CHAPTER 2
Instrumentation and Quality Assurance
Summary of Important Points
DESIGN CHARACTERISTICS OF MAMMOGRAPHY UNITS
All mammography units are designed to image the soft tissue of the breast while displaying the necessary subtle contrast differences. All modern generators are high-frequency generators. Like the older units, these rectify the input to produce a direct current (DC) voltage waveform, but the modern high-frequency generators essentially provide a constant potential with about 1% ripple. The high frequency allows more efficient x-ray production and therefore produces a higher effective energy x-ray beam. The result is higher x-ray output for a given peak kilovoltage (kVp) and milliamperes (mA) setting.
Dedicated Mammography Unit
The breast consists of all soft tissue structure with very similar mass densities and atomic numbers. Because of the similarity in tissue, general radiography tubes cannot be used to image the breast tissue. In order to maximize the contrast at the highest possible resolution yet keeping radiation doses low, the general radiography systems had to be modified for mammography imaging.
Target Materials in Analog and Digital Mammography Units
• Breast tissue consists mostly of adipose, fibrous, and glandular tissue which all have relatively low atomic number. The energy of the x-ray produced must be just high enough to penetrate the tissue without overpenetration. The x-ray range found most useful in maximizing contrast in breast tissue is in the 17-24 keV range.
• Molybdenum targets produce characteristic x-rays in the 17-19 keV range after a photoelectric interaction. These are ideal when imaging fatty breast.
• The characteristic x-ray produced using rhodium targets are similar to that from molybdenum, but because rhodium has a slightly higher atomic number, more Bremsstrahlung x-rays are produced and the K-characteristic x-rays will be in the 20-23 keV range, which provides a better penetration of dense breast.
• With tungsten targets, Bremsstrahlung x-rays will predominate at energies above and below the 17-24 keV range. Although tungsten emits “harder” spectrum rays, the wider dynamic range can be utilized in digital imaging.
Common Target Material—Analog Units
• Molybdenum
• Rhodium
Common Target Material—Digital Units
• Rhodium
• Tungsten
• Some digital systems have a single anode target made of tungsten.
• Single track tubes can sometimes be more reliable and less expensive to manufacture.
• Single track x-ray tubes can support a higher anode heat loading therefore allowing for shorter exposure times when compared to dual track x-ray tubes. This is especially useful when imaging large breasts.
• Single track tungsten tubes can support 2-3 times the maximum anode load when compared to dual track tubes.
Beam Filtration
• Filtration is defined by the half-value layer (HVL). In mammography added filtration shapes the emission spectrums of the x-ray beam and makes it compatible with the image receptor and breast characteristics of each patient.
• Filtration will also improve the energy-distribution x-ray spectrum by selectively removing the very low energies that will be absorbed in the superficial layers of the skin and contribute to skin dose. Also, filtration will remove the higher energies outside of the desired range. With proper filtration, the x-ray beam entering the breast will have very few photons above or below the preferred mammographic energy range.
• In digital imaging, filtration will also help to reduce exposure time, eliminating potential motion artifacts.
• Minimum HVL is specified by regulations and should not measure less than 0.30 mm Al at 30 kVp or 0.25 mm at 25 kVp to ensure that the patient will not receive excessive radiation dose. The HVL also should not exceed 0.40 mm Al at 30 kVp to avoid excessive filtration that would reduce contrast by filtering out the low-energy photons necessary to penetrate the breast tissue.
• Any HVL assessment must include an assessment of both the inherent and added filtration.
Inherent or Added Filtration
As the x-ray beam leaves the target of the anode, it is filtered in 2 ways.
• Inherent filtration includes the glass or metal window of the x-ray tube, oil in the tube housing, the mirror of the collimation assembly and the compression paddle.
• Added filtration is usually an aluminum (Al) equivalent material, positioned in the path of the emerging beam thus affecting its penetrating power which is defined by HVL.
Compression Plate
The compression plate is made of a radiolucent material that will not attenuate the low photons necessary for breast imaging. The Mammography Quality Standard Act (MQSA) has specific standards regulating the shape of the compression device and semiannual tests are required to ensure that the compression device is working properly. MQSA standards:
• Minimum automatic compression should not be less than 25 lbs (111 N).
• Automatic compression should never exceed 45 lbs (200 N) on the initial power drive.
• All units should have a manual, hand-controlled compression device, controlled by the mammographer.
Material of the Exit Port
Beryllium (Be) is most often used because a regular glass or metallic window would harden the emerging beam by eliminating the low photons needed for breast imaging.
Common Target/Filtration Combinations
• In analog units, the filters used are often the same element as the x-ray tube target. Matching the filter to the same element as the x-ray tube target will allow the K-characteristic x-rays to pass while blocking the higher and lower energy Bremsstrahlung x-rays. Therefore with molybdenum and rhodium targets, the filtration is generally the same element.
• If the tube target is tungsten, rhodium (Rh) can be used for fatty breast and silver (Ag) for large or dense breast.
• Some tungsten targets use molybdenum (Mo) for fatty breast and rhodium (Rh) for dense breast.
