Fig. 1
Source areas of individual subjects showing a positive non-zero signal (P < 0.001) within those regions of interest (ROI) and time of interest (TOI) where activation strengths differed significantly between fluent speakers (left) and stutterers (right). The symbols are filled with grey in that subject group which showed on average less activation in each ROI/TOI (Salmelin et al. 2000)
The third MEG study performed on stuttering subjects was by Walla et al. This MEG study looked at the lack of focused anticipation of verbal information in AWS during visually presented single words (Walla et al. 2004). When fluent readers spoke the word aloud, immediately after word presentation, neural activation (in the normalized MEG waveforms) was seen in the motor speech area before speech onset. They used a two-dipole model to approximate the sources for this pre speech activity. Pre speech brain activity was not detected in AWS. Indicating that this brain activity (also named Bereutschafts field 2) might reflect preparatory activity known to occur for many other voluntary movements, and may be the link to disfluent speech in AWS.
A fourth MEG study of speech perception indicated language processing in the auditory modality differs for AWS and normally fluent readers. Biermann-Ruben et al. (2005), detected alternate language processing pathways during speech perception prior to overt repetition of a spoken word and sentence, and a sentence transformation task. AWS had greater activation of left inferior frontal areas, thought to be important for speech preparation, during the temporal window of 95–145 ms post-word and sentence onsets. In addition, between 315 and 1,000 ms post-stimulus, activations of the right rolandic areas, thought to be involved in sensorimotor processing, were larger for single-word compared to sentence tasks for the fluent readers, but the opposite pattern was seen for the AWS. Their results suggest that activation in the left inferior frontal and right rolandic areas in AWS differs from that in controls during speech perception. These findings may reflect differences due to the paradigm set up as there was a delay prior to speaking. In a previous MEG study in fluent readers, which did not require speaking following speech perception, also did not elicit activation in the temporo-parietal, inferior-frontal, and rolandic areas (Helenius et al. 1998). This suggests the possibility that the differences between the AWS and fluent readers may be just due to activations for preparing to speak.
Our group (Bowyer et al. 2010) identified cortical activity in Wernicke’s area (posterior section of the superior temporal gyrus) activated at the same time in controls and AWS. Activation was located in the supramarginal gyrus (SMG) in the latency interval 230 ± 20 ms across all 14 subjects. This indicated that comprehension was normal in both groups during the early language components in a verb generation task. Visual activation was detected in all subjects at 101 ± 10 ms after stimulus onset during all language task runs, indicating that visual processing was normal across all subjects. Using a one word speaking aloud task differences were noted in Broca’s activation. AWS had delayed motor speech activation (434 ± 20 ms) compared to normal fluent readers (378 ± 36 ms) in the inferior frontal gyrus [p < 0.0001]. Also using an overt Verb Generation task AWS had delayed motor speech activation (450 ± 22 ms) compared to normal fluent readers (350 ± 29 ms) in the inferior frontal gyrus [p < 0.0001] (Fig. 2).
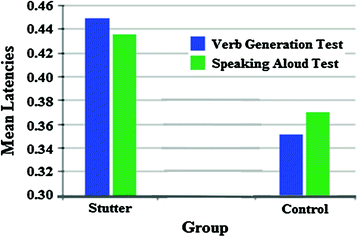
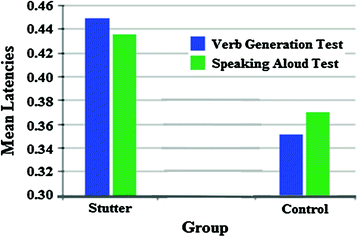
Fig. 2
Mean latencies of the groups during the verb generation task and the speaking aloud task. Broca’s activation in AWS was significantly higher than that of control subjects
This study used a current distribution technique [MR-FOCUSS (Moran et al. 2005)] to localize brain activity during these language tasks. This MEG technique is different from the ECD method used in the previous MEG studies in that it allows the identification of simultaneous brain activity that is occurring across the brain to be displayed, where as the ECD method only allows one location in the brain to be displayed. Figure 3 depicts the MR-FOCUSS results for one individual AWS and one fluent reader. The inferior frontal gyrus in the Left hemisphere is active in both subjects but at different latencies. The MEG waveforms depict a large amplitude wave at ~100 ms representing visual processing. The line is located at the latency where Broca’s area (inferior frontal gyrus) was active.
