Fig. 1
Biocontinuum of adverse early and late effects (with permission from Rubin and Casarett 1968)
The primary effect of radiation toxicity is alterations in the rectal mucosa. Early radiation-induced rectal damage includes mucosal cell loss, acute inflammation in the lamina propria, eosinophilic crypt abscesses, and endothelial swelling in the arterioles which may progress to fibrosis of connective tissue and endarteritis of the arterioles resulting in rectal tissue ischemia, mucosal friability, bleeding, ulcers, strictures, and fistulae (Babb 1996). Late rectal toxicity is manifested by a progressive vasculitis leading to thrombosis of small arteries and arterioles resulting in varying degrees of ischemia of the bowel wall (Donner 1998). Molecular changes associated with acute and chronic radiation-induced bowel toxicity are being investigated to better understand the etiology and provide targets for future therapies which could ameliorate symptoms and improve quality of life.
Sophisticated treatment planning software has provided the means to further define rectal wall dose volume constraints enabling physicians and physicists the ability to design dose escalation studies which minimize rectal toxicity.
The Biocontinuum of adverse early and late effects are shown in Fig. 1.
2 Anatomy and Histology
2.1 Gross Anatomy (Normal)
The rectum and anus are the terminus of the digestive tract. The rectum, from the Latin rectum intestinum or straight intestine, begins at the terminus of the sigmoid colon approximately 12–14 cm cepahalad from the anal verge (Fig. 2). The rectum, a tube like structure with many layers, is normally empty until stool enters from the sigmoid colon and dilates near its terminus to form the rectal ampulla. The upper 1/3 of the rectum is covered by peritoneum. The rectum functions not only as a conduit organ but also as a reservoir of feces (Shafik et al. 2006). The rectum lies just anterior to the sacrum and posterior to the bladder and prostate in men, and vagina in women (Fig. 2).
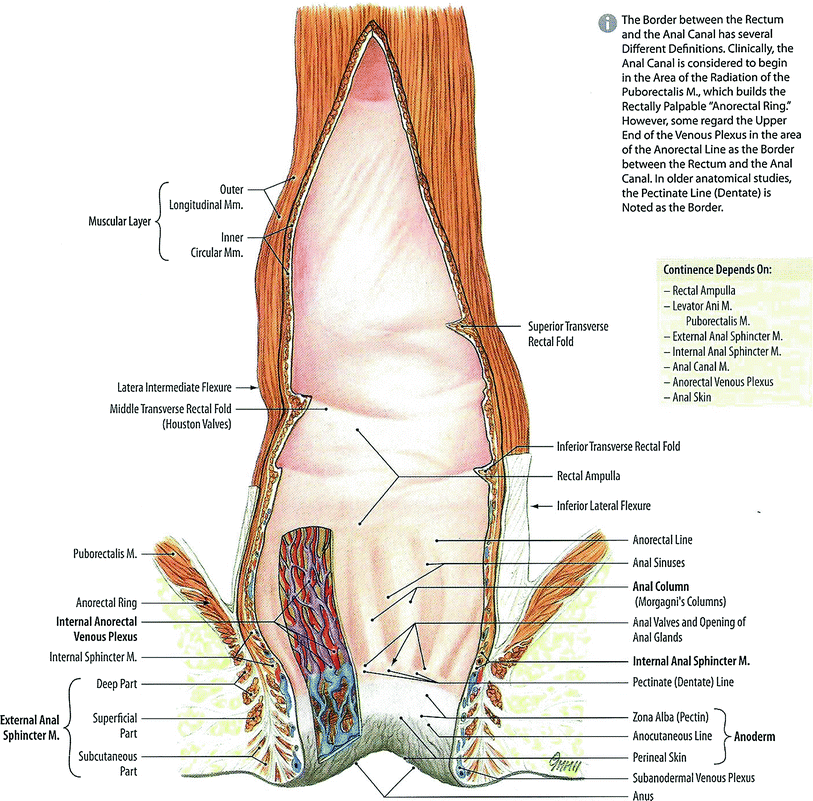
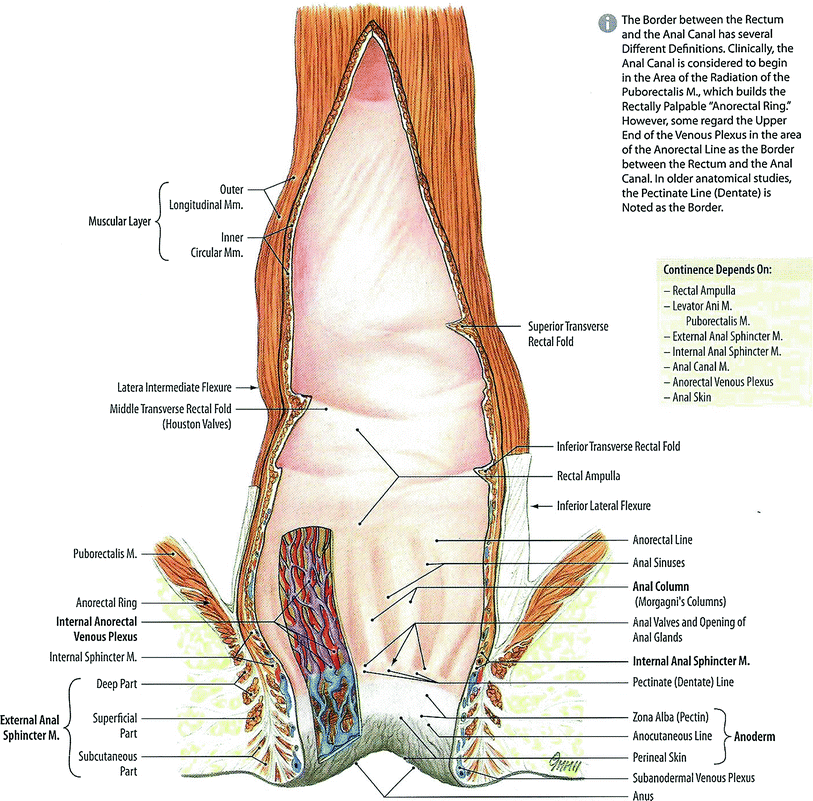
Fig. 2
Anatomy of normal rectum and rectal anal structure (with permission from Tillman 2007)
The junction of the sigmoid colon and rectum is not well defined but is marked by anatomic changes that distinguish the transition between the two organs. First, externally there is a change in the peritoneal relationship, direction, and musculature. The sigmoid colon can be considered to start at the superior aperture of the lesser pelvis. It passes anteriorly to the sacrum to the left side of the pelvis and curves on itself turning to the right reaching midline at the 3rd sacral vertebrae extending downward losing its mesentery to transition into the rectum. It is covered entirely by peritoneum and innvervated by pelvic splanchnic nerves for parasympathetic innervations while lumbar splanchnic nerves provide sympathetic innervations via the inferior mesenteric ganglion. The rectum follows the sacrum extending inferiorly ending in the rectum. The upper third of the rectum is covered on its front and sides by peritoneum while the middle aspect of the rectum is only covered on the front by peritoneum and the lower third of the rectum is without peritoneum. The rectum contains three and sometimes more lateral curvatures corresponding to the transverse rectal folds. The rectum is without mesentery and haustra and has an almost complete outer longitudinal muscular coat instead of teniae. Second, internally there can be a change in the character of the lining mucosa with the fluffy, rugose mucosa of the colon giving way to the smooth mucosa of the rectum. This, however, may not be apparent in the non-distended bowel (Hollinshead 1974). The inferior part of the rectum is readily distensible and is known as the rectal ampulla. Caudally, the rectum extends to the perineum, where the rectum turns sharply backward and downward through the pelvic diaphragm giving rise to the anal canal.
