Fig. 44.1
68Ga-DOTATOC PET scan of a patient with extensively metastasized neuroendocrine lung tumor, who also had a small brain metastasis (red arrow) as well as an ossal lesion in the skull (yellow arrow) (Courtesy of Valentina Ambrosini, Nuclear Medicine, Azienda Ospedaliero-Universitaria di Bologna, Policlinico S. Orsola-Malpighi, Bologna, Italy)
44.8 Metabolic Imaging
18F-fluorodeoxyglucose (18FDG), which is an important PET tracer in oncology, has a limited role for the detection of brain metastasis due to a high metabolic rate of glucose in the healthy brain which results in a strong background signal (Kruger et al. 2011). The potential role of 18FDG PET scanning for the detection of neuroendocrine brain metastasis will likely be even more limited. In contrast to many other malignancies, well-differentiated GEP neuroendocrine tumors (NETs) generally have a low glucose metabolism (Adams et al. 1998; Belhocine et al. 2002). For the primary staging of neuroendocrine tumors, 18F-fluorodeoxyglucose (18FDG) PET scanning has limited value due to the only moderately increased glucose metabolism of most GEP NET. Therefore, 18FDG PET is probably not suited as a staging tool for the detection of suspected brain metastasis in patients with neuroendocrine tumors (Adams et al. 1998; Pasquali et al. 1998).
Many neuroendocrine tumors and their metastases have an increased synthesis and secretion of hormones and neurotransmitters. Serotonin, catecholamine, and histamine are the most common examples of hormones secreted by these tumors (Jager et al. 2008). The increased biosynthesis of catecholamines and serotonin in neuroendocrine tumors enables imaging with specific amine precursors. In this chapter, we will shortly discuss 6-18F-L-3,4-dihydroxyphenylalanine (18F-DOPA), a PET tracer for the catecholamine pathway, and β-11C-5-hydroxy-L-tryptophan (11C-5-HTP), a PET tracer for the serotonin pathway.
44.9 Catecholamine Pathway
Catecholamines act as neurotransmitters in the brain or as hormones in the body, e.g., via α- and β-adrenergic receptors located on vessels and internal organs. For the synthesis of catecholamines, phenylalanine and intermediate products such as L-3,4-dihydroxyphenylalanine (L-DOPA) are taken up via system L large amino acid transporters (LAT). These precursors are intracytoplasmatically decarboxylated to dopamine by the enzyme aromatic amino acid decarboxylase (AADC). The resulting products are taken up by vesicular mono amino transporters (VMAT) for intravesicular storage. Within vesicles, dopamine can be further metabolized to noradrenalin and adrenalin via specific enzymatic pathways. These vesicles are capable of releasing the end products dopamine, noradrenalin and adrenaline in the extracellular environment. Selective reuptake systems, e.g., dopamine and noradrenalin transporters, are capable of transporting released hormones back into the cell.
Both 6-18F-L-3,4-dihydroxyphenylalanine (18F-DOPA) and 6-18F-dopamine are available as tracers for the catecholamine pathway. However, currently no data exist on the use of 6-18F-dopamine for imaging of brain tumors or their metastases, thus this chapter will be limited to 18F-DOPA.
18F-DOPA can be produced via two different synthesis routes: regioselective fluorodestannylation (electrophilic fluorination) and nucleophilic fluorination, which is a multistep procedure. This last synthesis procedure is more time-consuming but has the advantage of the ready availability of large quantities of no-carrier-added 18F-fluoride (Lemaire et al. 1994; Jager et al. 2008).
The tracer 18F-DOPA was developed and used for initial human studies at McMaster University (Hamilton, ON, Canada) during the 1970s and 1980s (Garnett et al. 1983). In the early years after its development, this tracer was solely used for imaging of the dopaminergic system of the brain. Only several years later, whole-body 18F-DOPA imaging was applied in patients with NETs and other tumors (Heiss et al. 1996; Hoegerle et al. 2001).
Although 18F-DOPA PET(/CT) imaging has been found to be a powerful tool for the detection of neuroendocrine metastases outside the brain, unfortunately no data are reported on the use of 18F-DOPA for the detection of such metastases within the brain (Koopmans et al. 2008). For other brain tumors, like glioma, 18F-DOPA has proven to be of value (Chen et al. 2006).
