondrosarcomas.
CT can be used for the evaluation of congenital and developmental anomalies of the brain, such as semilobar holoprosencephaly, septo-optic dysplasia, schizencephaly, gray matter heterotopia, cortical dysplasia, unilateral megalencephaly, and Dandy-Walker malformation.
Diseases of white matter are classified into two major groups, dysmyelinating and demyelinating diseases. Dysmyelinating diseases, also known as leukodystrophies, are a group of disorders resulting from enzyme deficiencies that cause abnormal formation and metabolism of myelin. Demyelinating diseases are a group of disorders in which myelin is degraded or destroyed after it has formed in a normal fashion.
Abnormalities involving the lateral, third, and fourth ventricles, as well as the cerebral aqueduct, are well seen with CT because of the difference of attenuation between the brain parenchyma and cerebrospinal fluid (CSF). The production of CSF occurs in the choroid plexus within the ventricles. CSF circulates from the lateral ventricles through the foramina of Monro into the third ventricle. The third ventricle communicates with the fourth ventricle via the cerebral aqueduct. CSF from the fourth ventricle enters into the subarachnoid space through the foramina of Luschka and Magendie. Mass lesions along the CSF pathway can result in obstructive hydrocephalus with dilation of the ventricles proximal to the blockage.
Some asymmetry of the lateral ventricles can be seen normally. Altered morphology of the ventricles can result from various congenital anomalies (e.g., holoprosencephaly, septo-optic dysplasia, unilateral hemimegalencephaly, gray matter heterotopia, and Dandy-Walker malformation), as well as from distortion from intra- or extra-axial mass lesions.
The sizes of sulci can vary depending on multiple variables, such as age, congenital malformations, vascular abnormalities (e.g., cerebral infarcts, Sturge-Weber syndrome, and arteriovenous malformations [AVMs]), intra- or extra-axial mass lesions, hydrocephalus, and inflammatory diseases. Sulci should have CSF attenuation within them. The presence of contrast enhancement within the sulci and basilar cisterns is usually associated with pathology, such as inflammatory or neoplastic disease. Acute subarachnoid hemorrhage is typically seen as amorphous zones with high attenuation within the sulci and cisterns near the site of bleeding.
CT angiography (CTA) is a powerful imaging modality for evaluating normal and abnormal blood vessels. CTA has proven to be clinically useful in the evaluation of intracranial arteries, veins, and dural venous sinuses. Pathologic processes involving intra cranial blood vessels, such as aneurysms, AVMs, arterial occlusions, and dural venous sinus thrombosis, can be seen with CTA.
CT perfusion is a relatively new technique using dynamic IV infusion of contrast to measure CBF, cerebral blood volume (CBV), and mean transit time (MTT) of contrast enhancement to selected volumes of interest in the brain. CT perfusion has major clinical use in the evaluation of cerebral infarcts and adjacent zones of decreased perfusion (penumbra and oligemic areas) at risk for progression to infarction. Maintenance of CBF is critical for neuronal function. With arterial occlusion, loss of normal neuronal electrical activity occurs within seconds after arterial occlusion. Cellular death is dependent on the duration and magnitude of ischemia, the metabolic vulnerability of specific anatomical sites, and the oxygen content of blood. Normal CBF ranges from 50 to 60 mL/100 g/min. When the CBF is reduced to 15 to 20 mL/100 g/min for several hours (mild to moderate hypoxia), spontaneous and evoked neuronal electrical activity decreases significantly secondary to ischemia, although it can be reversed with reperfusion with CBF > 50 mL/100 g/min. With severe hypoxia/anoxia resulting from CBF < 10 mL/100 g/min, cellular membrane depolarization and ischemia leading to brain infarction may occur in several minutes.
When thrombotic or embolic arterial occlusions occur, CBF in the involved brain tissue is usually heterogeneous, with a central core showing the greatest reductions in CBF causing irreversible cell damage and infarction and a surrounding zone (referred to as the salvageable penumbra) that may have moderate reduction in CBF resulting in ischemia that may be reversible with reperfusion. The penumbra typically shows loss of neuronal electrical activity without immediate anoxic depolarization, as well as loss of autoregulation. If reperfusion does not occur, the penumbra will progress to infarction. An oligemic zone of mildly reduced CBF may also be seen surrounding the penumbra, which is less vulnerable to infarction than the penumbra. Thrombolytic medication can be useful and beneficial when it results in timely reperfusion to the penumbra and oligemic zones. Estimating the sizes of the penumbra and oligemic zones can be done in the acute setting with dynamic contrast-enhanced CT. CT perfusion with iodinated contrast delivered as an IV bolus can use the linear relationship between contrast concentration and attenuation to directly calculate and quantify CBF, CBV, and MTT for sites of ischemia and infarction in the brain prior to thrombolytic treatment (Fig. 1.1).