The rectum is surrounded by a filmy layer of connective tissue forming a tubular sheath called the rectal fascia. Superiorly, the rectal fascia becomes continuous with the subperitoneal connective tissue of the sigmoid. Transverse rectal folds project into the rectum and consist of mucosa, submucosa, and circular muscles and help to support rectal contents.
The arterial supply of the rectum comes primarily from the superior rectal artery with the inferior part of the rectum receiving blood supply from the middle rectal arteries. The venous drainage of the rectum follows the arterial supply. The lymphatics primarily drain superiorly as well paralleling the superior rectal blood vessels and emptying into the internal iliac lymph nodes. The lymphatics of the inferior third of the rectum are continuous with the lymphatics of the superior part of the anal canal and may drain into the superficial inguinal lymph nodes. The nerve supply of the rectum is through the rectal plexus which is a derivative of the inferior mesenteric plexus. Sensory fibers traveling in the lumbar splanchnic nerves are responsible for pain and the pudendal nerve is responsible for the control of emptying the bowel. Therefore the afferent and efferent side of the rectum receives its innervation through the pelvic splanchnic nerves which exit at approximately the second through fourth sacral nerves (Hollinshead 1974).
2.2 Histology and the Functional Subunit
The mucosa of the large intestines, including the rectum, has a simple columnar epithelium shaped into straight tubular crypts without villi as the majority of nutrient absorption occurs in the small intestines. The major function of the large intestines is to absorb water while the rectum is the repository of feces prior to evacuation. In addition, the mucosa of the large intestines is thicker than the small intestine, its crypts do not contain any Paneth cells, but it has more goblet cells. The proportion of goblet cells increases from the proximal colon to the rectum (Fig. 3).
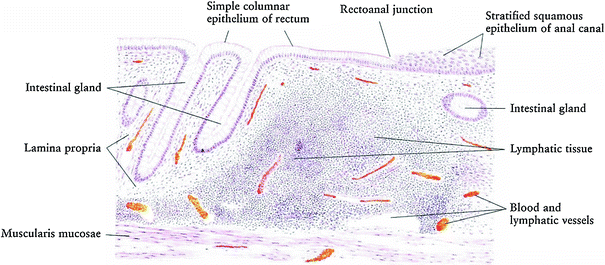
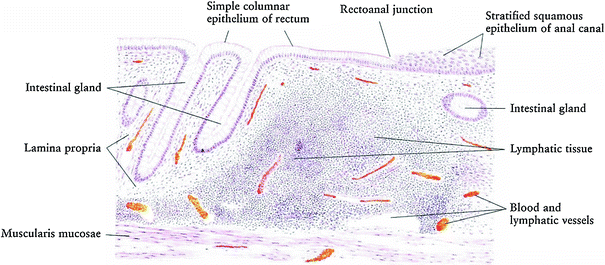
Fig. 3
Histology of normal rectum and rectal anal structure (with permission from Zhang 1999)
Cell migration occurs, with epithelial cells dividing in the deeper half of the crypts and migrating to the surface. The crypts are separated by lamina propria composed of loose connective tissue infiltrated by white blood cells, capillaries, and thin strands of smooth muscle (Fig. 3).
The muscularis mucosa in the rectum is very thin only a few muscle fibers in thickness beneath the deep ends of the crypts. The muscularis externa consists of an inner circular and outer longitudinal smooth muscle band. The outer longitudinal band is collected into 3 flat bands known as the teniae coli. The rectum does not have an outer layer or serosa but has an adventitia.
3 Biology, Physiology, and Pathophysiology
3.1 Molecular Biology Mechanisms of RT-Induced Rectal Injury
The alimentary tract has the same embryological route of development involving all three germ layers of the developing embryo, therefore, it is hypothesized the mechanism for mucosal injury along the alimentary tract is the same or similar regardless of location (Yeoh et al. 2007; Keefe 2004; Keefe et al. 2004). Originally, it was thought that radiation induced toxicity via cytotoxic effects on the basal cells of the epithelium (Spijkervet et al. 1991; Stiff 2001). Further study, however, determined the primary damage response occurred in endothelial cells (Menendez et al. 1998; Maj et al. 2003; Paris et al. 2001; Garcia-Barros et al. 2003). Molecularly, NF-KB and COX-2 play important roles in the pathophysiology of radiation induced colitis (Yeoh 2004; Yeoh et al. 2006) (Fig. 4).
