321CHAPTER 20
Immunoradiosurgery: The Convergence of SBRT and the Immune Response
Stereotactic body radiation therapy (SBRT) provides an opportunity for harnessing technological advancements (i.e., physical and geometric) to improve upon tumor control by delivering ablative doses of highly conformal ionizing radiation in a reproducible manner to a limited target volume. It has proven effective in the treatment of patients with a wide spectrum of malignancies and is now integrated into all phases of the oncologic treatment paradigm including definitive therapy and palliation, as well as into the curative treatment of patients with a limited burden of metastatic or oligometastatic disease (1–3). It is in this setting that the least volume of data exists but the greatest opportunity for not only the expansion of indications for SBRT but also an increased understanding of the radiobiology of SBRT. Therefore, opportunity exists to exploit and integrate SBRY in combination with novel systemic therapies to offer potentially curative therapy and an expanded approach with the integration of immune therapies, especially the introduction of modulators of the checkpoint signaling cascade (4, 5).
Immunotherapy, although used for over 30 years in diseases such as malignant melanoma, has renewed clinical interest with introduction and subsequent Food and Drug Administration (FDA) approval of novel checkpoint inhibitors into the management of a variety of malignancies including non–small-cell lung cancer (NSCLC) (6). Despite the optimism surrounding immune-checkpoint blockade, the vast majority of patients will eventually progress secondary to immune exhaustion and succumb to their disease (7–10). The mechanism of immune escape and strategies to circumvent this process are not well understood at the moment and are being explored but one option includes the use of radiation therapy (RT). Ionizing radiation and its effects on DNA damage are a proposed mechanism in which the immune response is manipulated by increasing tumor antigen release, activating innate immunity, increasing T-cell infiltration, and modifying the tumor microenvironment (11, 12). The manipulation of the immune response with ionizing radiation, especially as a minimally-invasive technique, along with the use of novel imaging agents is of great interest when combining SBRT and immunotherapies as treatments could be modified and optimized based on immune response.
CLINICAL RATIONALE
The immune system is made up of both innate and adaptive immune cells. Both branches play an integral role in forming and shaping the tumor 322microenvironment. When a cancer cell expresses or releases antigens, they are commonly released into the lymphatics. These tumor-associated antigens (TAAs) are then taken up by antigen-presenting cells (APCs) such as dendritic cells (DCs) (Figure 20.1). The antigens are processed by antigen-processing machinery and placed on major histocompatibility complex I and complex II (MHC-I and MHC-II) molecules (13). These peptide–MHC complexes are then available for antigen presentation: MHC-I to CD8+ T lymphocytes and MHC-II to CD4+ T lymphocytes. Secondary signaling through costimulatory molecule interactions such as CD80/86 with CD28 as well as tertiary cytokine signals is used for optimal effector cell activity. Once activated, CD8+ T lymphocytes become cytotoxic T lymphocytes (CTLs) and hone to the tumor to release cytolytic molecules such as granzyme B and perforin for cell kill. CTL activity is enhanced by CD4+ T-helper lymphocyte differentiation to T-helper 1 (Th1) phenotype-releasing cytokines such as interferon-γ (IFN-γ) to create a proimmunogenic environment. Throughout this activation and effector process, immune memory toward the antigen is formed. Normally the immune system is able to survey the body and aid as an extrinsic mechanism to eliminate cancer cells. This is otherwise known as “immunosurveillance.” The three hallmarks or stages of immunosurveillance are known as the 3Es: Elimination, Equilibrium, and Escape (Figure 20.2) (14, 15). The mechanism of cell-killing mentioned earlier is the elimination phase. Within the equilibrium phase, there is homeostasis of immune cells recognizing and keeping cancer cells at bay, whereas the current cancer cells undergo intrinsic changes to prevent detection and outright elimination. However, once a cancer develops subsequent mutation or the microenvironment becomes one of immune suppression, cancer cells can “escape” immune control and continue to grow and become clinically apparent. These intrinsic changes are the target of modern immune therapies and SBRT can be used as a therapeutic strategy to optimize treatment response.
FIGURE 20.1 SBRT targets the primary tumor and ultimately causes cell death. Tumor antigens are released into circulation and taken up by dendritic cells. Dendritic cells traffic back to lymph nodes and activate antigen specific T cells. Immunotherapeutic agents can also be used to enhance immune responses in conjunction with SBRT.