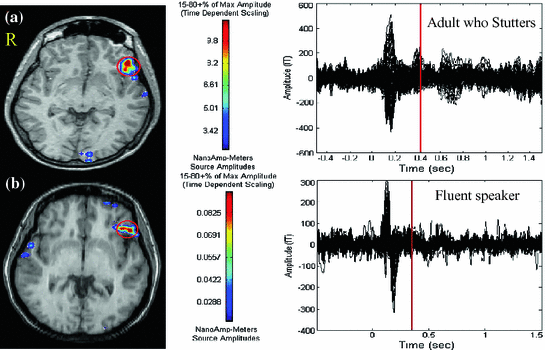
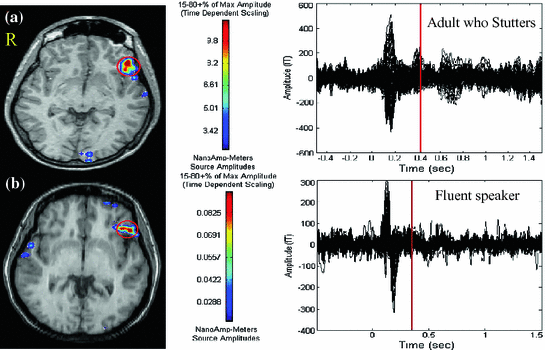
Fig. 3
MEG results during the overt verb generation task. Broca’s area activity is indicated by a circle on the MRI. Scale is in nanoAmp-meters. a Maximum peak activity was seen at 440 ms in the evoked MEG waveform after word onset in this AWS. b Maximum activity was seen at 336 ms after word onset in this fluent reader. AWS had a much high current distribution seen in Broca’s area compared to fluent readers
Two recent MEG studies have investigated the auditory system in AWS which has implications for treatment, which we will discuss further later in this chapter. Kikuchi et al. (2011) found auditory sensory gating (P50m suppression) was impaired in the left hemisphere during basic auditory input processing and that some error signals in the auditory cortex could result in abnormal speech processing. The tonotopic organization in the right hemisphere of AWS is expanded compared with that of the controls, along with a significant increase in the gray matter volume of the right superior temporal gyrus, consistent with the right tonotopic expansion. This study used the ECD model to localize brain activity. They hypothesize that the functional and structural reorganization of the right auditory cortex maybe a compensatory mechanism for impaired left auditory cortex function in AWS.
Beal et al. (2011) found speech induced suppression of auditory evoked fields in children who stutter (CWS). They examined the auditory P50m response in children as it most likely reflects a motor-to-auditory relation. This group used an event related vector beamformer to localize brain activation. See Fig. 4. Both CWS and those that do not stutter demonstrated speech-induced suppression of the auditory P50m. However, CWS had a delayed auditory M50 peak latency to vowel sounds compared to children who do not stutter indicating a possible deficiency in their ability to efficiently integrate auditory speech information for the purpose of establishing neural representations of speech sounds.