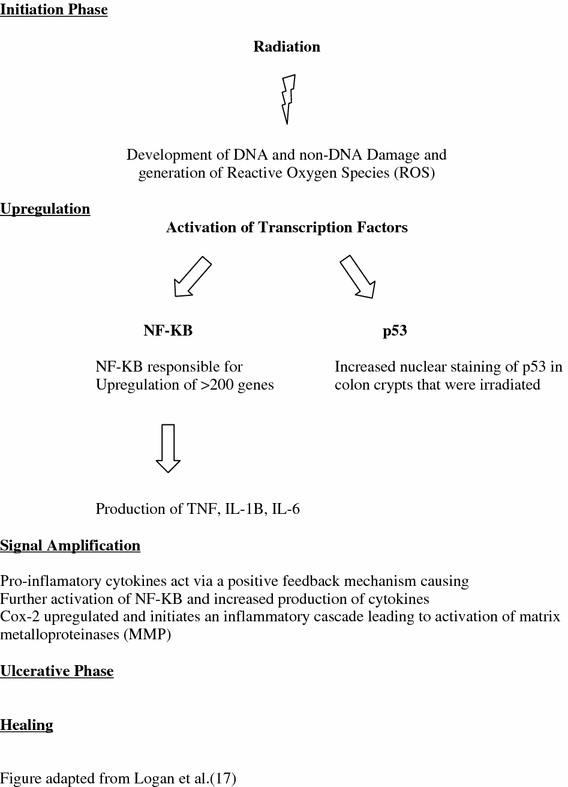
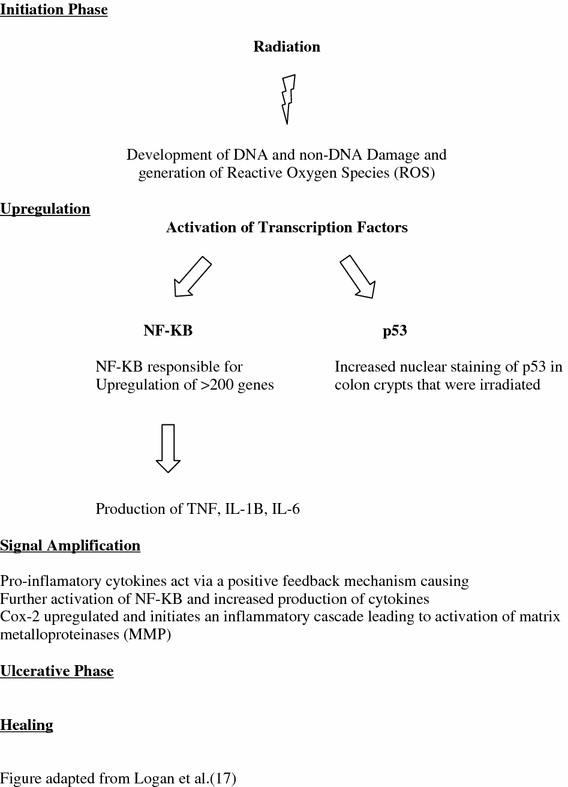
Fig. 4
The four stage model proposed by Sonis et al. outlining the molecular changes developing in the bowel acutely as a response to radiation (figure adapted from Logan 2007)
A four-stage model has been proposed by Sonis to describe the development of radiation-induced mucositis (Fig. 4) (Sonis 1998, 2004; Sonis et al. 2000, 2004). The four stage are initiation, upregulation, amplification/signaling, and finally ulceration. Although acute rectal toxicity of diarrhea and frequent stooling can be distressing to a patient while undergoing treatment, it is the late effects of radiation that are most problematic clinically, especially in a patient cured of their cancer. The majority of studies investigating radiation induced mucositis along the alimentary tract have focused on acute mucosal injury and its associated molecular changes. Since acute rectal symptoms predict for late rectal toxicity, acute molecular changes could be a proxy for late molecular changes. As discussed below, fibrosis and sclerosis of blood vessels, a histologic hallmark of late radiation change, were some of the distinct changes observed with accompanying increased expression of NF-KB within the blood vessel walls (Yeoh 2004). The acute radiation model is illustrated below.
3.1.1 Sonis Model
1.
Initiation Phase
Radiation causes the creation of reactive oxygen species as well as direct DNA and non-DNA damage.
2.
Upregulation and Message Generation Phase
Radiation-induced tissue damage as a result of the initiation phase results in the activation of a number of transcription factors including NF-KB. NF-KB is the “gatekeeper” functioning as the “on/off” switch in the pathogenesis of radiation-induced mucositis (Yeoh et al. 2006). Reactive oxygen species cause the degradation of IKB-a, which activates NK-KB moving from the cytoplasm to the nucleus to further activate genes playing a significant role in mucosal injury (Lee et al. 1998). NF-KB is responsible for upregulation of over 200 genes affecting mucosa integrity by inducing clonogenic cell death, apoptosis, and tissue injury (Logan et al. 2007; Sonis 2004). Apoptotic counts were also found to be increased in colon cells irradiated compared to nonirradiated colon cells (Yeoh et al. 2006, 2007). Staining of p53 was also increased in irradiated crypt cells compared to un-irradiated controls (Yeoh et al. 2007).
NK-KB activation results in the production of pro-inflammatory cytokines, including tumor necrosis factor (TNF), interleukin-1B (IL-1B), and interleukin-6 (Il-6) Logan et al. (2007; Sonis et al. 2000, 2004; Sonis 2004).
3.
Signal Amplification
Cytokines produce earlier amplification of the primary signal or may activate NF-KB in other cells. TNF can work in a positive feedback loop causing further activation of NF-KB. Gene transcription occurs generating biologically active proteins mitogen-activated protein kinase (MAPK) and cyclooxygenase-2 (Cox-2). The inflammatory cascade is initiated leading to activation of matrix metalloproteinases further eliciting tissue damage.
4.
Ulcerative Phase
Characterized by the clinically overt presence of an ulcer, breakdown of the mucosal barrier can lead to bacterial colonization and associated products that can stimulate further amplification of cytokine production and further tissue injury.
5.
Healing
Following radiation, reepithelialization of the mucosa may occur due to signals from the extracellular matrix. The healing phase is probably the least well understood and studied with respect to mucositis pathobiology (Logan et al. 2007). The healed mucosa however has a persistently altered structure (based on both ultrastructural and histological studies) that compromises its ability to withstand further trauma or insults, a phenomenon originally observed by Rubin and Casarett in 1968 (Rubin and Casarett 1968).
