Patients hospitalized in the intensive care unit (ICU) often have multiple support lines and devices that need routine imaging evaluation by radiologists. In patients with cardiogenic shock or depressed cardiac function, mechanical circulation support devices are used in combination with medical therapies to improve patient outcomes and sometimes can stabilize patients for surgical intervention. This article discusses some of the more commonly encountered mechanical circulation devices seen in ICU patients, including intra-aortic balloon pumps, Impella devices, extracorporeal membrane oxygenation cannulas, and ventricular assist devices. Normal appearance and commonly encountered device-related complications that can be diagnosed on imaging are reviewed.
Key points
- •
Numerous technologies are available to provide cardiovascular support in the intensive care unit setting.
- •
Some of these devices provide temporary support, whereas others serve as a bridge to definitive therapy.
- •
Knowledge of their function and normal imaging appearance is necessary to recognize device complications that can be life threatening.
- •
Common complications with these devices include misplacement, migration, device failure, bleeding, vascular injury, stroke, and infection.
Introduction
Patients hospitalized in the intensive care unit (ICU) often have multiple support lines and devices that need routine imaging evaluation by radiologists. In patients with cardiogenic shock or depressed cardiac function, mechanical circulation support devices are often used in combination with medical therapies to improve patient outcomes and sometimes can stabilize patients for subsequent surgical intervention. This review discusses some of the more commonly encountered mechanical circulation devices seen in ICU patients and reviews both proper placement and commonly encountered device-related complications that can be diagnosed on imaging.
Intra-aortic balloon pump
Configurations and Physiology
The intra-aortic balloon pump (IABP) is a temporary mechanical circulatory support device used to augment coronary artery perfusion and reduce myocardial oxygen demand. First introduced in the late 1960s to treat cardiogenic shock, the IABP remains the most commonly used mechanical circulatory support device in the United States for acute myocardial infarction (MI), cardiogenic shock, various forms of cardiomyopathy, and in the perioperative cardiac surgery setting. The balloon, typically 20 to 25 cm in length, is mounted on a catheter and connected via external driveline to a control unit. The balloon inflates with helium during diastole, thus displacing blood and increasing arterial pressure in the ascending aorta to yield increased coronary perfusion pressure. Deflation in early systole decreases afterload and slightly reduces myocardial demand. The IABP can be placed by either femoral or axillary approach.
IABP use is contraindicated in patients with severe aortic regurgitation, as counterpulsation would significantly worsen regurgitant flow, and severe tachyarrhythmia may reduce IABP effect both by hindering timing of inflation/deflation and decreasing coronary filling time in diastole. Other contraindications include aortic dissection, severe peripheral vascular disease that prevents safe access, uncontrolled septicemia, and severe bleeding diathesis.
Normal Appearance
On frontal chest radiograph (CXR; Fig. 1 ), the IABP can be most readily identified by an approximately 4-mm linear metallic marker on each end of the balloon, although depending on the field of view, the lower marker may not be seen. For a femoral-approach IABP, the upper marker of the balloon should be located along the inferior aspect of the aortic arch or approximately 2 cm above the carina. If seen, the inferior marker typically projects over the T12 or L1 vertebral body. If the CXR is obtained in diastole, the balloon itself may appear as a tubular lucency projecting over the descending aorta (see Fig. 1 ). An axillary-approach IABP may be identified by the associated axillary sheath; positioning of the upper marker should be similar to femoral-approach devices.
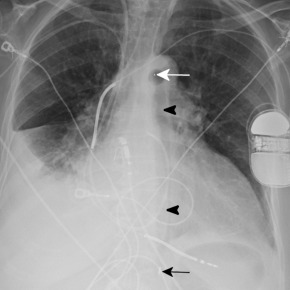
On nongated computed tomography (CT), the metallic markers will be evident, and the balloon will typically appear as a linear or gas-filled structure depending on the cardiac cycle. With electrocardiogram (ECG)-gating, the balloon will be more clearly cylindrical, and the process of inflation and deflation may be observed through different phases of the cardiac cycle ( Fig. 2 ).
