Integrated SPECT-CT and PET-CT in Cardiac Imaging
Oliver Gaemperli
Tiziano Schepis
Gustav K. von Schulthess
Philipp A. Kaufmann
In cardiac imaging, integration of computed tomography (CT) with single-photon emission computed tomography (SPECT) or positron emission tomography (PET) is importantly different from the situation in body imaging for tumors and inflammation. Anatomic information on cardiac soft-tissue structures is less relevant in integrated cardiac imaging. However, transmission correction in cardiac imaging is now widely used to avoid misinterpretations of findings in the inferoposterior wall. Transmission correction with CT is readily accomplished for SPECT as well as PET imaging with the use of integrated SPECT-CT and PET-CT systems.
With the newest generation multi-row detector CT scanners in combination with PET, a combined evaluation of coronary anatomy, calcium score, myocardial perfusion, and viability will potentially provide a comprehensive “one-stop shopping” examination for patients at risk for or with coronary artery disease (see Fig. 32.1). This has long been a so far unsuccessful quest of magnetic resonance imaging (MRI) because of inadequate coronary artery MR angiography. On the other hand, multi-slice CT is now being integrated in the daily clinical routine, providing consistent diagnostic data for noninvasive coronary angiography, particularly with the latest developments of 64-slice scanners. This may have major consequences for the diagnostic algorithm for screening and evaluation of coronary artery disease in the near future. Further developments, such as dual-beam CT, that have been announced (see Chapter 6) may accelerate the implementation of this technique as a first-line tool in cardiology.
Introduction
Integrating computed tomography (CT) with single-photon emission computed tomography (SPECT) or positron emission tomography (PET) has different aims in cardiac imaging than in body imaging. In body PET-CT, CT is used to localize the tracer accumulation seen on PET and for attenuation correction. Cardiac coronary anatomy is simpler but more difficult to image with CT, because the speed and spatial resolution requirements are harder to meet. With the new developments in CT technology, coronary anatomy imaging is on the verge of becoming a reality. The most important application of any cardiac imaging procedure is to evaluate coronary artery disease. Noninvasive approaches exist for assessing myocardial ischemia (see Chapters 29 and 30), which is currently done by using myocardial stress perfusion imaging, mainly with SPECT and PET, or by imaging wall-motion abnormalities due to ischemia, mainly with stress echocardiography and magnetic resonance imaging (MRI). The diagnostic gold standard for establishing the presence of coronary artery disease is still x-ray coronary angiography, with all its drawbacks. A major limitation of this technique is its invasive nature, with high procedure-related morbidity (1.5%) and mortality (0.15%).
In addition, the physiologic significance of any lesion is frequently difficult to assess from angiographic information alone, and perfusion abnormalities may occur in the absence of arteriographically assessable stenoses. Therefore, patients often are referred to perfusion imaging to determine the clinical relevance of a coronary stenosis after its invasive documentation. Thus, the integration of noninvasive assessment of coronary anatomy by multi-slice CT coronary angiography and of myocardial perfusion or viability by PET or SPECT is potentially useful.
In addition, the physiologic significance of any lesion is frequently difficult to assess from angiographic information alone, and perfusion abnormalities may occur in the absence of arteriographically assessable stenoses. Therefore, patients often are referred to perfusion imaging to determine the clinical relevance of a coronary stenosis after its invasive documentation. Thus, the integration of noninvasive assessment of coronary anatomy by multi-slice CT coronary angiography and of myocardial perfusion or viability by PET or SPECT is potentially useful.
Attenuation Correction with CT
The sensitivity and specificity of cardiac SPECT are commonly affected by image artifacts caused by photon attenuation. “Characteristic patterns” of attenuation in female and male patients are rendered unpredictable by the nonuniform attenuation characteristics of the human body, particularly in the chest area, and by the widely variable body habitus of individual patients. Breast and diaphragmatic attenuation are among the most common causes of these artifacts, but lateral wall artifacts also occur because of obesity. One strategy for identifying and correcting attenuation artifacts is to introduce electrocardiogram (ECG)-gated SPECT. Fixed defects in the anterior and inferior walls may be distinguished from infarction by the identification of normal wall motion. However, in 85 women, Taillefer et al. (1) were able to reclassify only five false-positive fixed defects as normal because of the presence of wall-motion information, and Lee et al. (2) found no gains with gating in 68 patients. By contrast, we found in 153 consecutive cases that by reclassifying the condition of patients with fixed defects and normal function as normal, patients with unexplained fixed defects (no clinical myocardial infarction) decreased from 29% to 10% (3). This confirms that gating adds considerable value to SPECT myocardial perfusion imaging in characterizing fixed defects and potentially improves test specificity.