SBRT, stereotactic body radiation therapy.
323
FIGURE 20.2 The cancer immunoediting concept. The 3 E’s of immune surveillance include Elimination, Equilibrium, and Escape. The immune system can control tumor growth with various immune mediators. However, tumor suppressor cells and immunosuppressive cytokines can permit tumor escape.
Source: From Schreiber RD, Old LJ, Smyth MJ. Cancer immunoediting: integrating immunity’s roles in cancer suppression and promotion. Science. 2011;331(6024):1565–1570. doi: 10.1126/science.1203486
RT has a mechanism of action that extends well beyond that of DNA damage. Preclinical data demonstrate that ionizing radiation results in effects beyond that of DNA damage including the release of tumor antigen and increase in the cell surface expression of antigen and receptors that influence T-cell response and subsequent T-cell trafficking and as antitumor activity (16–19). Successful integration of radiation and immunotherapies may serve to optimize the influx of T effector cells, while overcoming extrinsic immunosuppressive cells such as regulatory T cells (Tregs), myeloid-derived suppressor cells (MDSCs), and tumor-associated macrophages (TAMs) (20–22).
Selective dose and fractionation optimization with SBRT is of great interest in both preclinical and ongoing clinical trials. Data from murine models suggest that fractionated regimens (8 Gy × 3, 6 Gy × 5) in combination with anti-CTLA-4 antibody are more effective in maximization of tumor control as well as off-target, abscopal effects than single radiation doses (20 Gy × 1) (23). This is in contrast to manipulation of programmed death-1/programmed death-ligand 1 (PD-1/PD-L1) pathway blockade where single fraction data have both antitumor and abscopal effects (Figure 20.3) (11). Current clinical trials are exploring optimal combination of checkpoint blockade with radiation dose, fractionations, as well as the volume of tumor targeted.
CLINICAL EXPERIENCE
SBRT has revolutionized RT. Its use and indications have grown dramatically since its inception. This includes primary treatment of inoperable early stage NSCLC, metastatic disease, as well as reirradiation such as in recurrent head and neck cancer. Interestingly, in preclinical models, high dose per fraction of radiation such as ones with SBRT or hypofractionation in general is increasingly immunogenic when compared with a lower dose per fraction regimens. In addition, less of the circulating lymphocyte pool in blood is exposed to radiation when compared with standard fractionation. For these reasons, the role of SBRT with immunotherapies is being explored. For example, Seung et al. in 2012 reported a phase I clinical trial looking at patients with metastatic melanoma or renal cell carcinoma and treated with Interleukin-2 (IL-2) and SBRT 20 Gy in one to three fractions (24). Notably, they found a 67% partial or complete response rate (N = 12). In addition, there was a significant increase in the number of proliferating activated CD4+ T cells in treatment-responsive patients. The excellent response rate in this trial was surprising given historical response rates using conventional radiation treatment and IL-2 were lower. Overall the trial was suggestive that SBRT improved immunogenicity and therefore improved response rates.
324
FIGURE 20.3 SBRT can cause immunogenic cell death and T-cell priming. However, over time, T-cells can take on an exhausted phenotype. With PD-1 and PD-L1 inhibitors, exhausted T cells can regain effector function.
PD-1, programmed death-1; PD-L1, programmed death-ligand 1.