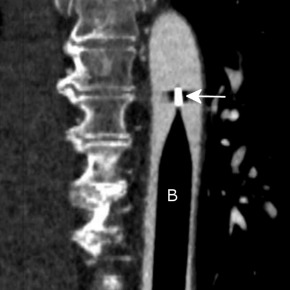
Complications
The most common complication is malposition of the IABP, which may occur in up to 50% of patients. High balloon malposition ( Fig. 3 ) may result in cycle-dependent occlusion of the arch vessels with extremity or cerebral diastolic hypoperfusion, whereas low balloon malposition ( Fig. 4 ) yields less efficient counterpulsation and may result in occlusion of the renal arteries, celiac, and mesenteric axes with distal organ diastolic hypoperfusion. Dissection is another possible complication of IABP placement and may occur in the aorta or anywhere else along the course of femoral or axillary catheter placement. Other complications include bleeding at the site of placement, distal embolic events, and limb ischemia. Balloon rupture can occur but cannot be diagnosed on radiograph.
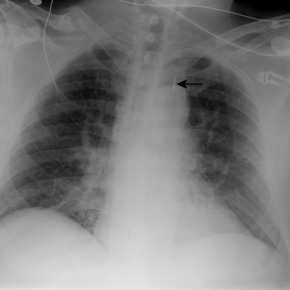
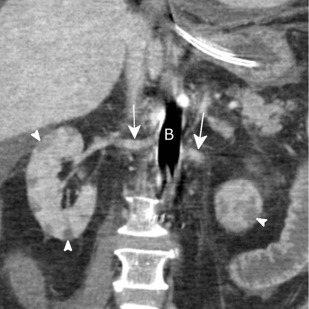
Impella
Configurations and Physiology
The Impella (Abiomed Inc., Danvers, MA) device is a newer percutaneous LVAD used to augment cardiac output and reduce myocardial workload in patients with advanced heart failure and/or cardiogenic shock. It has been shown to provide improved hemodynamic support compared with the IABP and is endorsed in certain settings by both European and American guidelines. Meta-analysis of clinical trials has demonstrated mortality benefit in early invention and thus the Impella is often placed before percutaneous coronary intervention (PCI) in acute MI with cardiogenic shock and in high-risk elective PCI procedures.
Impella variants exist for both left and right ventricular support, including multiple models for left ventricular (LV) support ( Table 1 ). The LV Impella design incorporates a catheter housing an axial pump and outlet area at its proximal end and a distal inlet area connected to a thin pigtail providing stabilization for positioning across the aortic valve into the LV. The rotational pump delivers nonpulsatile flow and, unlike the IABP, the Impella provides stable hemodynamic support regardless of cardiac rhythm. The Impella is approved by the Food and Drug Administration for 6 hours of use but is often used in off-label applications for days to weeks where it can provide support in the acute setting or serve as a bridge to ventricular assist device (VAD) placement or heart transplantation. Contraindications include mechanical aortic valve, severe aortic stenosis, ventricular septal defect, and severe peripheral arterial disease.
