Neurologic injury arises from treatment of central nervous system malignancies as result of direct toxic effects or indirect vascular, autoimmune, or infectious effects. Multimodality treatment may potentiate both therapeutic and toxic effects. Symptoms range from mild to severe and permanent. Injuries can be immediate or delayed. Many early complications are nonspecific. Other early and delayed neurologic injuries, such as posterior reversible encephalopathy syndrome, dural sinus thrombosis, infarctions, myelopathy, leukoencephalopathy, and hypophysitis, have unique imaging features. This article reviews treatment options for neurologic malignancies and common and uncommon neurologic injuries that can result from treatment, focusing on radiologic features.
Key points
- •
Neurologic injury arise from treatment of central nervous system malignancies (primary and secondary) as result of direct toxic effects or indirect vascular, autoimmune, or infectious effects.
- •
Neuro-oncologists, radiation oncologists, and radiologists are likely to encounter an increase in number of patients with treatment-induced complications given increased survival rates and increased treatment options, including novel immunotherapies.
- •
Neurocognitive effects can occur in patients who live more than 6 months after radiation therapy and have been best described in children.
- •
Central nervous system complications of chemotherapy include aseptic meningitis, stroke-like syndromes, posterior reversible encephalopathy syndrome, dural sinus thrombosis, transverse myelopathy, and delayed leukoencephalopathy.
- •
Immunotherapy in neurologic tumors currently include check point inhibitors, vaccines, viral therapy and chimeric antigen receptor T-cell therapy.
Introduction
Primary and secondary central nervous system (CNS) malignancies present a treatment challenge owing to overall poor prognosis and presence of blood brain barrier. Glioblastoma (GBM) is the most aggressive primary brain tumor in adults, with a 2-year survival rate of approximately 17% and current median survival of 15 months. Close to 20,000 patients are diagnosed in the United States with GBM each year. Brain metastasis occurs in 8% to 10% of adult patients with cancer. The common primary malignancies that metastasize to the brain are lung, breast, and melanoma. Additional cancers with brain metastases include renal cell, colorectal cancer, and gynecologic cancers. Brain metastases are typically associated with advanced stage cancer. With improvement in the survival of patients with systemic cancers, it is expected that incidence of brain metastasis will only increase. Mainstream treatments for CNS malignancies include resection, radiation, chemotherapy, immunotherapy, and combination treatments.
In general, treatments have improved outcomes in some malignancies, especially lymphoma, leukemia, and melanoma. Survival for malignant glioma has not significantly improved in the past 3 decades despite advances in treatment. Brain metastases are associated with a poor prognosis similar, to that of GBM, with a median survival of about 12 to 15 months. Despite major advances in oncologic diagnosis and treatment, the survival time for patients treated with radiation therapy remains at 3 to 6 months. Overall survival is often determined by the extent and activity of the primary tumor.
Unfortunately, all oncologic therapies are to some extent neurotoxic. Neurologic injury arise from treatment of CNS malignancies (primary and secondary) as result of direct toxic effects or indirect vascular, autoimmune, or infectious effects. Multimodality treatment may potentiate both therapeutic and toxic effects. Symptoms range from mild to severe and permanent and can be greatly debilitating to patients and their caregivers. Injuries can be immediate or delayed. Neuro-oncologists, radiation oncologists, and radiologists are likely to encounter an increase in the number of patients with treatment-induced complications given the increased survival rates and increased treatment options, including novel immunotherapies.
Many of the early complications are nonspecific and either do not require imaging for diagnosis or have no definite radiographic correlate. These complications include headache, nausea, vomiting, dizziness, confusion, dystonia, Parkinsonian symptoms, cerebellar syndrome, tremors, and seizures. Other early and delayed neurologic injuries, such as posterior reversible encephalopathy syndrome (PRES), dural sinus thrombosis, infarctions, myelopathy, leukoencephalopathy, and hypophysitis, have unique imaging features.
This article reviews the current treatment options for CNS malignancies and common and uncommon neurologic injuries that can result from treatment, with a focus on radiologic features.
Radiation therapy
Radiation therapy remains mainstay of treatment for primary and secondary CNS malignancies. Approximately 200,000 patients per year receive radiation yearly in the United States. Currently, photons, electrons, and protons are all used in particle-based radiation therapy. Cranial radiation therapy may be delivered externally or be injected or implanted. Treatment may be targeted or whole brain based, and administered as a single fraction or fractionated. Regardless, all radiation therapy relies on DNA breakage as the mechanism of cell death.