Source: From Walshaw RC, Honeychurch J, Illidge TM. Stereotactic ablative radiotherapy and immunotherapy combinations: turning the future into systemic therapy? Br J Radiol. 2016;89(1066):20160472. doi: 10.1259/bjr.20160472
One of the other first experiences of SBRT with immunotherapy can be seen in recurrent head and neck cancer patients. Here, patients previously treated with RT were enrolled and treated with 44 Gy in five fractions with concurrent cetuximab (25). Cetuximab is an anti-epidermal growth factor receptor (EGFR) monoclonal antibody shown to improve local regional control in head and neck cancers. It not only is a molecular therapy used in blocking ligand-mediated EGFR signaling but is also in fact an immunotherapeutic agent that kills tumors through natural killer (NK) cell antibody-mediated cellular cytotoxicity (ADCC). NK cells recognize the FcγIIIa of the antibody and thus can lead to ADCC (26–28). Furthermore, cetuximab promotes DC cross-priming and CTL responses (29, 30). At the time of initial dose escalation, cetuximab was not included in the phase I trial at the University of Pittsburgh Medical Center (UPMC) Hillman Cancer Center (31). This explains the lower than initially anticipated median survival and response rate seen in the phase I trial. Around the same time period, Bonner et al., in their study of head and neck cancer with cetuximab and concurrent conventional external beam RT, demonstrated improved locoregional control (32). This prompted a retrospective cohort study of SBRT with cetuximab; that study suggested a 10-month improvement in overall survival (OS) without increase in toxicity (33). Given the potential benefit of cetuximab, subsequent clinical trials have included this therapy. On the basis of phase II data from the UPMC, 1-year local progression-free survival (PFS) improved to 60% and locoregional control was 37%. Median survival was 10 months and 1-year OS was 40%. This represented a substantial improvement over SBRT alone. The grade 3 toxicity rate was 6% for both acute and chronic side effects. These results compared favorably with chemotherapy and reirradiation data in which 2-year OS was approximately 20%, G3+ acute toxicity of approximately 70%, and late G3 toxicity of about 30% (34, 35). Clearly cetuximab helped to mediate the benefit seen in the phase II trial without reporting the same high toxicity seen in the chemotherapy 325and reirradiation setting. Currently, most trials using cetuximab with other immunotherapies such as ipilimumab exist in the standard fraction testing. The UPMC does have a trial open investigating outcomes of cetuximab and SBRT with and without docetaxel in recurrent head and neck cancer setting.
Checkpoint inhibitors have become the main focus of recent studies. PD-1 and PD-L1 inhibitors are some of the most well known and studied. The initial use of checkpoint inhibitors used anti-CTLA-4 antibodies in the setting of malignant melanoma. The first phase III trial demonstrated a 4-month survival benefit when ipilimumab was added to gp100 peptide vaccine versus peptide vaccine alone (36). Since establishing the benefit of ipilimumab, numerous case reports and studies have demonstrated when combined with RT substantial partial and distant response to distant tumors away from the primary radiated area (3). The aforementioned abscopal effect gives credence to the important role RT plays in inducing an enhanced and robust immune response. Recurrent head and neck cancers have been shown to demonstrate a benefit in platinum-refractory recurrence setting. Specifically, the Checkmate 141 trial showed that when patients were treated with nivolumab versus standard-of-care methotrexate, docetaxel, or cetuximab, there was an improvement in median survival from 5 to 8 months, improvement in 1-year OS from 17% to 26%, and improved response rate from 6% to 13% without an increase in G3 to 4 toxicity (37). Ninety-one percent of patients had received RT earlier. With the success from Checkmate 141, the next question to ask was whether checkpoint inhibitors when added to SBRT treatment would improve outcomes. The KEYSTROKE trial (Radiation Therapy Oncology Group [RTOG] foundation 3507) is randomizing head and neck cancer patients with unresectable, recurrent, or second primary to SBRT plus or minus pembrolizumab. Pembrolizumab has earlier been shown to have overall response rate of 18%, 6-month PFS of 23%, and a 6-month OS of 59% (38). Other current ongoing studies looking at the use of SBRT and immune checkpoint inhibitors include NCT03446911 that is enrolling patients with early stage NSCLC treated with SBRT with and without pembrolizumab (39). The Belgium MOESM1 trial (NCT02826564) is seeking to evaluate the role of SBRT and pembrolizumab in the metastatic urothelial carcinoma setting (40). Current standard of care with patients with metastatic urothelial cancer is the use of cisplatin-based chemotherapy. Unfortunately, many cannot tolerate cisplatin and are subjected to less potent carboplatin in this setting. Their trial is designed to deliver pembrolizumab in four cycles 200 mg every 3 weeks. Phase I will randomize patients to receive hypofractionated RT to the largest lesion with the dose of 24 Gy in three fractions to either weeks 1 or 3. Primary end points will be dose-limiting toxicity, response rates, and immune responses on the basis of flow cytometry analysis. Another trial using an anti-PD-1 inhibitor (REGN2810) under NCT02383212 will investigate responses when including SBRT (41). Preliminary phase I findings from the trial looked at cyclophosphamide with PD-1 inhibitor of varying doses versus cyclophosphamide, PD-1 inhibitor, and hypofractionation with 27 Gy in three fractions or 30 Gy in five fractions. Initial results demonstrated an improvement in partial response from 10% with monotherapy to 41% with combined therapy. Johns Hopkins has two ongoing trials each with either pembrolizumab or nivolumab in borderline-resectable pancreatic cancer patients with 33 Gy in five fractions and the addition of cellular-based vaccines (42, 43). Additional work on a rarer but extremely aggressive metastatic anaplastic thyroid cancer is being studied at the Memorial Sloan Kettering Cancer Center (MSKCC) using combination of durvalumab (anti-PD-L1) and (anti-CTLA-4) with 9 Gy × 3 of SBRT (44).