Since 18F-DOPA has become commercially available in many countries, its increased availability may lead to a better understanding of the place of 18F-DOPA PET in clinical decision making for brain metastases of NETs.
44.10 Serotonin Pathway
Serotonin is a monoamine neurotransmitter present in the brain, blood platelets, and the intestinal wall. In the brain it acts as a neurotransmitter and plays a role in the regulation of mood.
The serotonin pathway shares the LAT and VMAT systems for transport and synthesis with the catecholamine pathway. In both pathways, the enzyme AADC plays a key role in the final step for the synthesis of a functional neurotransmitter, which is serotonin in the serotonin pathway. After uptake via the LAT system, the amino acid tryptophan is used as a precursor for serotonin synthesis via the intermediate product 5-hydroxytryptophan (5-HTP). This precursor is decarboxylated to serotonin via the enzyme AADC. Serotonin is stored into vesicles via VMAT transporters for future extracellular release. Released serotonin is eventually metabolized to 5-hydroxyindole acetic acid (5-HIAA) which is secreted in the urine. The serotonin pathway is overactive in many NETs, which makes this pathway a good candidate for imaging with PET tracers (Koopmans et al. 2009).
The carbon-11-labeled tracer β-11C-5-hydroxy-L-tryptophan (11C-5-HTP) was developed in the 1980s in Uppsala, Sweden, and is thus far the only suitable tracer for the serotonin pathway. The synthesis of 11C-5-HTP is complex, demanding an on-site cyclotron for the production of the 11C isotope which has a half-life of only 20 min. The radiochemical synthesis of the tracer proceeds in two multienzyme steps (Bjurling et al. 1989; Neels et al. 2006). 11C-5-HTP is therefore only produced by a few centers in the world. Interpretation of brain images is mostly easy due to a very low background uptake, and at least in extracranial NET tumors, a high tracer uptake is noted (Koopmans et al. 2008).
Due to the limited availability of 11C-5-HTP and the limited number of patients scanned with this tracer, no data have been published on the efficacy of 11C-5-HTP PET for the detection of brain metastases of neuroendocrine tumors. However, because of the favorable biodistribution of this tracer, a good uptake in brain metastasis can be expected. Hopefully, data will become available concerning the suitability of this promising tracer for the detection of neuroendocrine brain metastases. If serotonin precursors could be labeled with 18F, this might result in worldwide tracer availability which would definitely be beneficial.
44.11 Conclusion
Due to the low incidence of neuroendocrine tumors and metastatic brain lesions of neuroendocrine tumors, inadequate data is available to determine the relative efficacy of nuclear medicine imaging techniques such as 68Ga-DOTATOC PET, 18F-DOPA PET, and 11C-HTP PET for the detection of brain metastases of neuroendocrine tumors. From the available data it can be argued that these techniques may be of great value, but MRI is currently the recommended imaging modality for the detection of neuroendocrine brain metastases. Further research is definitely warranted, though due to the low incidence of such metastases, we can only hope for better data.