Impella 2.5 | Impella CP | Impella 5.0 | Impella LD | Impella RP | |
---|---|---|---|---|---|
Ventricle | Left | Left | Left | Left | Right |
Motor size | 12 F | 14 F | 21 F | 21 F | 22 F |
Insertion | Femoral or axillary artery, percutaneous | Femoral or axillary, percutaneous | Femoral or axillary artery cutdown | Surgical placement into aorta | Femoral vein |
Maximum blood flow | 2.5 L/min | 4.0 L/min | 5.0 L/min | 5.0 L/min | 4.6 L/min |
Normal Appearance
Impella devices have a distinctive appearance and are easy to recognize on chest radiograph and CT ( Fig. 5 ). The metallic pump and inlet/outlet areas are densely radiopaque, and the catheter housing/tip are usually readily apparent. The much more rarely encountered Impella LD lacks the pigtail associated with the other devices and takes a distinct, direct transaortic course. Radiographic evaluation should focus on proper transaortic valve placement with the inlet area and pigtail projecting over the LV contour and the outlet area projecting over the proximal ascending aorta near the aortic root in LV devices. In the right ventricular (RV) Impella device, the inlet area should project over the inferior vena cava and the outlet area over the main pulmonary artery ( Fig. 6 ). Evaluation as for any potential complications is also crucial.
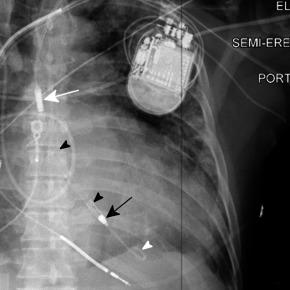
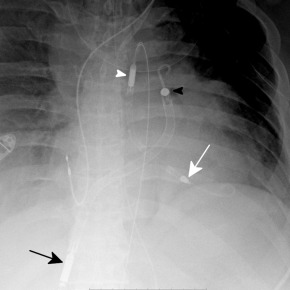
Complications
Reported complications associated with the Impella device include device failure (10%–20%), device migration (20%), hemolysis (5%–10%), and bleeding either during or after implantation (5%–10%). LV Impella malpositioning may occur either too proximal or distal ( Fig. 7 ). Rare complications include aortic valve injury, cardiac tamponade, and acute mitral regurgitation. Acute vascular complications including limb ischemia, hematoma, and/or pseudoaneurysm at the site of insertion occur in approximately 15% to 20% of cases.
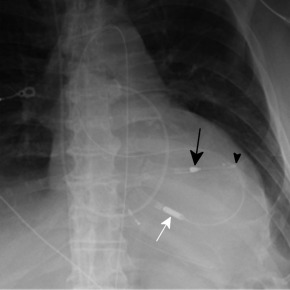
Extracorporeal membrane oxygenation
Configurations and Physiology
Extracorporeal membrane oxygenation (ECMO) provides cardiopulmonary support in cases of potentially reversible acute respiratory and/or cardiac failure. Venous blood is pumped through an extracorporeal oxygenator that saturates hemoglobin with oxygen and removes carbon dioxide before returning blood to circulation.
There are 2 main configurations of ECMO: venovenous-ECMO (VV-ECMO) and venoarterial-ECMO (VA-ECMO). In VV-ECMO, a single-cannulation or double-cannulation approach may be used. Single-cannulation technique uses a dual-lumen cannula (Avalon Elite; Avalon Laboratories, Rancho Dominguez, CA), which drains venous blood through outer lumen fenestrations in the superior vena cava and inferior vena cava (IVC), and then returns oxygenated blood through a second lumen with a port in the right atrium ( Fig. 8 ). Double-cannulation technique involves placement of 2 separate cannulas, one via the common femoral vein for drainage and the other via the internal jugular vein or femoral vein for infusion ( Fig. 9 ). In VA-ECMO, a cannula drains venous blood from the vena cava or right atrium and oxygenated blood returns to arterial circulation through the femoral, axillary, or carotid artery in a so-called peripheral ECMO circuit ( Fig. 10 ), or through the aorta in a central ECMO circuit.