Investigational work with other potentiators of the immune system includes LAG3, OX40, 4-1BB. An ongoing trial of patients with prostate metastatic prostate cancer to bone treated with OX40 agonist and 8 Gy × 1 resulted in the increased proliferative and functional activity of NK cell, and CD4+ and CD8+ T cells (45). Another trial with metastatic breast cancer to liver and lung with OX40 agonist and one to two fractions of SBRT (10–25 Gy) from Providence Health and Services (NCT01862900) is accruing (46). On the basis of preclinical work, single agent anti-LAG3 and anti-4-1BB are being tested alone in current trials. The next progression of trial investigation for these two agents is likely to include the use of hypofractionation or SBRT.
The role of SBRT and other immunomodulatory factors such as cytokines, cancer vaccines, and DC-based vaccines is an active area of research. For example, the SBRT-ATAC trial (NCT02581787) out of Stanford University is seeking to establish the maximal tolerate dose and rate of radiation-induced pulmonary fibrosis in early stage NSCLC with fresolimumab, an anti-TGF-β antibody (47). It is believed that lowering the “levels” of TGF-β will lead to a less 326immunosuppressive environment and improve efficacy of SBRT by changing the suppressive tumor microenvironment. Galunisertib is a TGF-β-RI kinase inhibitor that downregulates the expression of SMAD2 and that ultimately leads to decreased levels of the cytokine, making the microenvironment less immunosuppressive (48). This drug with SBRT is subject to a study at the University of Pennsylvania with hepatocellular carcinoma delivering 18 Gy × 1 fraction between cycle 1, day 15 and cycle 1, day 28 with or without galunisertib (NCT02906397) (49). Ongoing studies with IL-2 and SBRT continue to accrue patients with metastatic melanoma or metastatic renal cell carcinoma. On the basis of Portland Providence Medical Center, SBRT (20 Gy in two fractions) was combined with IL-2 (50). A similar trial is being run at the University of Texas Southwestern (UTSW) Medical Center using 8 to 20 Gy in one to three fractions with 14 doses of IL-2 administered in a similar fashion as the trial mentioned earlier (51). It is presumed that creating an immunogenic microenvironment will allow for increased immune responses. DCs, as mentioned earlier, are important to the immune response. The research team at Albert Einstein and Celldex Therapeutics have initiated a trial (NCT02839265) for patients with advanced NSCLC who have progressed through prior treatment and treated them with FLT-3 ligand (CDX-301) to stimulate DCs and treating patients with 34 Gy × 1, 10 Gy × 5, or 18 Gy × 3. Primary end point is 4-month PFS (52). The C4-MOSART trial at the University of Chicago Medical Center in conjunction with Bristol Myers Squibb is targeting immunosuppressive cells such as TAMs with their approach with immunotherapy and SBRT (53). Here they combine nivolumab with either cabiralizumab, which inhibits CSF-1R and blocks TAMs, or nivolumab with urelumab, which binds to costimulatory molecule 4-1BB, against individuals with metastatic disease of any histology. SBRT that is to be delivered in the trial is between 30 and 50 Gy. The optimal timing of SBRT when using in conjunction with immunotherapies is also undergoing investigation. The University of Chicago has the COSINR trial recruiting individuals with metastatic lung cancer with either concurrent or sequential nivolumab and ipilimumab after three to five fractions of SBRT (54).