References
Batson OV (1940) The function of the vertebral veins and their role in the spread of metastases. Ann Surg 112:138–149PubMedCentralPubMedCrossRef
Belhocine T, Foidart J, Rigo P, Najjar F, Thiry A, Quatresooz P et al (2002) Fluorodeoxyglucose positron emission tomography and somatostatin receptor scintigraphy for diagnosing and staging carcinoid tumours: correlations with the pathological indexes p53 and Ki-67. Nucl Med Commun 23:727–734PubMedCrossRef
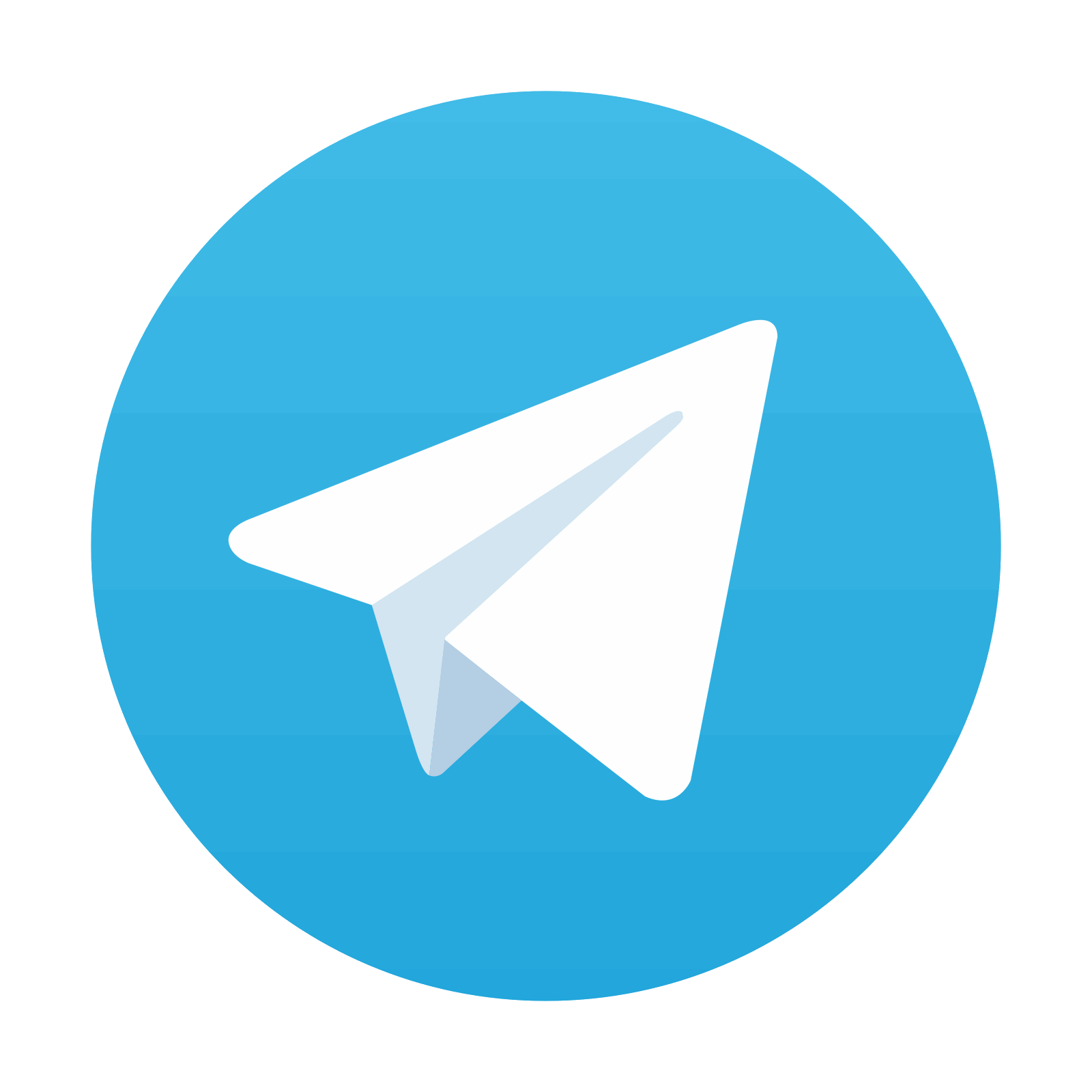
Stay updated, free articles. Join our Telegram channel

Full access? Get Clinical Tree
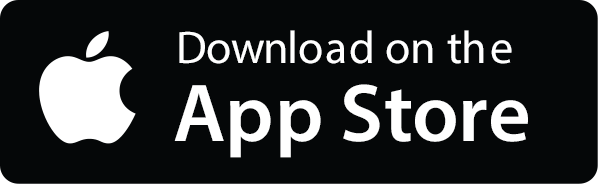
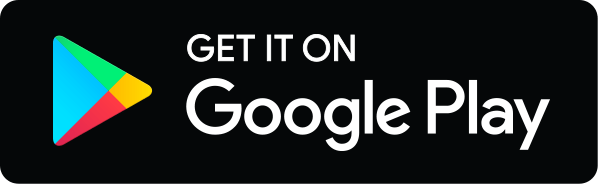