Recently, plasminogen activator inhibitor type 1 (PAI-1) has been found to play a critical role in radiation-induced intestinal damage. Up-regulation of PAI-1 in the endothelium was found in mice as well as patients with radiation-induced damage (Milliat et al. 2008). A p53/Smad3-dependent mechanism was responsible for the increased PAI-1 expression. C-reactive protein, however, was not a prominent feature in uncomplicated chronic-radiation-induced toxicity (Khalid et al. 2007). The relationship between hypoxia expression and radiation-induced late rectal toxicity was studied in C57BL/6 N mice having 25 Gy delivered to the rectum in a single fraction. Expression of transforming growth factor beta1 (TGF-beta1), hypoxia-inducible factor-1alpha (HIF1-alpha), vascular endothelial growth factor (VEGF) and endothelial cell marker CD31 increased significantly with the formation of fibrosis induced by radiation compared to unirradiated controls (Liu et al. 2008). Maximum expression of TGF-beta1, HIF-1alpha, and VEGF was found 14, 30, and 90 days after irradiation, respectively, consistent with the dynamic change of fibrosis.
3.2 Cellular Dynamics and the Radiation Response
Acutely, radiation impairs the proliferative ability of the intestinal mucosa resulting in a deficit in cell repopulation while the differentiated cells continue to shed. The continued cell loss without repopulation results in tissue hypoplasia. The lamina propria is then infiltrated by a plasma cells, neutrophils, and eosinophils. Crypt abscesses and sloughed epithelial cells could also be present. Radiation could also cause crypt disintegration with disorganization and loss of goblet cells. The muscularis mucosa at the base of the lamina propria can be fibrosed and disorganized with leukocyte infiltration (Yeoh et al. 2005). Telangeictatic vessel formation and fibrosis and sclerosis of the blood vessels were principal features in the submucosa with increased density and thickening of the collagenous fibers making up the submucosa (Yeoh et al. 2005). The circular and longitudinal muscle layers can be fibrosed with a neutrophil infiltration into the muscle layers. The fat layer surrounding the rectum showed evidence of fibrosis and sclerosis of the blood vessels with telangiectatic vessels present (Yeoh et al. 2005). The degree of goblet cell damage is proportional to the radiation dose. Yeoh et al. found less goblet cell degeneration further away from the area of the primary tumor that had received a lower radiation dose (Yeoh et al. 2005). Mucin secreted by the goblet cells is also affected with a mixed borderline to normal pattern of mucin differentiation the further away from the tumor compared to sialomucins predominate with little sulfomucin apparent characteristic of an abnormal mucin pattern closer to the primary area of irradiation. This corresponds to the goblet cell composition changing from more acidic to more neutral (Yeoh et al. 2007). Tissue hypoplasia with the cellular reaction is responsible for the decreased intestinal function and decreased stool caliber leading to a more watery stool or mucoid diarrhea (Zimmermann and Feldmann 1998).
Chronic radiation injury to the bowel is manifested by a progressive vasculitis leading to thrombosis of small arteries and arterioles resulting in varying degrees of ischemia of the bowel wall (Donner 1998). Figure 5a is a photomicrograph showing fibrin thrombi in a vessel in a patient with late radiation-induced rectal toxicity. The arteritis and submucosal fibrosis can lead to structuring and obstruction. Severe ischemia can lead to necrosis, deep ulceration, and fistula formation. Telangiectasis and neovascularity form later as a result of a network of collateral small vessels which are superficial and fragile, susceptible to trauma resulting in bleeding. The abnormal blood supply to the mucosa results in regenerating crypts and may lead to distortion and atypia of the epithelial cell lining resembling dysplasia (Donner 1998). The lamina propria can become fibrotic. Collagen can become deposited below the surface epithelium leading to ulceration, mucosal sloughing, and fistulization. Trauma to the fragile neovasculature results in chronic low grade rectal bleeding leading to iron deficiency anemia (Buchi and Dixon 1987).
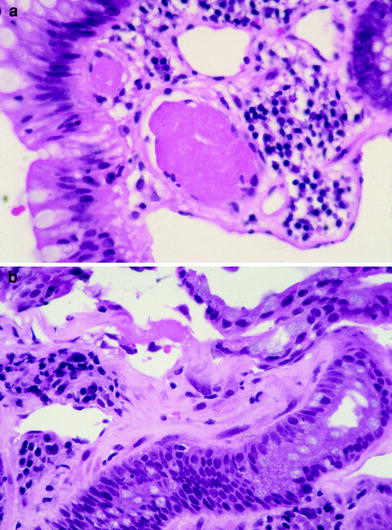
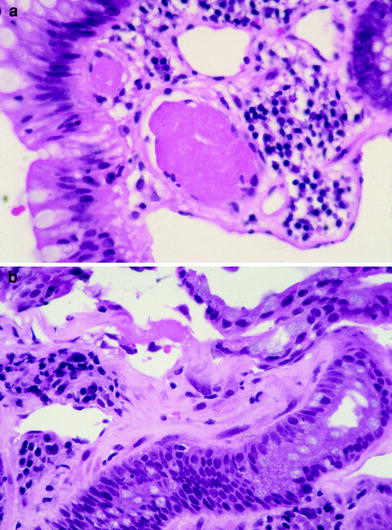
Fig. 5
a Photomicrograph of a fibrin thrombi in a small blood vessel characteristic of chronic radiation proctitis. b Photomicrograph showing submucosal fibrosis in the rectum of a patient with chronic radiation proctitis (courtesy of Dr. Harry Cooper, Department of Pathology, Fox Chase Cancer Center)
The ability of the mucosa to regenerate is impaired resulting in a thin and vulnerable mucosa with atypical epithelial cells. Small abscesses can be found in the deep crypts with large foam cells seen beneath the intima considered to be evidence of radiation vascular injury. The submucosa becomes thickened and fibrotic containing large, bizarre-formed radiation fibroblasts with the muscularis propria exhibiting focal areas of fibrosis or involvement of deep fissures or penetrating ulcers (Fig. 5b). The serosa can develop diffuse hyaline changes with fibroblasts, telangiectasia or small vessels, and ischemic changes in larger vessels resulting in an opaque, grayish, and thickened tissue surrounding the intestinal wall (Zimmermann and Feldmann 1998).