As we enter an era of new combinatorial treatment with SBRT and immunotherapies, an important new question is raised: How are we to measure outcomes and response to treatment? SBRT requires precision and accuracy to deliver such high doses of radiation. Treatment immobilization is critical to that success. Furthermore, daily cone-beam CT (CBCT) is routinely used to ensure pretreatment planning scan and online imaging. Other than avoiding geographical target miss, this does not provide further information about current treatment-related effects on the tumor. Currently, there is a wave of new technology aimed at tracking tumor responses in real time rather than with static imaging used in image-guided radiation therapy (IGRT). Two particular studies have examined monitoring dynamic changes within tumors during treatment. Our group has reported on the use of immune-PET/CT in murine models of melanoma (B16F10) and p16+ head and neck cancer (MEER) with 89Zr-labeled PD-L1 antibody to visualize radiation-induced changes in the tumor microenvironment in vivo with respect to PD-L1 (55). In our study, we demonstrated differential expression of radiation-induced PD-L1 on the different tumor cell lines with a single fraction of radiation (2 or 10 Gy). B16F10 tended to have higher levels of PD-L1 at earlier time points. In another experiment comparing nonirradiated (non-IR) with irradiated (IR, 2 Gy × 4 fractions) tumors, there was a statistically significant difference in SUVmean demonstrating higher PDL1 expression in the irradiated tumor than in the non-IR tumor. Replicating the same experiment but this time with the MEER cell line using 2 Gy × 10 fractions ± anti-PD-1 inhibitor, there was increased SUVmean from 0.56 to 0.94 comparing non-IR with IR-treated tumors. These findings were confirmed with ex vivo flow cytometry staining and analysis. In addition, CD45+ tumor-infiltrating leukocytes also were shown to express higher levels of PD-L1 compared with the CD45-negative population. Overall, our preclinical model represented one of the first reporting of dynamic change in PD-L1 expression postradiation treatment using PET imaging. A study from Vrije University in Amsterdam in conjunction with Bristol Myers Squibb aimed to characterize PD-1 and PD-L1 expression in the clinical setting in patients with NSCLC treated with nivolumab using PET imaging (56) or labeling; this group used 89Zirconium-labeled nivolumab (89Zr-nivo) and 18F-labeled BMS-986192 (18F-PD-L1). Response was measured in SUVpeak after 6 weeks of treatment with nivolumab. Seven patients with 11 total lesions were evaluated. Each radiolabeled compound was safe and well tolerated, and could 327label lesions of varying PD-1 and PD-L1 expressions. Most importantly, this study showed that in five patients who were appropriate for response evaluation, one patient who had a partial response to treatment also had the highest 18F-PD-L1 SUV uptake value of 14.4. Although this represents an exceedingly small sample size, it is suggestive that safe in vivo tracking of tumor response to immunotherapy is possible and might predict for responders to immunotherapy. Taking these two studies and others into account, we are moving toward a major paradigm shift. Indeed, instead of solely relying on static IGRT, we are approaching an era of dynamic biology-guided radiation treatment (BGRT). BGRT represents three core functions: anatomic guidance, biology guidance, and biological adaptation. With this emerging technique, CBCTs can be used for alignment and live treatment planning. PET tracer–labeled immune markers can hone directly to the tumors that express these markers and act as a biological fiducial sparing normal uninvolved tissue surrounding it. Lastly, using BGRT, immunotherapy can be administered and used in patients who are more likely to respond to treatment. Those whose tumors do not express PD-L1 could be routed toward another treatment modality. Moreover, optimal radiation dosing and timing can be individually tailored to individual patients.
CONCLUSIONS
With the proliferation of combined SBRT and immunotherapy of various approaches, we anxiously await outcomes of these exciting new therapeutic trials and treatment paradigms as we usher in a new era of cancer treatment. Some have suggested that the classic four Rs of radiobiology—reoxygenation, repopulation, repair, and reassortment—actually can have a fifth R for immune-mediated rejection (48). This line of thinking is very much in line with the theory of immunosurveillance and one of the 3Es core concepts of elimination, thus further reinforcing the integral and additive role of immunotherapy and RT.
Radiosurgery and stereotactic body radiation therapy is on the precipice of major change. The potential benefits of fewer fractions, fewer toxicities and the possible enhancement of response rates by exploiting the immunomodulatory effects is tremendous. The need for novel clinical trials to test these interactions and formulate the optimal combination is essential as we look toward a future where the burden of cancer is reduced if not eliminated.