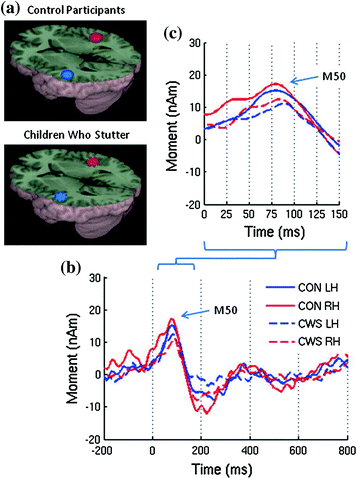
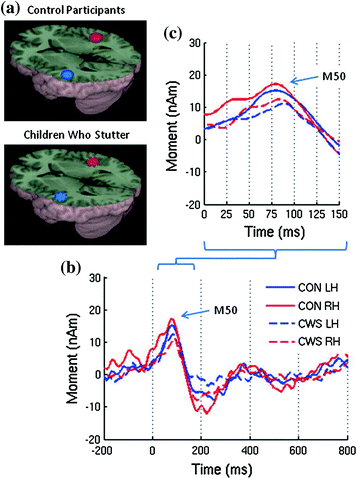
Fig. 4
a Group averaged source images of the auditory evoked magnetic fields for the speak vowel task overlaid on the MNI canonical brain. b Group averaged source magnitude variations from 200 ms prestimulus to 800 ms post stimulus corresponding to those sources and c a detailed view of the early components (Beal et al. 2011)
The millisecond temporal resolution and millimeter spatial resolution of these seven MEG studies provided significant information on how the stuttering brain is processing higher order cognitive language functioning differently than fluent speaking control subjects. Though these studies all used either a single visual or auditory word or sentence task, the results provided a more integrated image of the neuronal processing that underlies stuttering. Advances in MEG signal processing and artifact removal techniques make it easier to collect realistic speech and analyze the location of corresponding brain activity more effectively than 10 yeas ago. The refinement of protocols to look at continuously connected speech will also provide an abundant amount of data to investigate in more detail the underlying brain regions activated in the stuttering process. There is currently no other imaging technique available providing combined high temporal resolution and high spatial resolution in a safe, noninvasive imaging modality for studying children.
1.5 Studying PWS Across Their Development
When studying stuttering it is extremely important to understand how age effects the results. Researchers have been suggesting for many years that drawing conclusions about the cause and nature of developmental stuttering in CWS from studies of AWS is unwise (Conture and Kelly 1991; Yairi 1993) because it disregards the “influence of development, learning history, and experience” (Conture 1990).
Although limited, there have been some studies exploring the neural processes involved in children. In a study examining CWS (Chang et al. 2008), differences were found in Broca’s area but no differences were found in the right hemisphere regions. These findings supported the suggestions from many studies completed with AWS that right hemisphere involvement may be the result of compensatory reactions (De Nil et al. 2000; De Nil and Kroll 2001; Fox and Raichle 2007; Chang et al. 2008; Loucks et al. 2011). Weber-Fox et al. (2008) found that neural processes related to phonological processing in CWS were different than children who do not stutter (CWNS). It is also noteworthy that, when compared to a similar study done with AWS (Weber-Fox et al. 2004), the differences were qualitatively different and more pronounced in the CWS.
Although the research completed by Weber-Fox et al. (2008) and Chang et al. (2008), are steps in the right direction of exploring stutter in children, both studies were completed with school-aged children, with the youngest being 9 years old. The onset of stuttering typically occurs in young children when rapid cognitive, linguistic and motor development is occurring (Fox et al. 2000). This stage typically occurs during the preschool years, between the ages of two and five (Ambrose and Yairi 1999). To explore the underlying neurophysiology of developmental stuttering and the likely causes of it, it is important to study children close to the point of recovery or persistency (Loucks et al. 2011) to avoid contamination “by time-related adjustments to internal and external responses” (Yairi 1993). Many have avoided completing neuroimaging studies with young children, however, because there are many challenges to studying young children, including a reluctance to participate and a shorter time period for tolerance of the protocol (Loucks et al. 2011).
When looking at the above research, it becomes clear that exploring stuttering across the continuum of development is essential to tell the story of the developmental and cortical contributions to stuttering. Studying different age groups in different stages of stuttering will give comparative data on the neurophysiology of stuttering. That data may then be evaluated to give a better picture of the causal versus resultant neural processes involved in stuttering.
1.6 Network Activity Seen in PWS
Recently resting state MEG data has been used to evaluate the default mode network (DMN) in different disorders such as Epilepsy (Elisevich et al. 2011). The DMN is a network of brain regions that are active when the individual is not focused on the outside world and the brain is at wakeful rest. fMRI and MEG provide neuroimaging techniques that can look at the source location of the coherent brain oscillations during rest. Although the DMN has not been a main area of focus for stuttering research studies in the past, high areas of coherent activity in the inferior frontal gyrus and auditory cortex during the resting state in AWS was hypothesized to create an abnormal DMN and cause decreased efficiency in accessing those areas for AWS (Bowyer et al. 2010).