• Aluminum (Al) is used as the filtration in the Hologic digital tomosynthesis units and low dose photon-counting imaging systems.
The Line-Focus Principle
• The focal spot is the area that electrons strike on the target.
• As the size of the focal spot decreases, the heating of the target is concentrated into a smaller and smaller area.
• In the design known as the line-focus principle, the target is angled to allow a larger area for the electrons to strike while maintaining a small effective focal spot.
• A large effective focal spot area on the target is therefore matched with a much smaller actual focal spot size.
Anode Heel Effect
• Portions of the x-ray beam at the anode side of the tube will pass through the anode, resulting in a lower intensity beam.
• To increase the chances of an even intensity beam across the breast, the thickest part of the breast (nearest the chest wall) is positioned at the cathode and the nipple area of the breast is positioned to the anode. This reduces the anode heel effect and allows more equal x-ray intensity across the detector.
Anode Angle and Tube Tilt
• Mammography tubes have anode angles ranging from 0-16 degrees depending on the manufacturer. A common tube tilt is 6 degrees.
• Tubes are positioned with a source to image-receptor distance (SID) of about 60-75 cm (24-30 in). Therefore, in order to achieve adequate field coverage for a field of view of 24 × 30 cm, and also to allow the central axis of the x-ray beam to run perpendicular to the plane of the detector at the chest wall, the tube must be tilted. Half-field geometry ensures that the x-ray beam does not extend beyond the edge of the detector especially at the chest wall.
• Decreasing the target angle will further decrease the effective focal spot.
Advantages of Tube Tilt
• Allows smaller target angle
• Allows greater anode heating capacity
• Reduces anode heel effect
Factors Affecting Focal Spot Size in Mammography
The size and shape of the focal spot can be affected by
• Size and shape of electron beam hitting anode
• Design and relationship of the filament coil to the focusing cup
• Angle of the anode
Focal Spot Sizes in Mammography
Most mammography units are dual focus, i.e., they have 2 focal spot sizes.
• The larger focal spot may be 0.4 but is generally 0.3 mm.
• The small focal spot ranges from 0.15-0.1 mm. The most common size is 0.1 mm.
Routine work utilizes the large focal spot size while the small focal spot size is used for magnification work.
Grids
• Grids are used to improve radiographic contrast by decreasing the amount of scattered radiation that reaches the image receptor.
• Modern mammography units utilize a moving grid to improve contrast.
• Grids are not used with magnification mammography, Hologic tomosynthesis, and photon-counting systems.
Grids and Exposure
All grids result in increased exposure and patient dose but will improve contrast. Because grid use necessitates an increase in radiation exposure and will therefore significantly increase the radiation dose to the patient, mammography grids are thinner than general x-ray grids. They also have a lower grid ratio than general radiography grids.
• The grid ratio of mammography grids usually varies from 3:1 to 5:1 (average of 4:1), focused to the SID for increased contrast. Grid frequencies of 30-50 lines per centimeter are common.
• The interspace material of the grid may be carbon fiber, wood, or other low-attenuation interspacing instead of the aluminum that is used in general radiography grids.
• High transmission cellular (HTC) grid is a specialized grid used in some mammography units. It has the characteristics of a crossed-grid capable of reducing scatter in 2 directions rather than 1. Copper is used instead of lead for the grid strip and air is used as the interspace material. This combination maintains a low radiation dose to the patient.
SYSTEM GEOMETRY (SID, OID, SOD, AND MAGNIFICATION)
SID is the source to image-receptor distance and refers to the distance from the x-ray source to the image receptor.
• SID is one of the many factors affecting recorded detail. The image loses recorded detail at short SID. In imaging, the aim is to have the smallest focal spot coupled with longest SID. In mammography the units have very low kVp coupled with very small focal spots, in order to reduce unsharpness. The units generally have relatively short SID of 60-75 cm (24-30 in).
OID is the object to image-receptor distance (OID) and refers to the distance the object (or body part) is from the image receptor.
• The OID should be as small as possible to minimize magnification.
• The only exception is the microfocus magnification techniques used in mammography imaging.
SOD is the source to object distance and refers to the distance of the source to the object or body part.
• The SOD should be as long as possible to reduce skin dose.
• The only exception is the microfocus magnification techniques used in mammography imaging.
Magnification
• Magnification is used in mammography to assess small lesions or microcalcifications.
• To magnify the breast, the OID is increased.
• Without modification, magnification will reduce the resolution of the image because of geometric unsharpness. To compensate for the loss of resolution when using magnification, a very small focal spot is utilized.
• Magnification factor = SID/SOD or image size/object size. The magnification factor or degree in which the image is magnified will vary. The standard is 1.5 times and 1.8 times but some manufacturers offer 2 magnification factors with one as high as 2.0 times.
• Magnification mammography should not use a grid. The scattered radiation is significantly reduced as a result of the large OID or air-gap and a grid will only contribute to increase radiation dose to the patient.
• Radiation dose to the patient is of concern because with magnification the radiation to the breast can double for just a single exposure because the breast is closer to the radiation source where the radiation intensity is greater.