3.3 Physiology and Pathophysiology
The rectum is not only a conduit organ but also a reservoir (Shafik et al. 2006). The pelvic splanchnic nerves, responsible for emptying the bowel, increase the peristaltic activity of the rectum and with the help of increased abdominal pressure move the feces out of the rectum. Voluntary muscle activation of the muscles around the anal canal restrains bowel movements. If the increase in abdominal pressure is great enough, voluntary contraction of the external anal sphincter may also be initiated but cannot be maintained for long time periods. This reflex activity depends upon the presence of a part of the rectum; if the rectum is entirely removed, the passage of gas or feces can be resisted only by the rather incompetent voluntary contraction of the external anal sphincter. Patients with coloanal anastomosis may have incontinence but patients with the lower 2 cm of the rectum remaining can have good control of the passage of gas and feces (Hollinshead 1974).
Diarrhea can be a result of an enteroenteric or enterocolonic fistula, stricture formation resulting in stasis and bacterial overgrowth, and from direct injury of the colonic mucosa with a collagenous colitis-type syndrome interfering with absorption of fluids (Donner 1998). The pathophysiology of abnormal bowel function was studied in 30 randomly selected women treated with radiation for carcinoma of the cervix. Bile acid, vitamin B12 and lactose absorption were less in patients receiving radiation. Bile acid absorption was below the control range in 14 of 30 patients and dietary calcium intake was lower in those patients with lactose malabsorption Yeoh et al. (1993). In addition, gastric emptying and whole gut transit were inversely related to stool frequency. Either bowel frequency, bile acid absorption, vitamin B12 absorption was outside the control range in 19 of 30 patients (Yeoh et al. 1993). Stool weight was also greater and whole-gut transit faster 1–2 years after patients received both pelvic and abdominal radiation (Yeoh et al. 1993).
The pathophysiology of incontinence is not well understood. Although patients may be incontinent, they may not exhibit any change in the internal or external sphincter (Petersen et al. 2007). Yeoh et al. found no significant change in the thickness of the internal or external anal sphincter 6 weeks after radiation (Yeoh et al. 1998). The clinical correlates of incontinence can be differentiated into two groups, core factors and associated factors. Reduced anal resting tone is considered as a core factor of incontinence. Further clinical signs of radiation-induced incontinence are a reduction in squeeze pressure and decrease in rectal wall stretch (Petersen et al. 2007; Yeoh et al. 1998; Berndtsson et al. 2002).
Two of the most important factors in the development of fecal incontinence are connective tissue remodeling of the rectum and diminished neural function of the continence organ. Smooth muscle hypertrophy and myenteric plexus damage may contribute to the reduction in rectal volumes at sensory threshold, constant sensation and maximal tolerance, and in rectal compliance (Varma et al. 1985, 1986). Lumbosacral plexopathy has been implicated in fecal incontinence after pelvic radiotherapy (Iglicki et al. 1996; Georgiou et al. 1993). In addition, a significant change in the density of the nerves in the internal anal sphincter was seen as early as 6–12 months after radiotherapy reflecting myenteric plexus injury (Da Silva et al. 2003). Further strengthening the argument in favor of nerve damage, Kushwaha et al. found an appreciable decrease in the electrosensitivity of the rectal wall in 31 patients receiving radiotherapy for prostate or bladder cancer (Kushwaha et al. 2003). Recently, causes of fecal and urinary incontinence were studied in cadavers. Cadaver total mesorectal excision (TME), surgery demonstrated that, especially in low tumors, the pelvic floor innervation can be damaged (Wallner et al. 2008).
A number of patient-associated factors have been linked to a higher rate of radiation proctitis including young age, previous abdominal surgery, hypertension, vasculopathy, diabetes, and hemorrhoids (Tagkalidis and Tjandra 2001; Potish et al. 1979; Gilinsky et al. 1983; Lanciano et al. 1992). Other patient factors include inflammatory bowel disease, connective tissue disorder, previous acute radiation proctopathy, and ataxia-telangiectasia gene (Garg et al. 2006).
4 Clinical Syndromes
4.1 Detection (Symptoms)
Acute proctitis can occur in the latter half of fractionated radiation regimens and are often expressed with symptoms of diarrhea and accompanied by pain depending on the severity of the mucositis (Figs. 5 and 6, Tables 1 and 2).