Whole brain radiation therapy is used for brain metastasis. It is administered to the entire brain, usually over multiple treatments. Local field external beam radiation is used for malignant gliomas because the overwhelming majority of recurrences occur at or immediately adjacent to the original tumor. Stereotactic radiosurgery delivers precisely targeted radiation to brain lesions and can be used for both metastases and primary brain tumors. For high-grade gliomas, adjuvant radiation therapy after maximal safe resection improves local control and survival. Involved field radiation therapy delivers radiation to the tumor or tumor bed plus a margin of radiographically normal tissue. The standard dose for GBM is 60 Gy given over 6 weeks in 2-Gy fractions, usually with concurrent chemotherapy. For anaplastic astrocytoma (World Health Organization grade III), usually a slightly lower total dose is used in slightly smaller fractions.
Particle radiation (neutrons, helium ions, and protons) have been studied as boosters to conventional radiation therapy. The theoretic advantage to these particles are finite path lengths and the ability to concentrate the majority of their dose at the end of their path length, with little exit dose. This feature potentially decrease radiation exposure of normal surrounding tissue. Proton radiation is commonly used to treat pediatric CNS tumors, such as medulloblastoma, but its superiority in other CNS malignancies is not established. Similarly, brachytherapy, which is the placement of radioisotope seeds in the tumor or surgical cavity, is of limited usefulness in malignant gliomas. , Malignant gliomas tend to be infiltrative tumors, and brachytherapy delivers high-dose radiation to areas located few millimeters from the seeds; therefore, it does not adequately reach the full extent of disease.
Sheline was the first to classify the radiation-induced side effects according to their time of appearance after irradiation into acute disorders (days to weeks), early–delayed complications (1–6 months), and late–delayed complications (<6 months). The mechanism of radiation therapy-induced damage to the CNS seems to be complex and likely to include a combination of vascular injury, demyelination, neuronal damage, effects on the fibrinolytic enzyme pathway, and immune mediated. The vascular injury is thought to be partly responsible for the abnormal vasculature, thrombosis, and fibrinoid necrosis eventually leading to radiation necrosis. Radiation therapy also causes cell depletion, especially oligodendrocytes, which in turn leads to demyelination and white matter necrosis. Other cells such as neurons, astrocytes, and microglia are also damaged. Other factors that increase the risk of radiation-induced toxicity are older age, concurrent diseases (such as diabetes and hypertension), vascular disease, concurrent chemotherapy, and genetic predisposition. In stereotactic radiosurgery, additional risk factors include dose, treatment volume, location of lesion(s), and concurrent systemic treatment.
In the human body, different cell systems have different sensitivity to the radiation. Cells that are actively reproducing are more sensitive to radiation therapy than those that are not. Blood cells that are constantly regenerating are, therefore, most sensitive, whereas nerve and muscle cells are the slowest to regenerate and therefore are least sensitive to radiation. Although the vascular endothelial cells and oligodendrocytes have been regarded as direct primary targets of radiation, the overall effect is thought to be multifactorial and attributed to more than 1 cell lineage.
Early Complications of Radiation Therapy
Many of the early complications are nonspecific and without radiographic features, including fatigue, headache, local skin reaction, alopecia, nausea and vomiting, dizziness and vertigo, and anorexia. Some patients present with acute encephalopathy and seizures without imaging correlates. Focal neurologic deficits and seizure in some cases can worsen owing to cerebral edema can be seen in some cases, especially when the treated lesion is larger in size and after stereotactic radiosurgery, and can account for symptoms within 2 weeks of treatment. Other complications include serous otitis and parotitis.
Delayed Complications of Radiation Therapy
Pseudoprogression refers to treatment effects that mimic the appearance of tumor progression, often occurring in the first 3 months after treatment. Pseudoprogression is more common in patients with MGMT -methylated GBM. Differentiating psuedoprogression from true progression is a particular challenge in the interpretation of surveillance MR imaging. Radiation necrosis (RN) is the delayed effect of radiation therapy that can occur anywhere from 1 to 10 years after treatment, with the peak period between 1 and 3 years. On MR imaging, RN can have a confusing and bizarre appearance with hyperintensity on T2-weighted (T2W) imaging and fluid attenuation inversion recovery (FLAIR) imaging, edema, and marked enhancement. Knowledge of the radiation therapy history and plan is helpful to differentiate RN from true progression. Advanced MR techniques are also helpful, especially perfusion and spectroscopy, because the cerebral blood volume will be low in RN and spectroscopy will demonstrate elevated lactate without elevation of choline ( Fig. 1 ). Necrosis seems to be dose dependent and tends to occur at or near the site of treatment. RN can occur in more distant sites, such as the inferior frontal lobes and brain stem in patients treated for nasopharyngeal carcinoma. Pseudoprogression and RN are discussed in detail elsewhere in this issue.