Guitar (1998) suggested that atypical neurological organization predisposes certain people to stuttering, however, those atypical organizations do not appear to be consistent from person to person. In fact, he hypothesizes that patterns of neural activity develop in unique ways and may account for the wide variety of symptoms seen in people who stutter.
Our group investigated the resting state, when no langauge processing was occurring, and found Broca’s areas had significantly higher coherence in PWS (0.31 ± 0.08) compared to controls (0.13 ± 0.04) [p < 0.0001]. Figure 5 displays one PWS and one fluent speaking control subject’s resting state results. These MEG resting state results possibly indicate continuous brain activity is occurring in the motor speech area thus providing competition for brain resources resulting in stuttering.
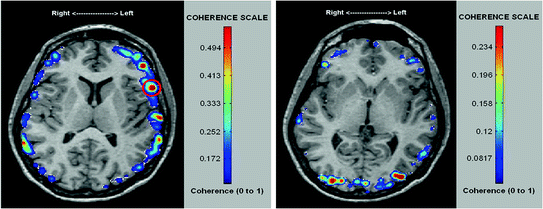
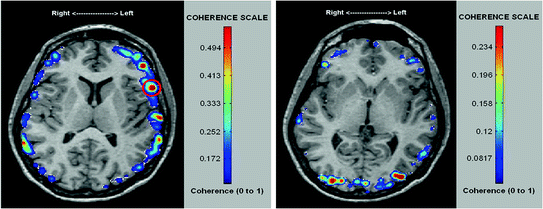
Fig. 5
a DMN coherence of a PWS. Highly coherent activity was found in Broca’s area (Brodmann’s Area 45) during resting state. b DMN coherence of a fluent speaking control subject finds little or no coherent activity in Broca’s area
This finding was supported by a recent fMRI resting state study in 44 male AWS compared to 46 aged matched fluent speakers. (Xuan et al. 2012). Increased low frequency fluctuations were found in the left Inferior frontal gyrus as well as in the Left auditory and bilateral prefrontal cortices in PWS. This study also found that functional connectivity within anterior and posterior speech- and language-associated areas was increased in PWS compared to controls.
However, more extensive MEG studies will be needed to further explore how these results can be used for diagnois and detection of treatment responses. The nice thing about this type of task is that even infants can preform a task where they lay quietly for a few minutes with their eyes open.
1.7 Treatments
Currently, there are no cures for stuttering, although pharmacological and clinical avenues for potential treatments are being pursued (Bothe et al. 2006a, b; Blomgren 2010; Ratner 2010). The nature of the treatment will differ, based upon a person’s age, communication goals, and other factors. Therapy for stuttering is primarily done by a certified speech-language pathologist.
For very young children, early treatment may prevent developmental stuttering from becoming a lifelong problem. Certain strategies can help children learn to improve their speech fluency while developing positive attitudes toward communication. Health professionals generally recommend that a child be evaluated if he or she has stuttered for at least 6 months, exhibits awareness of the stuttering and/or struggle behaviors associated with stuttering, or has a family history of stuttering or related communication disorders.
The two therapy techniques most commonly used for pre-teens though adulthood focus on strategies for improved fluency (fluency enhancing techniques) and strategies for modifying the stutter (stuttering modification techniques). It is widely upheld that a more integrated approach, utilizing a combination of the two techniques to address the behaviors, thoughts and feelings associated with stuttering, is the best practice for clinicians to treat PWS (Bennett 2006).
Drug therapies with Risperidone and Asenapine, which are dopamine-antagonist agents, have been shown to moderately improve a patients’ stuttering frequency (Maguire et al. 2011; Tavano et al. 2011). However, better treatment methods are being sought as dopamine antagonists can lead to severe side-effects including Parkinson’s disease and other conditions characterized by dopamine-deficiency.