REFERENCES
1. Flickinger JC, Kondziolka D, Lunsford LD, et al. A multi-institutional experience with stereotactic radiosurgery for solitary brain metastasis. Int J Radiat Oncol Biol Phys. 1994;28(4):797-802. PubMed PMID: 8138431.
2. Salama JK, Chmura SJ, Mehta N, et al. An initial report of a radiation dose-escalation trial in patients with one to five sites of metastatic disease. Clin Cancer Res. 2008;14(16): 5255-5259. doi: 10.1158/1078-0432.CCR-08-0358
3. Milano MT, Katz AW, Muhs AG, et al. A prospective pilot study of curative intent stereotactic body radiation therapy in patients with 5 or fewer oligometastatic lesions. Cancer. 2008;112(3):650-658. doi: 10.1002/cncr.23209
4. Balar AV, Weber JS. PD-1 and PD-L1 antibodies in cancer: current status and future directions. Cancer Immunol Immunother. 2017;66(5):551-564. doi: 10.1007/s00262-017-1954-6
5. Ahmadzadeh M, Johnson LA, Heemskerk B, et al. Tumor antigen–specific CD8 T cells infiltrating the tumor express high levels of PD-1 and are functionally impaired. Blood. 2009;114(8):1537-1544. doi: 10.1182/blood-2008-12-195792
6. Topalian SL, Hodi FS, Brahmer JR, et al. Safety, activity, and immune correlates of anti–PD-1 antibody in cancer. N Engl J Med. 2012;366(26):2443-2454. doi: 10.1056/NEJMoa1200690
7. Kansy BA, Concha-Benavente F, Srivastava RM, et al. PD-1 status in CD8+ T cells associates with survival and anti-PD-1 therapeutic outcomes in head and neck cancer. Cancer Res. 2017;77(22):6353-6364. doi: 10.1158/0008-5472.CAN-16-3167
8. Concha-Benavente F, Srivastava RM, Trivedi S, et al. Identification of the cell-intrinsic and-extrinsic pathways downstream of EGFR and IFNγ that induce PD-L1 expression in head and neck cancer. Cancer Res. 2016;76(5):1031-1043. doi: 10.1158/0008-5472.CAN-15-2001
9. Blackburn SD, Shin H, Freeman GJ, et al. Selective expansion of a subset of exhausted CD8 T cells by aPD-L1 blockade. Proc Natl Acad Sci U S A. 2008;105(39):15016-15021. doi: 10.1073/pnas.0801497105
10. Gros A, Robbins PF, Yao X, et al. PD-1 identifies the patient-specific CD8+ tumor-reactive repertoire infiltrating human tumors. J Clin Invest. 2014;124(5):2246-2259. doi: 10.1172/JCI73639
11. Deng L, Liang H, Burnette B, et al. Irradiation and anti–PD-L1 treatment synergistically promote antitumor immunity in mice. J Clin Invest. 2014;124(2):687-695. doi: 10.1172/JCI67313
12. Demaria S, Formenti SC. Radiation as an immunological adjuvant: current evidence on dose and fractionation. Front Oncol. 2012;2:153. doi: 10.3389/fonc.2012.00153
13. Joffre OP, Segura E, Savina A, et al. Cross-presentation by dendritic cells. Nat Rev Immunol. 2012;12(8):557. doi: 10.1038/nri3254
14. Dunn GP, Old LJ, Schreiber RD. The immunobiology of cancer immunosurveillance and immunoediting. Immunity. 2004;21(2):137-148. doi: 10.1016/j.immuni.2004.07.017
15. Dunn GP, Bruce AT, Ikeda H, et al. Cancer immunoediting: from immunosurveillance to tumor escape. Nat Immunol. 2002;3(11):991. doi: 10.1038/ni1102-991
16. Ferrara TA, Hodge JW, Gulley JL. Combining radiation and immunotherapy for synergistic antitumor therapy. Curr Opin Mol Ther. 2009;11(1):37. PubMed PMID: 19169958.