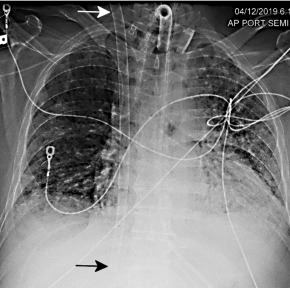
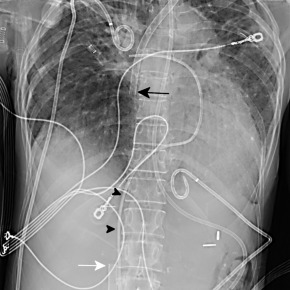
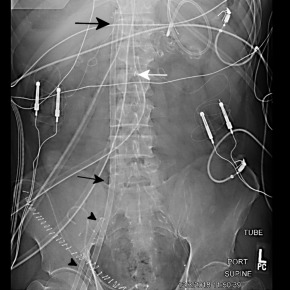
Both VV-ECMO and VA-ECMO provide respiratory support by supplementing oxygenation, but a key distinction is that only VA-ECMO provides hemodynamic support. VA-ECMO bypasses the pulmonary circulation allowing for decreased pulmonary artery pressures and decreased LV preload, thereby decompressing the LV. Direct arterial blood return also increases systemic pressures, improves end-organ perfusion, and maintains a higher Pa o 2 compared with VV-ECMO. Given the different physiology of VV-ECMO versus VA-ECMO, their indications also differ. VA-ECMO is used in the setting of acute cardiogenic shock when low cardiac output and hypotension are refractory to volume resuscitation, vasopressors, inotropic supplementation, or IABP. VA-ECMO also may be used post cardiothoracic surgery in patients unable to be weaned from cardiopulmonary bypass or as a bridge to heart transplantation. However, ECMO is often transitioned to a VAD before heart transplantation as a direct ECMO bridge to transplant is associated with poor 1-year and 5-year posttransplant outcomes.
Either VV-ECMO or VA-ECMO can be used for respiratory support to provide rescue therapy in acute respiratory failure or as a bridge to lung transplantation, although VV-ECMO may be preferred if cardiovascular support is not needed, as it avoids the complications of arterial cannulation. ECMO in the management of acute respiratory distress syndrome (ARDS) provides a significant survival benefit. ECMO allows for “lung rest” and facilitates lung-protective ventilation strategies including decreased tidal volumes and Fi o 2 to decrease ventilator-associated injury and oxygen toxicity.
Imaging
Chest and abdominal radiographs are obtained to confirm and monitor cannula placement. Single-cannulation VV-ECMO cannula is placed via an internal jugular approach with distal tip in the IVC above the hepatic veins, outflow port oriented toward the tricuspid valve (see Fig. 8 ). In the double-cannulation VV-ECMO technique, the inflow cannula is placed in the IVC near the inferior cavoatrial junction from a femoral approach and the outflow cannula is positioned in the right atrium near the superior cavoatrial junction from either a femoral or internal jugular approach (see Fig. 9 ).
In central VA-ECMO, the inflow cannula should be positioned in the right atrium and outflow cannula in the ascending aorta. In peripheral VA-ECMO, the inflow cannula tip is again placed in the IVC or right atrium from a femoral approach (internal jugular approach less common), but the arterial cannula is placed via the femoral artery into the common iliac artery (see Fig. 10 ). A distal perfusion catheter connected to the ECMO arterial outflow may be placed at the site of arterial access. This will appear as a smaller caliber catheter near the arterial ECMO cannula with tip oriented in the opposite direction, retrograde in the femoral or axillary artery.
CT is helpful in the evaluation of patients with ECMO. However, altered hemodynamics in VA-ECMO circulation poses a challenge during contrast-enhanced CT. In full VA-ECMO, there is complete cardiopulmonary bypass with an empty LV and closed aortic valve. Contrast injected peripherally would be drawn into the venous cannula, circulate through the ECMO circuit, then infuse into the arterial cannula. Any contrast opacification of the aorta in this case is a result of retrograde flow from pressure of blood returning from the arterial ECMO cannula, with contrast mixing or layering in the aortic lumen ( Fig. 11 ). Mixing artifact and contrast layering can be misinterpreted as an aortic dissection and/or thrombus. A delayed phase of imaging can often provide more homogeneous vascular opacification.