Multiple studies have affirmed that choral speech (speaking in unison with others) and singing can greatly reduce the frequency of stuttering in affected patients (Howell et al. 2004; Toyomura et al. 2011
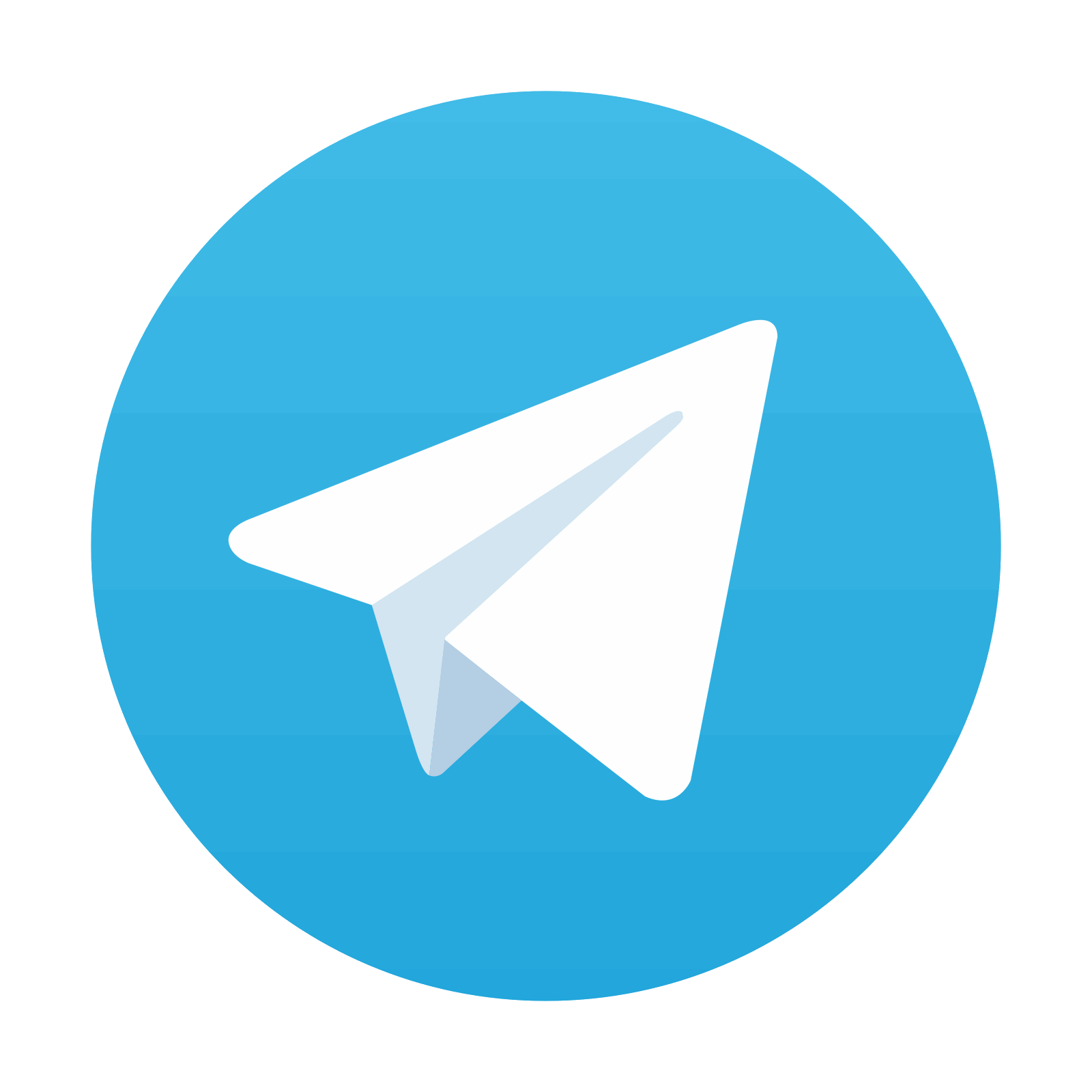