• The greater the magnification factor the greater the skin dose to the patient.
Density Compensation Circuit
• The density compensation circuit allows the selection of at least 2 steps above and 2 steps below the normal setting. Each step translates to a 12%-15% increase or decrease in the mAs or a 0.15 change in the optical density.
Beam-Restricting Devices
• In general radiography the beam-restricting devices are cones, collimators, or diaphragms used to limit or collimate the size of the x-ray field to the area of interest. Collimating or decreasing field reduces scatter and therefore improves the contrast but decreasing the x-ray field will therefore require an increase in exposure to maintain constant density.
• Despite the benefits of collimation, no collimation is used in routine mammography imaging and the entire detector—not just the area of the breast—is therefore exposed.
• The main reason is to prevent extraneous light from compromising the perception of fine detail when the radiograph is viewed. Under MQSA standards the collimation should not extend beyond any edge of the image receptor by more than 1% of the SID.
Analog Mammography Cassettes
In analog imaging the cassette holds and stores the film during exposure. The newer, thinner cassettes are designed for both daylight and regular systems and have an identification system slot, capable of recording patient information on the radiograph.
• All cassettes are easy to open, durable, and have low absorption characteristics relative to the kVp.
• Mammography cassettes are designed for used with a single emulsion film and are therefore matched with a single intensifying screen.
Effects of Exposure Time, mA and mAs
• Low mAs reduce radiation dose to the patient in both analog and digital systems.
• Short exposure times minimize motion blur due to patient motion.
• In analog imaging the mAs will affect image density and patient dose.
• In digital imaging mAs selection will affect patient dose; however, the mAs has little effect on image brightness (the term brightness replaces density in digital imaging).
• Image acquisition and display are separated in digital imaging.
• In digital imaging the user can alter the image brightness after the exposure.
• In digital imaging the controlling factor of brightness includes not only the mA, but also the processing software and digital algorithm.
Effects of kVp in Analog and Digital Imaging
• The kVp controls the contrast in analog imaging. However, in digital imaging the image acquisition and display are separated.
• In digital imaging the user can alter the image contrast after the exposure.
• In digital imaging the controlling factor of contrast includes kV, processing software and digital algorithms.
• The wide latitude in digital allows visualization of the skin and penetration of dense breast tissue.
• The kVp selection on mammography generators can range from 20-40 kVp. However, the normal clinical practice use in the United States is 25-28 kVp.
Automatic Exposure Control in Analog Imaging
• Automatic exposure control (AEC) is used to control the length of the exposure and will therefore determine the density of the final image. The AEC allows the unit to respond to different breast compositions and various breast sizes.
• Units can have up to 10 AEC detector positions, which can be activated depending on the breast placement and size.
AEC Failure in Analog Imaging
• Commonly caused by activating the wrong detector relative to breast placement.
• If the activated detector is located under fatty breast tissue, the glandular tissue will be underexposed.
Exposure Control in Digital Imaging
• The surface that the breast rests on is the detector. The entire surface functions to monitor and control the exposure.
Back-Up Timer
• The back-up timer is used to prevent gross overexposure.
• The back-up timer for grid techniques is set at 600 mAs and for nongrid at 300 mAs.
• The back-up timer is reached during an exposure when the selected exposure is too low. This will occur if there are low-energy photons that cannot penetrate the breast because the breast is too dense or too large.
Avoiding Back-Up Timer During an Exposure
• Select a high kVp value, since the maximum mAs was reached and increasing the mAs is no longer an option.
• The back-up timer is also reached whenever imaging implants, using AEC. Implants should be imaged using manual techniques unless sufficient breast tissue covers the AEC cell or detector.
DIGITAL IMAGING
Bit
• A single binary digit of data such as “0” or “1.”
• The computer can only understand binary language which is “0” or “1”; however, the computer can use as many bits as necessary to express a decimal digit.
• A bunch of 8 bits is called a byte.
Bit Depth
• Number of gray shades that a pixel can produce. Represented by the formula 2n, where n = bit depth.
• Number of bits per pixel determines the bit depth or shades of gray demonstrated.
• The greater the bit depth the more accurate is the digital signal. It will have more shades of gray and therefore the image has greater detail.
• If the pixel has a bit depth of 8. Number of shades of gray that the pixel can produce = 28 or 256.
Matrix
• A digital image is a matrix of picture elements (pixels). Each cell corresponds to a specific location in the image. The matrix is therefore a box of cells with numeric values arranged in rows and columns.
• The size of the image matrix is determined by the number of pixels in the rows and columns. It is expressed by listing the number of pixels in each dimension (length and width).
• Common sizes: 256 × 256, 512 × 512, and 1024 × 1024
Pixel (Picture Element)
• One individual cell in a matrix
Recorded Detail or Resolution
• Controlled by the digital acquisition, i.e., pixel size and display matrix and the capabilities of the monitor.
• Spatial resolution of the imaging system is the minimum separation between 2 objects at which they can be distinguished as 2 separate objects in the image.
• The smaller the pixels, the greater the spatial resolution.
• The image resolution of the system is controlled by the number of pixels.