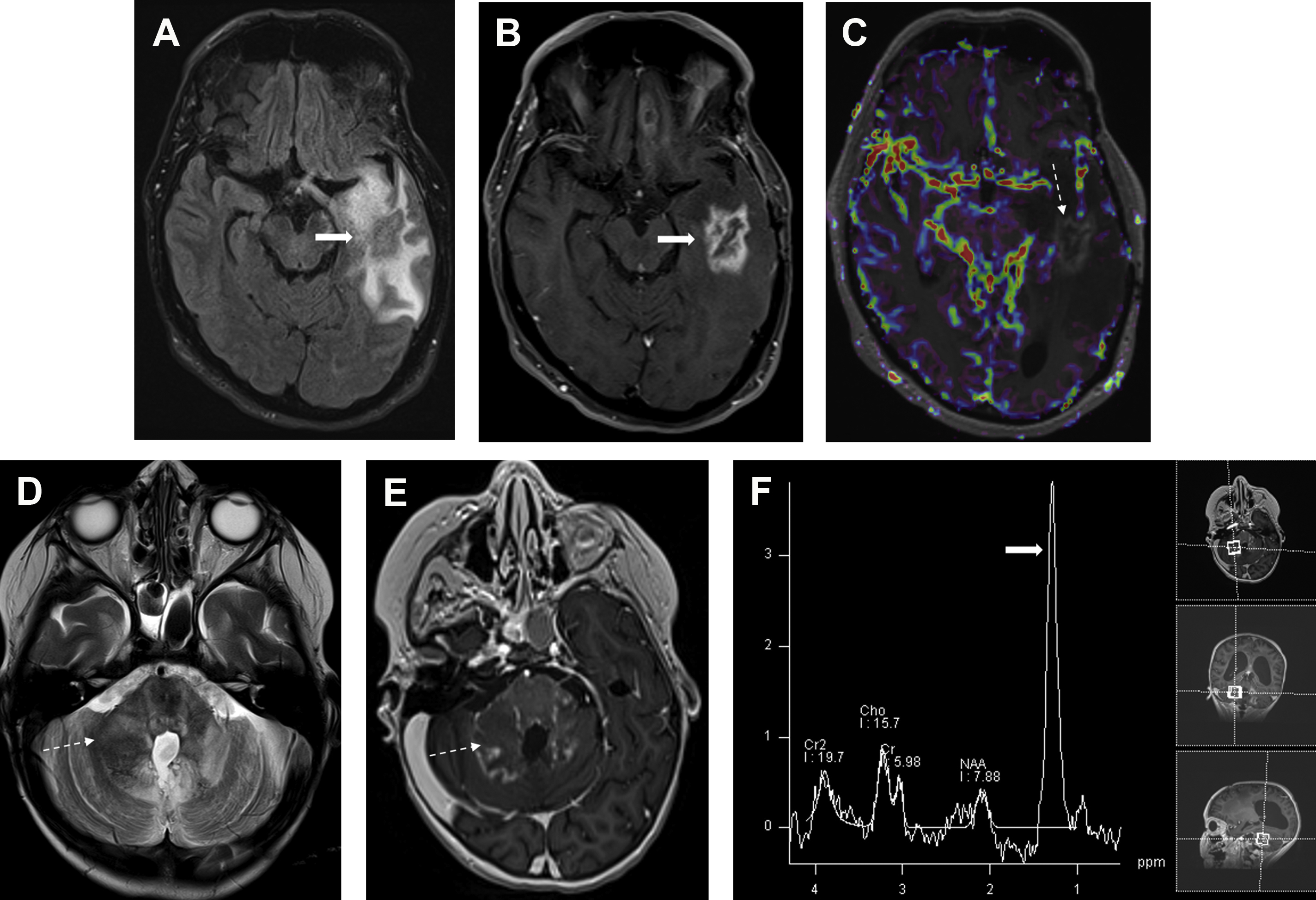
Stroke, cavernous malformations, and hemorrhage can occur as a delayed complications of radiation therapy, especially in patients who received radiation therapy at a younger age or received high doses, with irradiation of the circle of Willis region. Stroke has been noted in both pediatric and adult patients and seems to be associated with dose to the circle of Willis. Moya moya–like disease has been reported approximately 3 to 4 years after radiation therapy and is found in higher rates in children and those with neurofibromatosis type 1. Cavernoma develops approximately 3 to 6 years after radiation therapy and may grow over time; occasionally, they can hemorrhage and cause edema. The typical MR imaging appearance of cavernoma is of a relatively well-defined lesion with heterogeneous T1-weighted (T1W) signal owing to subacute blood products and a T2W hypointense rim. There is exuberant blooming on susceptibility weighted imaging sequences ( Fig. 2 ). Usually, there is little or no surrounding edema, unless there is a recent hemorrhage.