Table 1
LENT SOMA. Clinical syndromes
GRADE 1 | GRADE 2 | GRADE 3 | GRADE 4 | |
---|---|---|---|---|
Subjective | ||||
Tenesmus | Occasional urgency | Intermittent urgency | Persistent urgency | Refractory |
Mucosal loss | Occasional | Intermittent | Persistent | Refractory |
Sphincter control | Occasional | Intermittent | Persistent | Refractory |
Stool frequency | 2–4/day | 4–8/day | >8/day | Uncontrolled diarrhea |
Pain | Occasional & minimal | Intermittent & tolerable | Persistent & intense | Refractory & excruciating |
Objective | ||||
Bleeding | Occult | Occasionally >2/week | Persistent/daily | Gross hemorrhage |
Ulceration | Superficial ≥1 cm2 | Deep ulcer | Perforation, Fistulae | |
Stricture | >2/3 normal diameter with dilatation | 1/3–2/3 normal diameter with dilatation | <1/3 normal diameter | Complete obstruction |
Management | ||||
Tenesmus & stool frequency | Occasional, ≥2 antidiarrheals/week | Regular, >2 antidiarrheals/week | Multiple, >2 antidiarrheals/day | Surgical intervention/Permanent colostomy |
Pain | Occasional non-narcotic | Regular non-narcotic | Regular narcotic | Surgical intervention |
Bleeding | Stool softener, iron therapy | Occasional transfusion | Frequent transfusions | Surgical intervention/Permanent colostomy |
Ulceration | Diet modification, stool softener | Occasional dilatation | Steroids per enema, hyperbaric oxygen | Surgical intervention/Permanent colostomy |
Sphincter control | Occasional use of incontinence pads | Intermittent use of incontinence pads | Persistent use of incontinence pads | Surgical intervention/Permanent colostomy |
Analytic | ||||
Barium enema | Assessment of lumen and peristalsis | |||
Proctoscopy | Assessment of lumen and mucosal surface | |||
CT | Assessment of wall thickness, sinus, and fistula formation | |||
MRI | Assessment of wall thickness, sinus, and fistula formation | |||
Anal manometry | Assessment rectal compliance | |||
Ultrasound | Assessment of wall thickness, sinus, and fistula formation |
Table 2
Late injury to the rectum/anus may be broadly, and somewhat arbitrarily, segregated into categories as shown
Focal | Global | |
---|---|---|
Subclinical | 1. Telangiectasia 2. Asymptomatic stricture via endoscopy or radiographs 3. Thickened bowel wall by imaging | 1. Asymptomatic abnormalities in anorectal manometry |
Clinical | 1. Bleeding, ulceration 2. Stricture | 1. Incontinence 2. Constipation |
Chronic radiation proctitis typically occur anywhere from 8 to 13 months after completion of treatment (Tagkalidis and Tjandra 2001) (Fig. 5a). Bleeding is the most common symptom of chronic radiation proctitis compared to acute radiation proctitis where bleeding is relatively uncommon. Other symptoms of chronic radiation proctitis include mucous discharge, urgency, fecal incontinence, diarrhea, and pain (Zimmermann and Feldmann 1998). Constipation can occur in the presence of a stricture most notably in the sigmoid colon region usually occurring as a result of an intracavitary implant in the treatment of cervical cancer or endometrial cancer. Fibrosis can also be seen following RT (Fig. 5b).
The Radiation Therapy Oncology Group/European Organization for Research and Treatment of Cancer (RTOG/EORTC), Late Effects of Normal Tissue (LENT) and the Common Terminology Criteria for Adverse Events (CTCAE) version 3.0 are the three most widely used scoring systems use to grade late toxicity.
The removal of the separation between acute and late radiation effects was made when CTCAE v3.0 was adopted. An event is defined and graded relative to the time the evaluation was performed but CTCAE v3.0 does not separate criteria by acute or chronic. The CTCAE v3.0 criteria classify symptoms along the GI tract and not by anatomic region. http://ctep.cancer.gov/protocolDevelopment/electronic_applications/docs/ctcaev3.pdf
The LENT SOMA system provides a means to categorize and grade toxicities (Table 1) (Rubin et al. 1995). An alternative way to segregate endpoints are provided in Table 2.
Fecal incontinence after pelvic radiotherapy is poorly understood and potentially underreported occurring in perhaps 3–53 % of patients (Putta and Andreyev 2005). The underreporting may be due to patient’s embarrassment and associated failure to report incontinence and because prospective studies often fail to properly assess incontinence as a specific endpoint using validated and reproducible methodologies. Patients with prostate cancer may have a lower incidence of incontinence compared to patients with gynecologic, bladder, rectal, or anal cancer because of potentially lower dose volume parameters involving the anal sphincter. Most studies assessing anorectal function are generally not adequately controlled or powered, do not adequately assess anorectal function, and often fail to follow patients for extended time. Thus, the reported data are limited and suspect (Putta and Andreyev 2005).
4.1.1 Endoscopy
Flexible endoscopy is becoming the most frequently used diagnostic examination to treat and manage radiation proctitis. Flexible sigmoidoscopy or colonoscopy can be used to visualize the colon and assess for other etiologies of rectal bleeding or diarrhea. The classic endoscopic findings of radiation proctitis include prominent telangiectasia, erythema, and friability (Gilinsky et al. 1983; Reichelderfer and Morrisey 1980). A distinct margin can be detected between the normal rectal mucosa and the area affected by radiation. A typical endoscopic picture of radiation proctitis is shown in Fig. 6. Patients suspected of having a radiation reaction should not be biopsied. The trauma of the mucosa can lead to infection and/or a poorly healing wound.
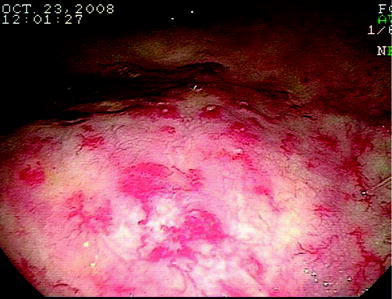
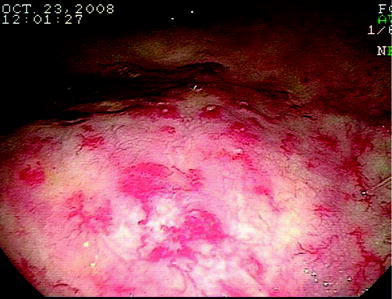
Fig. 6
An endoscopic view of the rectum showing multiple telengectasias of the rectum as a result of radiation
4.1.2 Functional Tests
Anorectal manometry is a common and widely used test to assess anorectal function (Sun and Rao 2003). Continuous fluid-inflation procometrograms have been used to evaluate the symptoms of chronic radiation proctitis in patients who received radiation. Significant reductions in rectal volumes at sensory threshold, constant sensation and maximal tolerance, and in rectal compliance are seen in patients treated with radiotherapy compared to age- and sex-matched controls who did not receive radiation (Varma et al. 1985).