• The greater the number of pixels, the greater the image resolution.
Digital Printers
• Any printer used for full-field digital mammography (FFDM) must meet Food and Drug Administration (FDA) 510(k) clearance for digital mammography. Most digital printers offer daylight film loading features and use a photothermographic dry laser system.
• After exposure to an ultraprecise laser, the photosensitive film is then uniformly heated using a unique thermal element technology.
Preprocessing
• In digital imaging, preprocessing is automatic and occurs before the image is visualized at the acquisition workstation monitors (AWS). Preprocessing involves:
• Automatic rescaling to correct the raw data.
• Enhancing of contrast and image sharpness.
• Correction of artifacts due to bad detector elements or bad pixels.
• Application of processing algorithms to generate a histogram.
Postprocessing
• Image brightness is controlled mainly by the processing software and the algorithms that can be applied.
• Brightness can be altered after the exposure, independent of the mAs used to acquire the image.
• Image contrast is controlled by processing software and the algorithms that can be applied.
• Contrast can be altered after the exposure, independent of the kVp used to acquire the image.
• Windowing
• Changing the brightness and/or contrast.
• Window width controls the ratio of black to white displayed, therefore the contrast. Increased window width equals decreased contrast.
• Window level controls the brightness displayed. Increased window level equals increased brightness.
• Noise reduction or suppression removes digital signals that do not provide useful image data. Noise suppression tends to blur the image which reduces the visibility of fine details and can result in loss of contrast.
• Edge enhancement, frequency processing, or spatial frequency filtering is the averaging of the signal strength of 1 pixel with the strength of adjacent pixel or neighboring pixels. The more pixels involved in the averaging, the smoother the image appears.
• Smoothing is the averaging of each pixel’s frequency with surrounding pixels’ values to remove high-frequency noise. Smoothing reduces noise and contrast.
Film Digitizers
• Film digitizers are used to convert a hard copy x-ray film produced with an analog imaging system to a digital version that can be electronically transmitted, viewed, analyzed, and stored.
• In some of the film digitizers, a precalibrated light source is used to scan the image and a photodetector (PD) then measures the intensity of light passing through the film.
• Output from the PD is converted to a digital signal.
DETECTOR TECHNOLOGY IN DIGITAL MAMMOGRAPHY SYSTEMS
• Computed mammography (CM)
• CM adapts an analog imaging system to digital. It uses an imaging plate (IP) instead of a cassette.
• Mammography unit with IP plus a computer reader. The IP is physically removed from the unit and inserted into the computer reader.
• FFDM or DM uses a cassetteless system
• Built-in flat panel detector technology
• Photon-counting MicroDose
• A multislot scanning technology eliminates the scattered radiation.
COMPUTED MAMMOGRAPHY
Components of a Computed Mammography System
• IP or CM cassette which contain a photostimulable phosphor (PSP)
• Processing reader
• Computer
• Display monitor
The Imaging Plate
• A rigid cassette device with a radiolucent front, made of a hard plastic or similar radiolucent material to allow x-rays to enter and expose the PSP.
• Contains a PSP that is sometimes called a storage phosphor screen (SPS). The phosphor is the site of latent image formation.
Layers of the PSP
Protective Layer
• A thin tough layer of plastic that protects the phosphor layer from damage.
Phosphor Layer
• Common phosphor is europium-doped barium fluorohalide (BaFl: Eu). Europium (Eu) acts as an activator and is responsible for the storage properties of the PSP.
Conductive Layer
• This layer holds and traps excited electrons at higher energy levels.
• The conductive layer also grounds the plate to reduce static electricity problems and absorbs light to increase sharpness.
Support Layer
• Semirigid material provides the imaging sheet with strength and is a base for the other layers.
Light Shield Layer
• Prevents light from erasing the data on the image recorder or striking through the backing layer.
Backing Layer
• A soft polymer that protects the back of the IP.
Phosphor Characteristics
• Turbid phosphors have crystals distributed throughout the phosphor level. They produce lateral spreading of light which reduces the spatial resolution of the image. For example, barium fluorobromide and europium, barium fluorobromide iodide with europium, barium fluoroiodide with europium, barium strontium-fluoride bromide-iodide, gadolinium oxysulfide, and rubidium chloride.
• Needle phosphors have crystals growing in columns. They have less lateral spread of light and better spatial resolution. For example, cesium iodide.
Process of PSP Image Capture
• When x-rays strike the atoms of barium fluorobromide the outer shell electrons become excited (due to Compton and photoelectric x-ray interactions) and gain energy. These excited electrons are called metastable electrons.
• The metastable electrons in the crystals move from their normal orbital location to conductive layer. The electrons are then move to the F-Trap where they are held. This process represents the latent image formation.
• The number of electrons captured in the F-Trap is directly proportional to the amount of energy absorbed by the PSP.
• The latent image can last for several hours but loses 25% of its energy in 8 hours. Fading is the term used to describe the time it takes for the latent image to disappear.
• Some but not all electrons raised to a higher energy level will spontaneously return to their resting state.