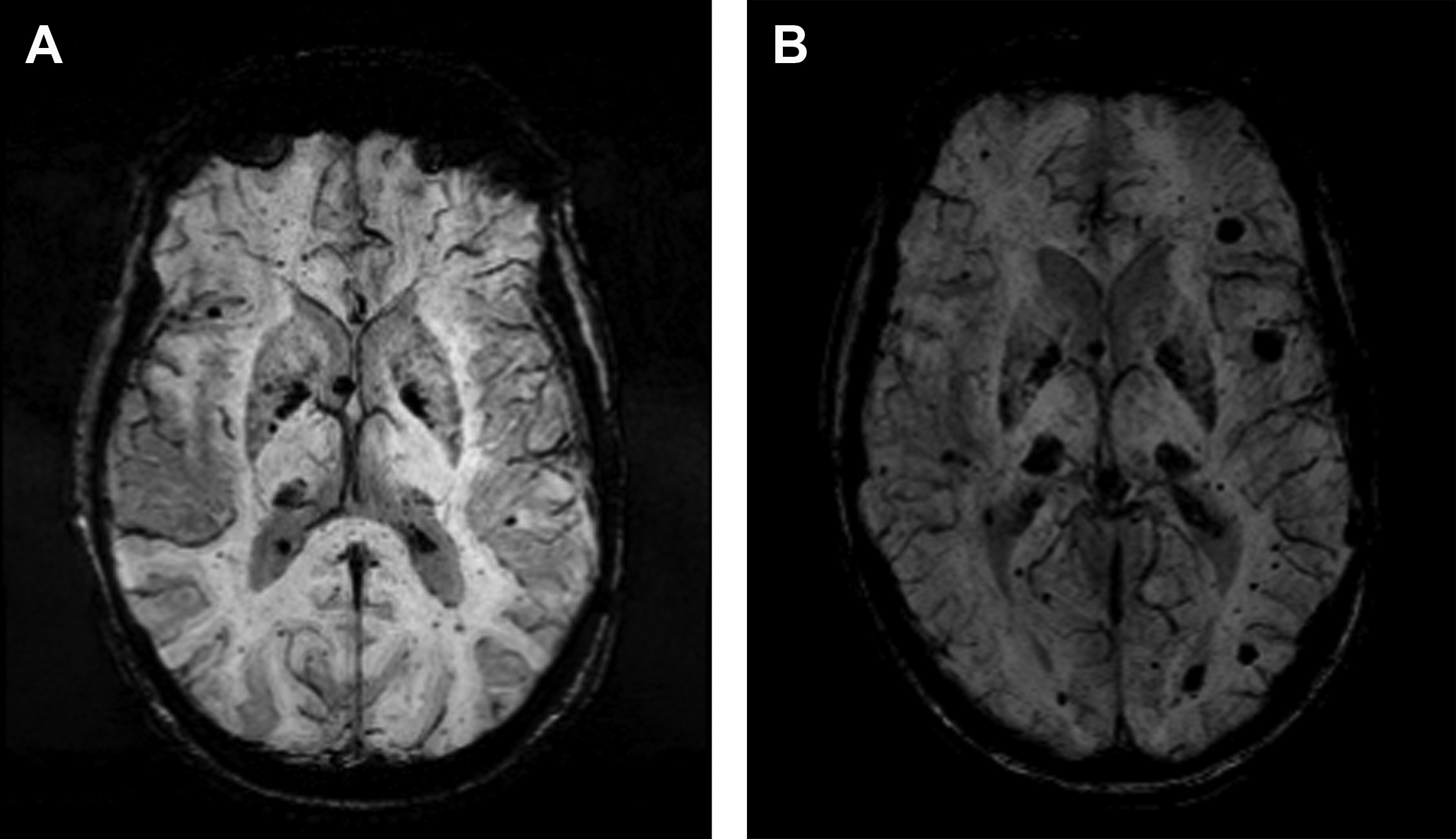
Stroke-like migraine attacks after radiation therapy syndrome is a delayed complication characterized by complex neurologic signs and symptoms in patients with history of chemoradiotherapy, usually whole brain radiation. It has been reported in patients ranging from 6 to 30 years after radiation treatment. Patients present with subacute onset of stroke-like symptoms, such as homonymous hemianopsia, hemiplegia, aphasia, and/or seizures. MR imaging reveals cortical edema and gadolinium enhancement, often leptomeningeal, not following any particular vascular distribution ( Fig. 3 ). Findings are potentially confusing for tumor progression or recurrence. It is still a poorly understood entity. The majority of patients have resolution of symptoms over the course of several weeks, with resolution of radiographic abnormalities, but up to 45% can have residual deficits. Stroke-like migraine attacks after radiation therapy syndrome can also recur.