4.2 Diagnosis: Imaging
4.2.1 Barium Enema
Double contrast barium enema, while standard in the past, has been replaced in the diagnosis of chronic proctitis by flexible sigmoidoscopy. Characteristic features of chronic radiation injury to the rectum seen in a barium enema include (Gilinsky et al. 1983) (Fig. 7a, b):
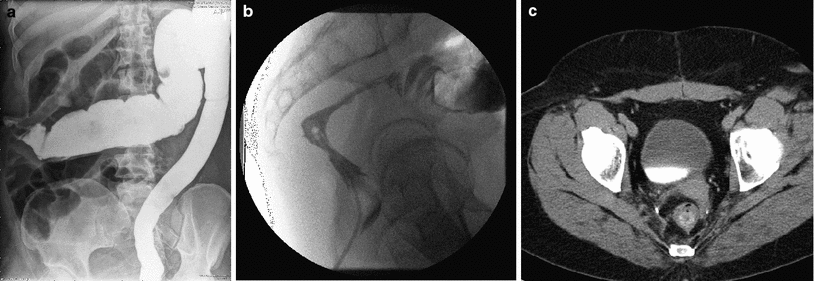
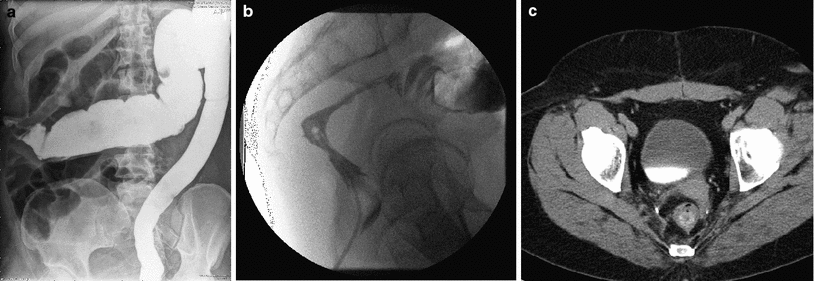
Fig. 7
a A barium enema of a patient who received post-operative radiation for adenocarcinoma of the rectum. The mucosal pattern is obliterated resulting in a smooth featureless tubular appearance of the rectum. b A stricture in the upper rectum as a result of radiation. c A CT image showing a thickened rectal wall in a patient having undergone a low anterior resection and postoperative radiation for an adenocarcinoma of the rectum
1.
Loss of distensibility of the rectum and/or sigmoid
2.
Fixed stenoses and stricture of various lengths and degrees of narrowing
3.
Shortening of the rectum and/or sigmoid, loss of normal rectal curves, and increase of retrorectal (presacral) space
4.
Mucosal pattern changes, including irregularities, pseudo-polypoidal protrusions, various degrees of obliteration of mucosa, sometimes resulting in a smooth, featureless tubular appearance of the rectum
5.
Irregularities of contour varying from tiny serrations representing shallow ulcerations to ragged marginations similar to those associated with malignancy
6.
Sinuses into the pelvic tissue and fistulae to the bladder, vagina, and small bowel.
4.2.2 Computed Tomography/Magnetic Resonance Imaging
Computed tomography (CT) can be helpful in the diagnosis of radiation proctitis. CT scans can show a thickened rectal wall and dilated proximal loops of bowel (Chen et al. 2003). CT and magnetic resonance imaging (MRI) are more sensitive in identifying changes in the surrounding tissue compared to barium enemas. MRI is more sensitive, however, in identifying early radiation changes (Rubin et al. 2002). T2-weighted images show increased signal intensity of the submucosa and inner muscle layer with a normal appearance of the outer muscle layer. The outer muscle layer of the rectum will develop increased signal with time on T2-weighted images. (Rubin et al. 2002) Fig. 7c is a CT scan of a patient who received postoperative radiotherapy for rectal cancer with radiation proctitis.
5 Radiation Tolerance: Predicting RT-Induced Rectal Injury
Radiation proctitis comprises a spectrum of symptoms ranging from rectal bleeding, mucus discharge, incontinence and fecal leakage. The TD 5/5 (probability of 5 % complication in 5 years) and TD 50/5 (probability of 50 % complication within 5 years) from Rubin and Casarett’s original work (1968) was updated by Emami and Lymen et al. with volume of tissue incorporated into the analysis. The toxicity endpoint selected for rectal toxicity was severe proctitis, necrosis, fistula, or stenosis. A volume effect did not exist for 1/3 and 2/3 of the organ volume for the TD 5/5 and TD 50/5 if the volume irradiated was 100 cm3 (Emami et al. 1991). The whole organ TD 5/5 and 50/5 was 6000 cGy and 8000 cGy, respectively (Emami et al. 1991). Unfortunately, because of the paucity of data, the clinical judgment of the physician on the normal tissue task force was used to generate these values.
A clearer picture of rectal tolerance has emerged with dose escalation trials for prostate cancer and improved radiation therapy treatment planning systems with the ability to calculate dose volume histograms. The greatest literature identifying risk factors for chronic radiation toxicity originates from reports of cervical and prostate cancer patients treated with radiation. The literature is sparse, however, detailing risk factors for chronic radiation toxicity for patients with rectal cancer treated with pre or postoperative radiation. This may be a result of the relatively lose doses used with pre and postoperative treatment. Large radiation treatment volumes and techniques were, however, mentioned as risk factors for late rectal toxicity (Glimelius et al. 2003).
5.1 Dose Time Fractionation
5.1.1 Cervix Cancer
Severe bladder and rectal complications occurred when the external beam dose was greater than 50 Gy in patients with Stages IIIA B cervical cancer treated with external beam radiation and 2 intracavitary implants (Stryker et al. 1988). It was recommended the whole pelvis dose should not exceed 40–45 Gy when combined with 2 intracavitary implants. There is controversy, however, if time dose factors (TDF) are able to predict late normal tissue reactions in patients with cervical cancer. TDF did not correctly predict late normal tissue reactions with increasing complication rates noted in patients receiving increased dose per fraction of external beam (Deore et al. 1992). Ogino et al. however, found both TDF and biologically effective dose (BED) values to significantly correlate with grade 4 rectal toxicity. Grade 4 rectal toxicity was not seen with a TDF < 130 or BED < 147 (Ogino et al. 1995). Ferrigno et al. reported patients with a cumulative BED > 110 Gy had a higher but not statistically significant rectal complication rate (Ferrigno et al. 2001). A 19.6 % rate of late rectal sequelae was noted when the cumulative BED was <110 compared to 36.4 % when the cumulative BED was >110 Gy (Chen et al. 2000). In another study, late rectal complications >Grade 2 were 5.4 % with a BED < 125 Gy compared to 36.1 % with a BED >125 Gy (Hyun Kim et al. 2005). Rectal dose in comparison to point A has also been reported to be a prognostic factor for patients with late rectal complications. Late rectal complications were noted in 9/13 patients when the rectal dose was greater than the prescribed dose to point A compared to 7/30 when the rectal dose was less than the prescribed dose (Clark et al. 1994). A greater rate of late rectal complications was also noted if the percent intracavitary dose to point A was 80 % or more (Shin et al. 1999) (Fig. 8a).