The Computer Processing or PSP Reader
• The computer reader device opens the IP and removes the PSP. It scans, reads, and erases the exposed PSP.
• The entire process can take up to 60 seconds and depends on the manufacturer.
• After scanning, the PSP is returned to the IP and the IP is ejected from the computer reader.
Image Extraction Process
• The IP is taken to a computer processing reader and the PSP is extracted.
• The conductive layer of the PSP has the excited electrons trapped at higher energy levels.
• A finely focused beam of infrared light scans the PSP in a transverse raster pattern.
• The light provides energy to the electrons trapped in the conductive layer. This causes electrons to release the energy that they absorbed from the x-ray beam and to return to their lower energy resting state.
• The energy released from the metastable is in the form of a bluish-purple light.
• The bluish-purple light released by the metastable electrons is collected by light guides of a photomultiplier tube (PMT), photodetector (PD), or charge-coupled device (CCD) and used to record the image.
• Photostimulable luminescence (PSL) is the emission of the bluish-purple light from electrons as they transition from higher energy to a lower energy state.
• The amount of light (photostimulable luminescence or PSL) emitted by the PSP during the reading process, related to the level of exposure received by the receptor, is termed the speed class. The technical factors (exposure received by the PSP) will determine the speed class of the system.
• The PSP must undergo a final erasure by a high-intensity light source to ensure that all the electrons are back at their ground state.
• The laser light is red while the PSP emits a bluish-purple light. This allows the PD to differentiate between the light used for PSP scanning versus the light emitted for image formation.
Types of Computer Reader–Processing Technology
• Point-scan reader (flying-spot scanner) is an older method of reading the PSP. The laser beam scans the entire PSP.
• Line-scan reader have several lasers in a row. The PSP is pulled under the linear scanner or the PSP remains stationary and the scanner moves across the plate. This method is capable of processing an entire line of the PSP at one time so is faster than the point-scan readers.
• Dual-sided readers (dual-head flying-spot scanner) scan both sides of the PSP which allows the collection of more image data with less image noise.
The Photomultiplier Tube, Photodetector, or Charge-Coupled Device
• The PMT collects the bluish-purple light given off by the trapped electrons as they return to their normal neutral state.
• The light is transmitted to an analog-to-digital converter (ADC).
• The ADC converts the analog signal to into a digital signal (the binary language or numeric data) that can be read by a computer.
• The digital signal is sent to a computer for processing.
• After processing, the digital signal (binary data) must be converted back to an analog signal (various shades of gray) for viewing at the display monitor.
Function of the High-Intensity Light Source
• The high-intensity light erases the plate by releasing any remaining electron energy.
• Some excited electrons will always remain in the conductive layer and if they are not removed they will result in ghosting on subsequent IPs.
• Any residual latent image is removed by flooding the phosphor with very intense light. This allows the PSP to be reused many times.
• IPs must be erased every 48 hours to remove background radiation even if not used.
• The IPs must be erased before using if the last time of erasure is unknown.
Function of the Analog-to-Digital Converter
• The ADC converts analog (light or electronic) signals from the PMT/detector to a digital signal consisting of binary or numerical data.
• Binary language is the only language that the computer will understand therefore any electronic or light signals must be converted to binary language. The computer then manipulates, processes, and stores the data.
Function of the Computer in the CM Digital System
• The computer receives a digital signal from the PMT/detector; applies selected algorithms to the data received, processes the data, and sends the digital data to a digital-to-analog converter (DAC).
• The DAC converts the binary computer language back to an electronic signal (various shades of grays) to be sent to the display monitor.
Function of the Digital-to-Analog Converter
• The DAC converts digitally manipulated computer data to an analog (electronic) signal.
• The image will appear on the display monitor as various shades of gray. However, the computer outputs a signal in computer language. This digital signal must be converted to an analog signal for display.
Advantages of CM
• Wide latitude and dynamic range.
• CM is faster than analog imaging systems.
• Reduced repeat due to exposure errors.
• Reduced risk of lost films.
• Allows a more efficient and faster workflow.
Disadvantages of CM
• Lower spatial resolution (digital can manipulate the pixel value after the exposure which compensates for the lower spatial resolution).
• IPs can be easily damaged during transport.
• IPs are susceptible to scratches in computer reader.
• Noise images at low exposures.
• Increased image noise due to the sensitivity of the PSP to radiation.
• “Exposure creep” due to lack of awareness of radiation safety and patient dose.
FULL-FIELD DIGITAL MAMMOGRAPHY
There are a number of DM systems available including indirect capture flat-panel detector and direct capture flat-panel detector.
Flat-Panel Detector Systems
• Nonscintillator based. Direct: 2-step process.
• The x-ray beam strikes a photoconductor, e.g., amorphous selenium (a-Se).
• a-Se converts x-ray to electrons.
• A high-voltage charge is applied to the a-Se after the x-ray exposure.
• Electrons are released from the amorphous selenium as a result of the exposure.
• Electrons migrate to the detector element (DEL) on a thin-film transistor (TFT).
• The electron signal is sent to an ADC then to a computer for analysis.
• Information from the computer is displayed on LCD monitor.