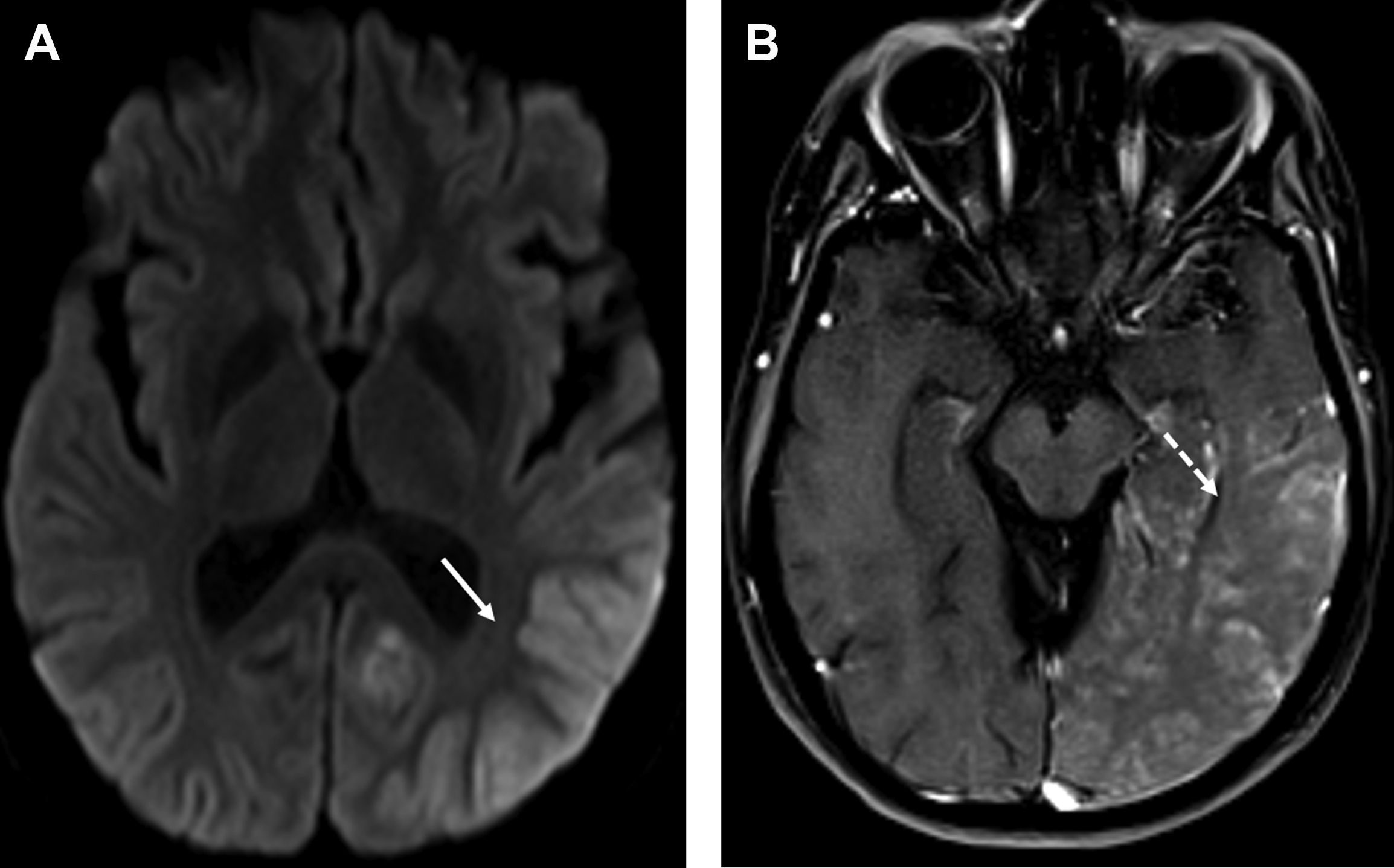
Pituitary and hypothalamic dysfunction are common after radiation therapy and may occur in up to 80% of patients, with grown hormone deficiency being the most commonly encountered complication in children. Young and pediatric patients with sellar and/or suprasellar disease may experience hypothalamic hyperphagia and obesity after treatment.
Delayed radiation effects on the spinal cord can present as a myelopathy syndrome or a lower motor neuron syndrome. Imaging shows T2W hyperintensity of the spinal cord with or without enhancement and follow-up imaging shows volume loss indicating myelomalacia. Rarely, patients can present with cord hemorrhage, thought to be from an underlying radiation-induced vascular malformation ( Fig. 4 ).