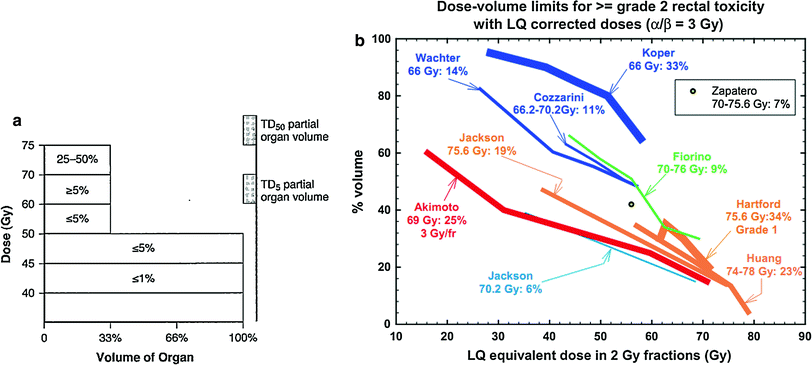
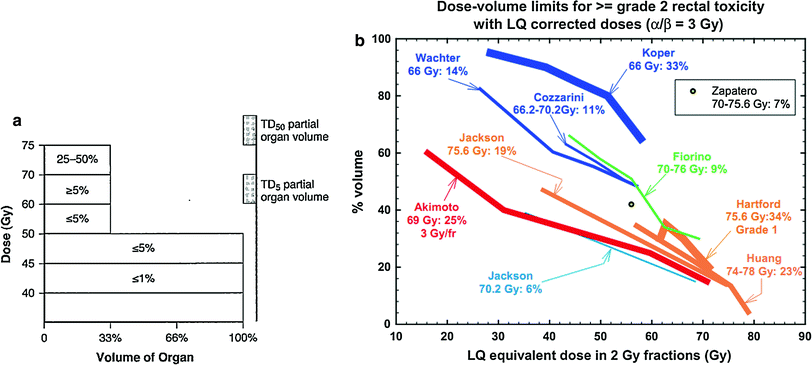
Fig. 8
a Dose–volume histogram for late effects seen in the gastrointestinal tract, illustrating the relationship between volume and tolerance doses. The right y-axis indicates the tolerance dose ranges for the TD5 and TD50 for partial organ volume (POV) irradiation. (Modified from Rubin et al. 2001 with permissions) b Dose–volume histogram thresholds found to be significantly associated with Grade ≥2 rectal toxicity. Thicker lines indicate higher rates of rates of overall toxicity (percentages are indicated on the graph along with the physical prescription dose). Threshold doses are expressed as linear-quadratic equivalent doses delivered in 2-Gy fractions, calculated using α/β = 3 Gy. The associated linear-quadratic equivalent prescription doses are coded by spectrum from lowest (blue), to highest (red). Volumes shown in the graph are based on the full length of the anatomic rectum. Curves for Huang and Wachter were adjusted downward by 15 % and by 50 % for Hartford, to account for the different definitions used for rectal volume. Dose–volume data from multiple centers converge at the high dose range, implying that these values are more consistently associated with toxicity. Abbreviations: LQ = linear quadratic (with permission from the Quantec review, Michalski et al. 2010)
With High dose rate (HDR), the dose per fraction was shown in multivariate analysis to be a significant factor influencing proctitis rate. Patients treated with 7.2 Gy for 3 fractions had a greater proctitis rate compared to patients receiving 4.8 Gy for 5 fractions (Wang et al. 2004). Higher complication rates were noted when the intracavitary fractional dose was ≥3.2 Gy or with a total intracavitary dose of ≥20 Gy or more (Shin et al. 1999).
5.2 Dose/Volume Constraints
Table 3 depicts the dose recommendations for patients with cervical cancer undergoing external beam radiotherapy and intracavitary implants. Most data from these studies were collected prior to the 3D-CRT era. These studies combine external beam radiation therapy and HDR intracavitary doses; the intracavitary fractionation schedules were variable. In addition, the late rectal endpoints were measured with different toxicity scales. Koom et al. correlated DVH parameters from 3D-CRT gynecologic brachytherapy planning with the rectosigmoid mucosal changes observed on flexible sigmoidoscopy (Koom et al. 2007). The degree of mucosal change was related to DVH parameters and International Federation of Gynecology and Obstetrics (ICRU) rectal point (ICRUrp) (Koom et al. 2007). The endpoint of visual mucosal change is not part of conventional toxicity scales and is of unclear clinical significance (Fig. 8b, Tables 3 and 4).
Table 3
Dosimetric recommendations for the rectal wall to limit injury in patients irradiated for cervical cancer
Study | Dosimetric Recommendations |
---|---|
Ferrigno et al. (2001) | A higher but not statistically significant complication rate noted with a BED > 110 Gy |
Ogino et al. (1995) | Grade 0 toxicity- Mean BED 126 Gy s.d. 35.3 Gy |
Chen et al. (2000) | BED < 110 Gy 19.6 % late rectal complication rate BED > 110 Gy 36.4 % late rectal complication rate |
Kim et al. (2005) | BED < 125 Gy 5.4 % late rectal complication rate BED > 125 Gy 36.1 % late rectal complication rate |
Huang et al. (2004) | BED < 100 Gy and external beam parametrial dose of <54 Gy |
Table 4
Dose/volume constraints for the rectum suggested in patients receiving external beam radiation therapy for prostate cancer
Study | V40 | V50 | V60 | V65 | V70 | V75 |
---|---|---|---|---|---|---|
Rectal bleeding | ||||||
Fiorino et al. (2008) | <55 % | <40 % | <25 % | <55 | ||
Peeters et al. (2006) | <30 % | |||||
Incontinence/Fecal leakage | ||||||
al-Abany et al. (2005) | <40 % | |||||
Fiorino et al. (2008) | <65–70 % | |||||
Unspecified late rectal toxicity | ||||||
van der Laan et al. (2008) | <65 % | <55 % |