• Scintillator based. Indirect: 3-step process.
• The x-ray beam strikes a scintillator, e.g., cesium iodide.
• Cesium iodide converts x-ray to light.
• Light strikes the photoconductive material, e.g., amorphous silicon (a-Si), i.e., photomultiplier, photodiode, or thin-film diodes (TFD).
• TFD converts light to electrons.
• Electrons migrate to the DEL on a TFT.
• The electron signal is sent to an ADC then to a computer for analysis.
• Information from the computer is displayed on LCD monitor.
Thin-Film Transistor
• The TFT is a device made of millions of detector elements, electrodes, photodiodes, storage capacitors, and other components that is used to accept and store electrons and to generate an electric charge from each stored electron.
• In the direct detection array, the components of the TFT are arranged on a-Se as the semiconductor material.
• In the indirect detection array the components of the TFT uses a-Si.
• A variation of the TFTs are used in high-quality flat-panel liquid-crystal displays (LCDs).
Detector Element (DEL or dexel)
• The detector elements (DELs or dexels) are located within rows and columns on the TFT and are part of the complex circuit device of the TFT.
• DEL is the sensitive component of the TFT that collects electrons emitted from either a-Se or a-Si, to represent individual components of a digital image.
• After the exposure, the DELs read those electrons in a sequential pattern that matches their location within the detector matrix.
• Once the DELs are read, the flat-panel detector automatically erases and is ready for another exposure.
• DEL size controls the recorded detail, or spatial resolution, for the flat-panel device.
• DEL size contributes to the image blur present in a flat-panel detector receptor.
• The larger the DELs in a flat-panel detector, the more image blur.
• DEL size is set by the manufacturer and is not under the technologist’s control.
Fill Factor
• Each square in the TFT matrix has sensitive and nonsensitive areas. The sensitive area represents the DELs that collect the electron charge.
• The fill factor is the ratio of the sensitive area to the entire detector area and is usually expressed as a percentage.
• Fill factor will affect the spatial resolution and signal-to-noise (SNR) ratio. A typical fill factor is 80%.
Advantages of DM
• No plates to drop or damage.
• No plates to be transferred.
• Has increased DQE over CM systems (detective quantum efficiency or DQE describes how efficient the system is in converting x-ray input signals into a useful output image).
Disadvantage of DM
• Image lag or memory effect because the charge is trapped in the metastable band and is released slowly over time. Image lag time varies and is shorter for flat-panel digital detectors based on indirect conversion.
Photon-Counting Image Capture
• Photon-counting MicroDose mammography system detects and captures individual x-ray photons leaving the breast using a crystalline silicon detector and photon-counting electronics, therefore eliminating the signal loss which occurs in digital systems when analog-to-digital conversions are used.
• The system uses tungsten anode and aluminum as the added filter.
• The system uses a mulislot system that virtually eliminating scatter, therefore a grid is not necessary, allowing a further reduction in patient dose. Images are acquired with a resolution of 25 megapixels.
Advantages of the Photon-Counting System
• Ergonomic design
• Warm detector
• Lower radiation dose
• 40% less than FFDM. Average glandular dose ranges from 0.4-0.8 mGy on the CC and 0.4-0.87 mGy on the MLO.
• Robust and temperature tolerant.
• 10-50°C (50-122°F)
• No ADC needed—therefore no lost signals.
• No ghost image—100% fill factor.
Disadvantages of the Photon-Counting System
• Higher recall rates
CONTINUOUS QUALITY IMPROVEMENT
Quality Assurance or Continuous Quality Improvement
• Quality assurance includes the total overall management of actions taken to consistently provide high image quality in the radiology department with the primary objective being to enhance patient care.
• Quality assurance is very important in mammography, both in meeting the MQSA standard and equally important in ensuring quality patient care. Every quality assurance program must include a quality control (QC) component which includes the collection and evaluation of data with a systematic and structured mechanism to control variables such as repeat radiographs, image quality, processing variations, patient technical factors, technical effectiveness, efficiency, and in-service education.
Mammography Quality Standard Act
• In mammography, concern about the poor quality mammograms available at some facilities in the United States led to the passage of the MQSA.
• It was enacted on October 27, 1992 after which the US Congress authorized the FDA to develop and implement the MQSA regulations. Interim regulation passed in Dec 1993, and became effective Feb 1994. In 1995 enforcement began.
Areas Subject to MQSA Inspection and Testing
• Policy and procedures manuals
• Mammography equipment testing
• Personnel records
• Initial training requirements and continuing education (CE) requirements for radiologic technologist, physicist, and radiologist
• Medical records
• Patient records and medical outcome audit
Advantages of MQSA
• Reduction of unnecessary radiation to patients by reducing repeats
• Improvement in overall efficiency of service
• Improved patient satisfaction
• Consistency of image production
• Cost-effectiveness
Analog QC Test and Frequencies (Performed by the Mammographer or Technologist)
• Daily tasks
• Darkroom cleanliness
• Processor quality control
• Weekly tasks
• View box and viewing conditions
• Phantom images
• Screen cleaning
• Monthly tasks
• Visual checklist
• Quarterly tasks
• Repeat analysis
• Analysis of fixer retention
• Semiannual tasks
• Darkroom fog testing
• Screen-film contact
• Compression
Digital QC
• Specific digital quality control tests are determined by the manufacturer. They vary and can include varying schedules of daily, weekly, monthly, quarterly, and semiannually quality control duties
Common Digital QC Test and Test Frequencies (Performed by the Mammographer or Technologist)
• Daily
• Monitor cleaning, overhead lights, and viewing conditions
• Weekly or Monthly
• Flat field test is a check of the detector.