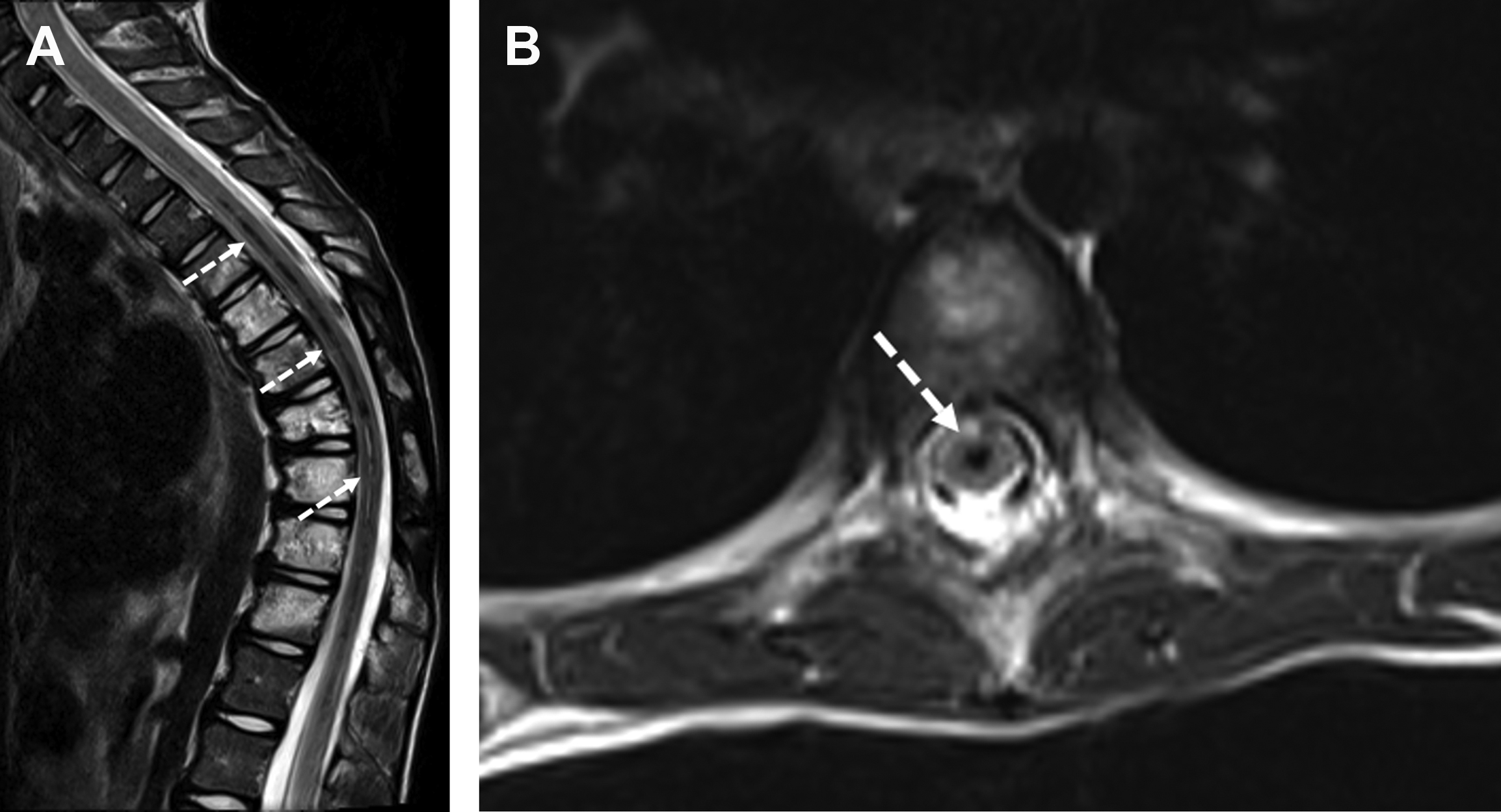
Radiation-induced malignancy is a dreaded late complication of wide field radiation. Malignancies that can develop include meningioma, glioma, sarcoma, and nerve sheath tumor ( Fig. 5 ). These may occur anywhere from 5 years to decades after treatment and are a serious concern for patients with long expected survival after primary malignancy. This entity is less of a concern in patients with metastatic CNS disease or GBM, whose expected survival is often (much) shorter than 5 years. It is possible that narrow field radiation such as stereotactic radiosurgery may be associated with a lower incidence of secondary malignancy. Outcomes comparing photon versus proton based therapies are lacking.