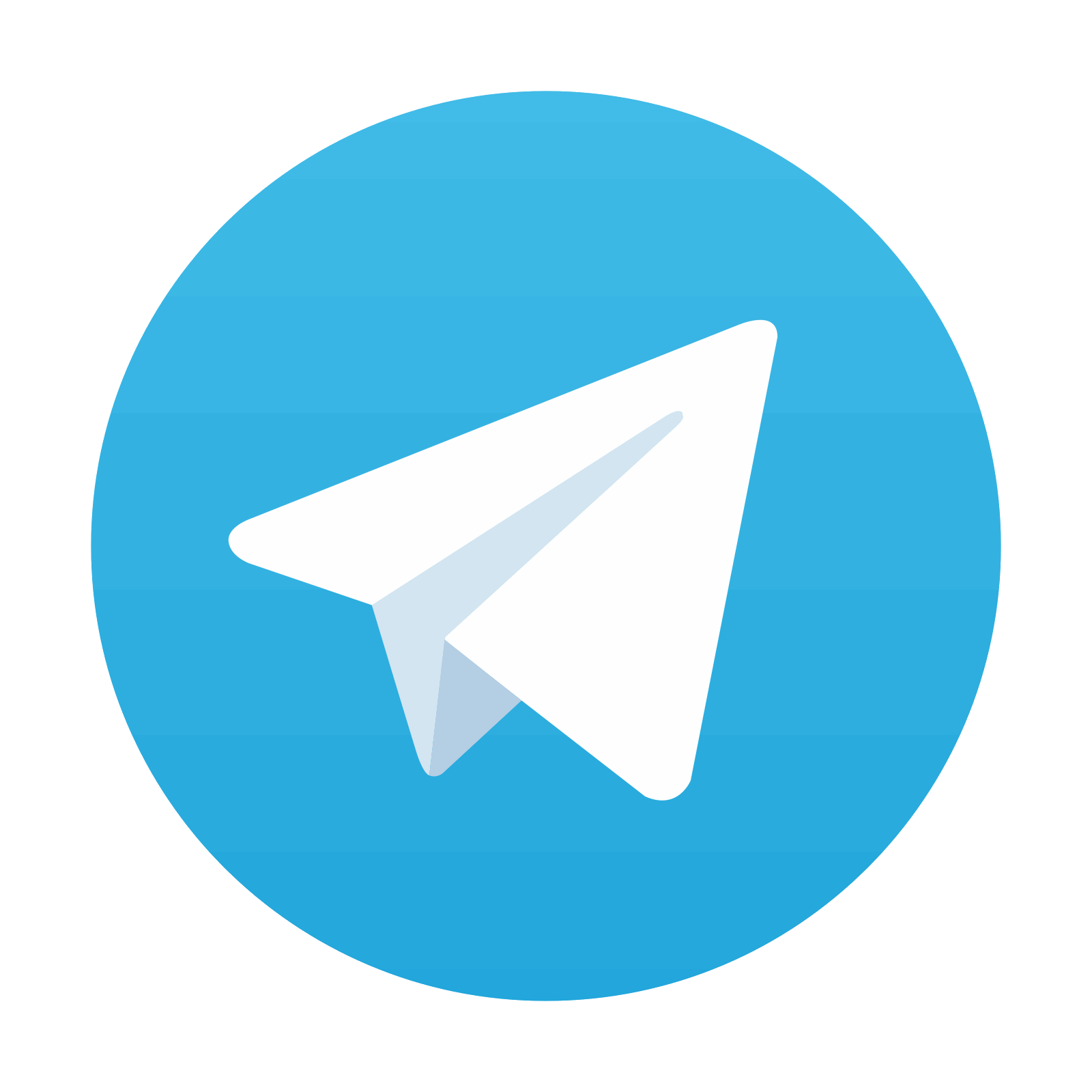
Stay updated, free articles. Join our Telegram channel

Full access? Get Clinical Tree
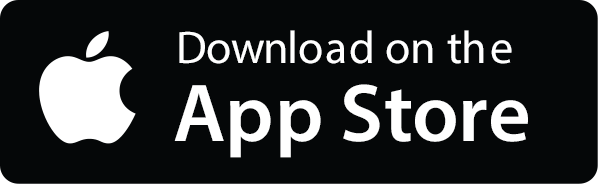
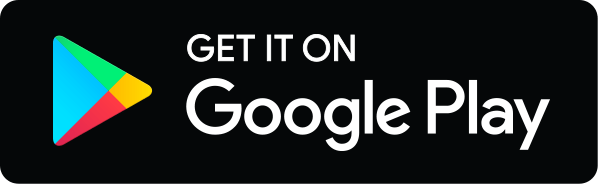