• SMPTE is a test pattern that can be used to check the communication between detector to the printer or the detector to the monitor.
• Phantom image is a check of the detector and communication between the detector and the AWS.
• CNR (contrast-to-noise ratio) measures the ability of the digital system to demonstrate objects of different brightness separate from their surroundings. CNR compares the image contrast to the level of background noise. Noise measures how much target is obscured by random signal variations.
• MTF (modulation transfer function) measurement is the ability of the detector system to transfer its spatial resolution characteristics to the image. The system should record the available spatial frequencies and produce an image exactly as the object. The ratio of image to object is expressed as a function of the spatial frequency.
• SNR (signal-to-noise ratio) compares the level of a desired signal to the level of background noise. It is defined as the ratio of signal power to the noise power. A ratio higher than 1:1 indicates more signal than noise. The signal represents the difference between x-rays transmitted to the detector and those absorbed. Sources of image noise can include scattered radiation, background radiation, or insufficient technical factors.
• Dynamic range is the receptor’s ability to respond to different exposure levels. Quantum mottle will appear if the exposure is 50% below the ideal exposure. If the exposure is 200% higher than the idea, there is reduced contrast.
• Automatic optimization of parameters (AOP) check to ensure dose reduction.
• Monthly
• Visual checklist surveys the room for cracks, missing lights or faulty equipment.
• Quarterly
• Repeat analysis checks the repeat and reject rates.
• Semiannually
• Compression checks the minimum and maximum compression force for automatic modes.
Quality Control Duties of the Radiologist
• The level of commitment by the radiologist is often reflected in the quality of a mammography program. General responsibilities include:
• Signing and checking the medical auditing.
• Patient communication.
• Overseeing the activities of the mammographer and the medical physicist.
Quality Control Duties of the Medical Physicist
• The medical physicist is a good potential source to consult prior to contacting the equipment service personnel and before any inspection. Recommendations and reports from the physicist should be reviewed with the radiologist and mammographer incharge of quality control. At a MQSA inspection, the medical physicist’s report is always checked. Most equipment failures allow a 30-day period to provide corrections. The exceptions are for excessive breast dose and phantom image quality, which must be immediately corrected. General responsibilities include:
• Evaluations of the mammographic unit
• Yearly reviews of all test procedures including records, charts, and mammography policies and procedures
• Written report and any corrective recommendation on all equipment testing
COMMON MQSA REQUIREMENTS
Personnel Requirements for Radiologist, Technologist, and Physicists
• State license required for some states
• Mammography education and initial training
• Performing regular mammograms, interpretations, and inspections for the mammographer (technologist), radiologist, and physicists, respectively
• Annual CE requirements
FDA Certificate Placement
• The certificate must be prominently displayed where all patients can see.
Consumer Complaints Mechanism
• The facility must have a written policy system in place to collect and resolve all patients’ complaints. Serious consumer complaints reports must be forwarded to the FDA or State certifying agency. The facility must have instructions on how to proceed with serious unresolved complaints and must maintain a record of each complaint for 3 years.
Infection Control
• Evidence of infection control, e.g., wipes, sink with soap, or hand sanitizer should be present.
Self-referrals
• A patient who comes for a mammogram but has no health-care provider is considered a self-referral. A facility can decide to accept or not to accept self-referrals. Facilities accepting self-referral must have a policy in place.
Medical Records
• Medical records must be kept for not less than 5 years in digital or hard copy format. If the patient has not had additional mammograms at the facility, records must be kept for not less than 10 years. Some state or local laws may require longer times. Originals, in hard copy or digital format, must be transferred at the request of the patient. Transferred records must be of interpretation quality.
• Medical records must contain the name of patient plus an additional patient identifier, e.g., medical record number, the date of examination, the name of radiologist interpreting the mammogram, and a final assessment of findings.
Assessment Categories
• These are categories recognized by all physicians and MQSA. They were designed to standardize breast interpretation.
BIRAD 0: Need additional imaging information and/or prior mammograms for comparison.
BIRAD 1: Negative
BIRAD 2: Benign finding
BIRAD 3: Probably benign finding—short-interval follow-up suggested
BIRAD 4: Suspicious abnormality—biopsy should be considered.
BIRAD 5: Highly suggestive of malignancy—appropriate action should be taken.
BIRAD 6: Known biopsy proven malignancy—appropriate action should be taken.