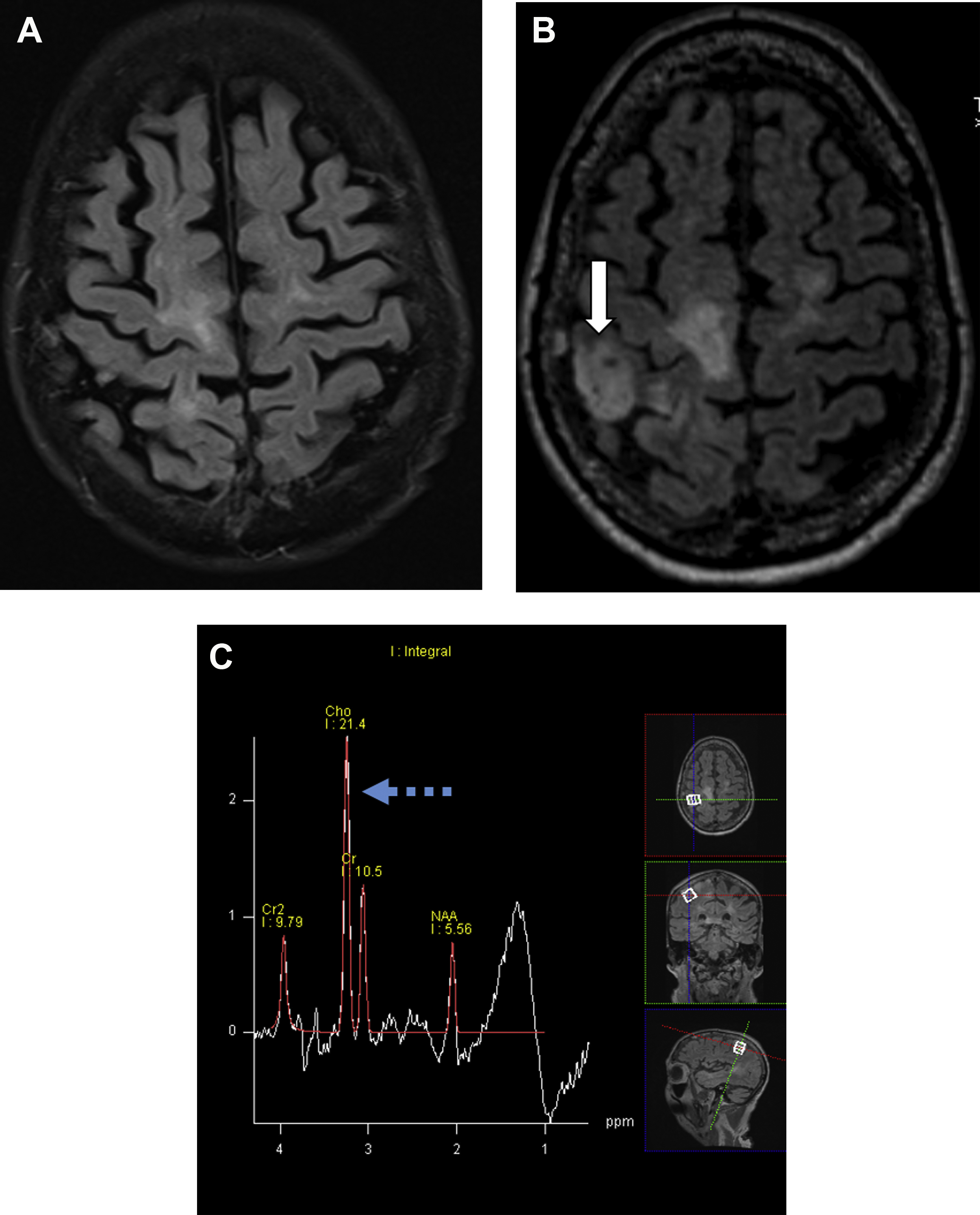
Neurocognitive effects can occur in patients who live more than 6 months after radiation therapy and have been best described in children who survived brain tumors or acute lymphocytic leukemia. , Children, especially those who were treated with high-dose chemoradiotherapy before age 6, showed lower scholastic achievement and lower IQ. This effect seems to be dose dependent based on research with children with high- versus low-risk medulloblastoma. In adults, whole brain radiation therapy has also been linked to decline in memory and learning functions in patients with non-small cell lung cancer. Radiographic findings include nonspecific white matter FLAIR hyperintensity and brain atrophy ( Fig. 6 ). The relationship between partial brain radiation and neurocognitive decline is less well-established, and targeted radiotherapies may decrease the effect on cognition.