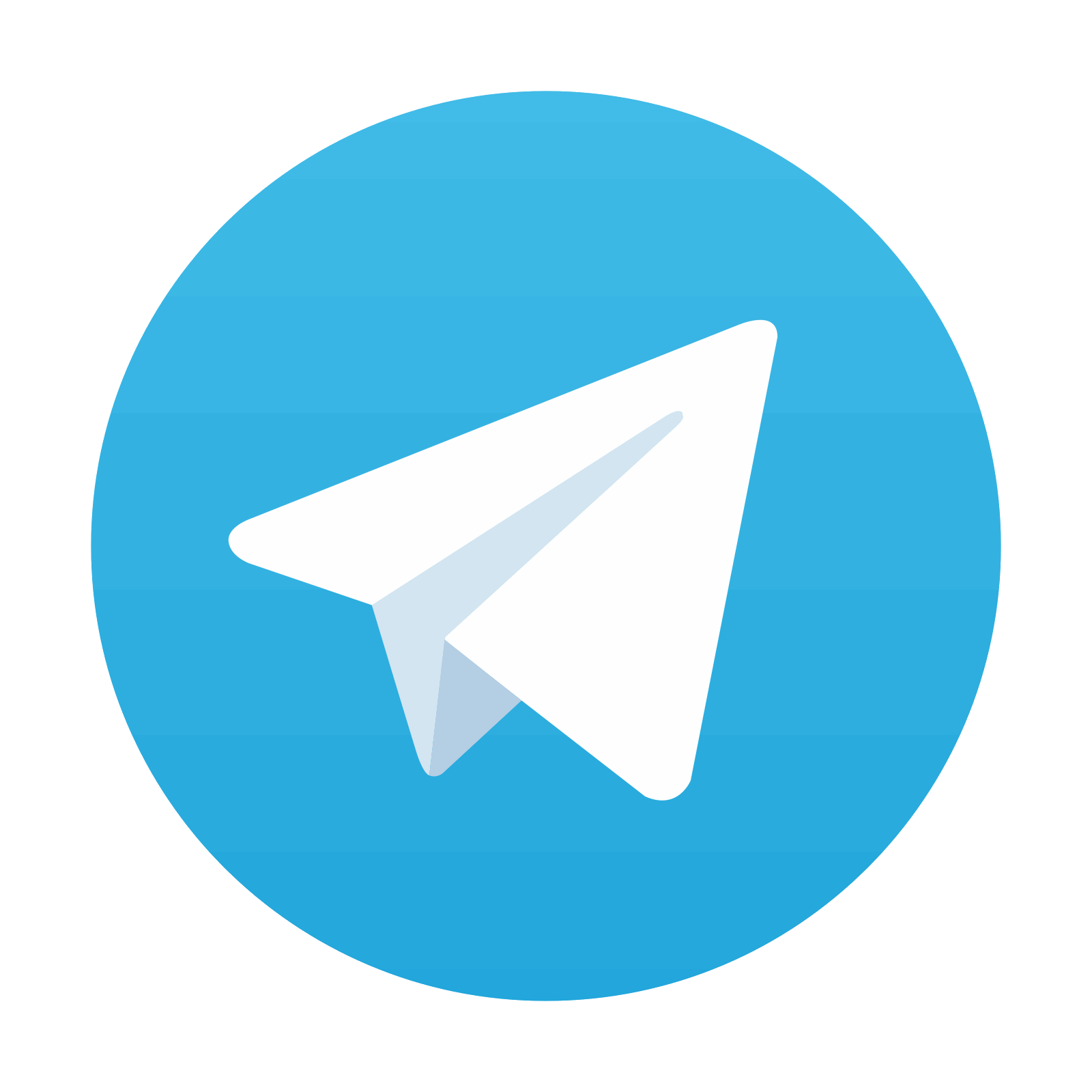
Stay updated, free articles. Join our Telegram channel

Full access? Get Clinical Tree
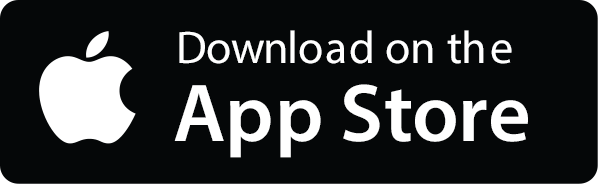
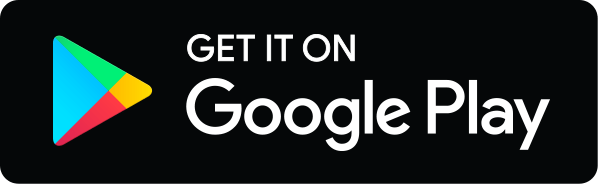