Communication of Results
• Results must be sent to the patient’s physician and if it is a concerned finding, it should be sent within 3 days. A written result in lay terms must also be sent to the patient as soon as possible for concerned findings and within 30 days of the mammogram for normal findings. Most inspectors interpret a concerned finding to be BIRAD 0, 3, 4, 5, and 6.
Medical Audit
• The medical audit is used to follow positive interpretation and to correlate them with pathology results. At a minimum the medical audit must track BIRAD 4 and 5 and must be reviewed annually by the interpreting physician.
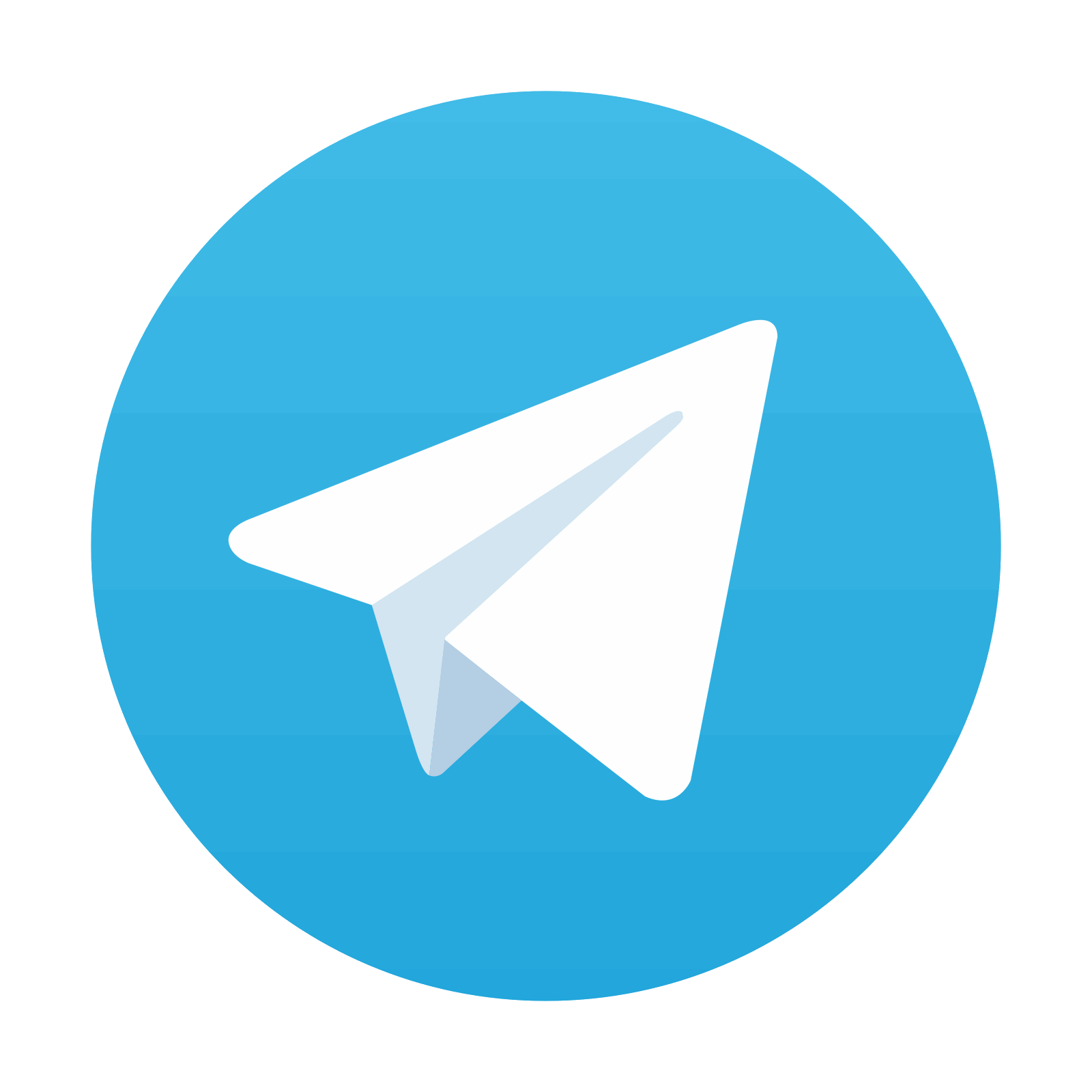
Stay updated, free articles. Join our Telegram channel

Full access? Get Clinical Tree
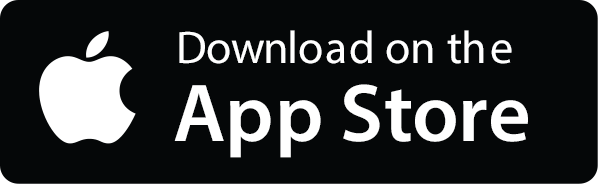
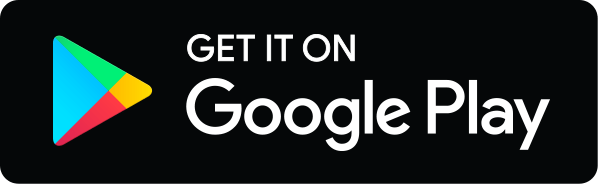