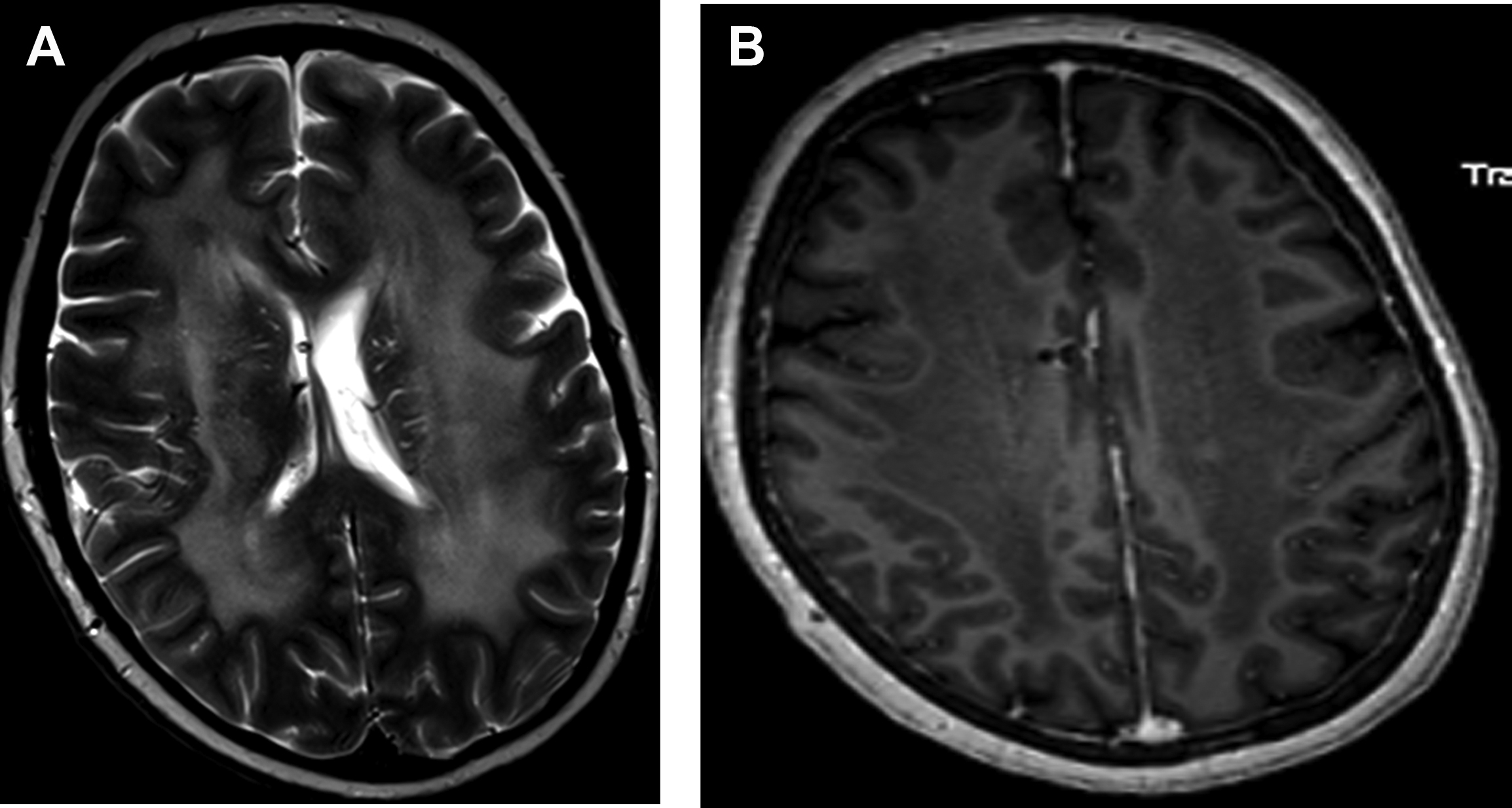
Chemotherapy
Chemotherapy, both systemic and intrathecal, and often in combination, remains some of the most effective and widely used treatments for cancer. There are many classes of chemotherapy agents based on their mechanism of action, and many are known to cause neurologic complications. Complications can be acute (during treatment or within 50 days from end of therapy), subacute or delayed (within 3 months of therapy), and late (beyond 3 months). Peripheral neuropathy is most common chemotherapy associated neurotoxicity, especially with platinum based agents. Taxanes and vinca alkaloid agents are also associated with sensory neuropathy, and vincristine has been associated with autonomic and cranial neuropathies.
CNS complications include aseptic meningitis, stroke-like syndromes, PRES, dural sinus thrombosis, transverse myelopathy, and delayed leukoencephalopathy ( Fig. 7 ). Methotrexate (MTX) CNS toxicity is best described, but other antimetabolites can cause optic neuropathy, cerebellar syndrome, acute encephalopathy, and cerebral venous thrombosis and venous infarction or hemorrhage in the brain.