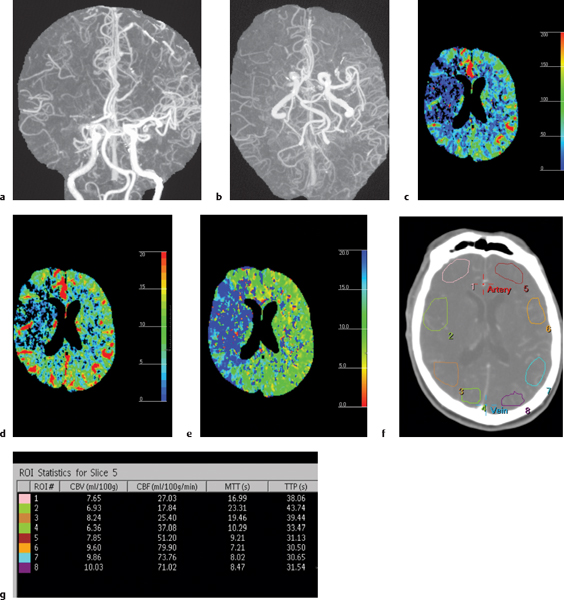
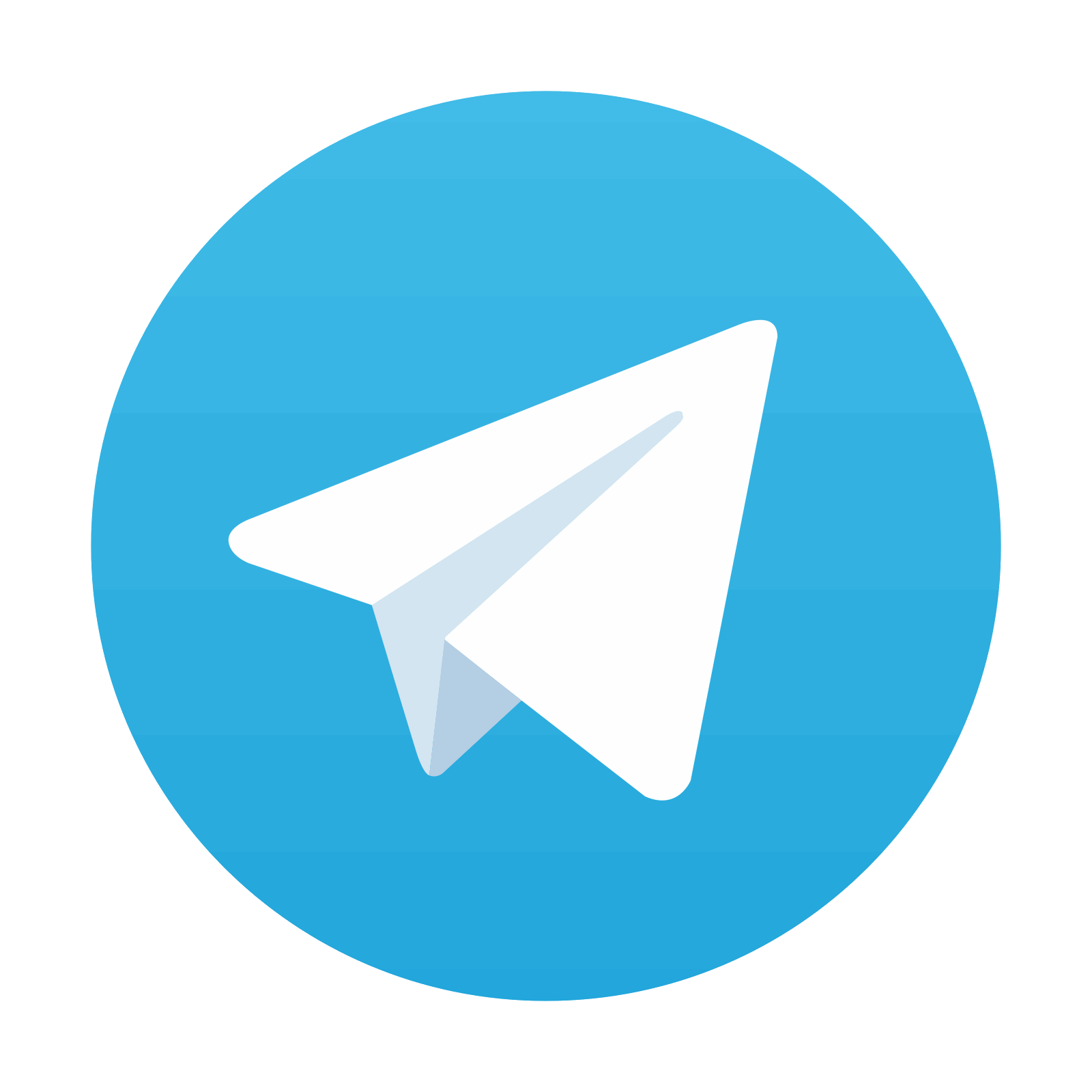
Stay updated, free articles. Join our Telegram channel

Full access? Get Clinical Tree
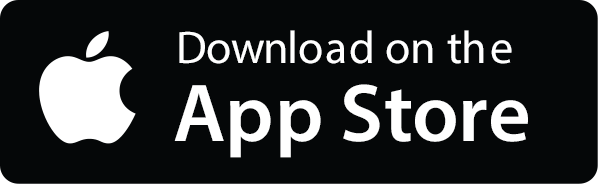
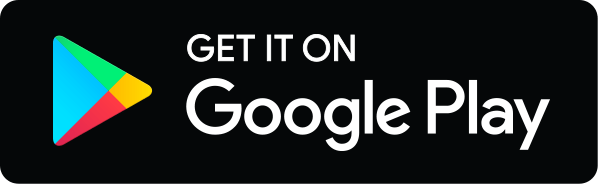