Whereas the accuracy of cardiac PET imaging has long benefited from correction methods for tissue attenuation, in SPECT imaging, commercial methods have only recently been made available. Various techniques with different line sources, such as americium 241 (241Am), gadolinium 153 (153Gd), and technetium 99m (99mTc), have been proposed. Initial reports indicate that some of these methods have achieved significant improvements but that others have created more artifacts than they have remedied. An overview of these methods, which have varied greatly in their clinical success, has been given elsewhere (4).
Certainly the use of CT for attenuation correction could well be an important step forward in solving some of the major attenuation correction problems of the SPECT technique, allowing consistent image reading in male and female patients. Because of the recent introduction of this technique, only limited data are available. Although initial experiences seem promising (5,6), there are some drawbacks that have to be overcome. For example, misalignment in the y-direction between SPECT and the attenuation map can lead to artifacts in the apical, septal, and anterior walls that will appear as defects. It also can cause overcorrection in the basal inferior and lateral segments. There is evidence that mismatches along the other directions may have a similar effect. The coregistration of SPECT and the attenuation map needs to be verified for every patient, even when using integrated dual-modality imaging devices (7).
In PET the major advantage of using CT for attenuation correction is the short time duration (less than 5 seconds) compared with conventional correction with germanium sources (10 to 20 minutes). This allows the separation of the acquisitions of perfusion and viability PET scans, as the time loss for an additional transmission scan is minimal. Logistically, this is a major issue in daily clinical routine, as otherwise the ammonia scan must precede the fluorodeoxyglucose (FDG) scan, a protocol sequence with severe limitations:
After the production of FDG, the slots for patients scheduled for an FDG scan are very tight, and any delay caused by potential problems during the nitrogen 13 [13N]NH3 protocol (e.g., unsuccessful [13N]NH3 production or intravenous delivery, acquisition delay, chest pain, and adenosine side effects) has an unfavorable effect on patient scheduling for the whole day.
At the end of the [13N]NH3 protocol, the patient receives a fluorine 18 (18F)-FDG injection and remains in the scanner for uptake for up to 40 minutes before imaging can be started. This dead time can be avoided by performing the scans on different days or at least separated by time, allowing 18F-FDG decay and putting the [13N]NH3 scans at the end of the protocol, which is favorable from the point of view of cyclotron logistics.
We recently analyzed the feasibility and reproducibility of CT attenuation correction for quantitative PET myocardial perfusion measurements. The results show that myocardial blood flow quantification is independent of CT beam intensity and that ECG gating of the CT beam seems not to be necessary (8). Therefore, an ungated low-dose CT scan with 5 mAs, the lowest possible dose available on our CT and corresponding to approximately 0.4 mSv radiation exposure, was used to document the reproducibility of the measurements. Attenuation correction with CT provided results highly comparable to those obtained by using germanium attenuation correction, as long as the same reconstruction algorithm (filtered back-projection vs. iterative reconstruction) was applied.
The benefit of CT attenuation correction for time-saving issues will be particularly important once the use of oxygen 15 [15O]H2O is ripe for clinical use in myocardial blood flow (MBF) assessment (see Chapter 29). The relatively short half-life of this tracer (2 minutes) allows repeated measurements within 10 minutes and flow reserve measurements with a total scan duration of approximately 20 minutes (9). Cutting down the additional 20 minutes for attenuation correction to approximately 4 to 5 seconds for CT correction will considerably shorten the protocol and increase patient throughput.
Cardiac “One-Stop Shopping” with PET-CT
Noninvasive assessment of coronary artery disease is an important task, because more than 1 million invasive diagnostic coronary angiography procedures are performed each year in the United States alone. Coronary angiography is at present the only accepted method for the clinical imaging of coronary artery disease, despite its cost, the inconvenience to patients, and the inherent risk of complications. These limitations have prompted an intensive search for alternative, noninvasive techniques for coronary artery visualization. The major obstacles that must be overcome are the small diameter of the coronary arteries, their tortuous course, their close anatomic relation to the coronary veins, and their continuous motion due to cardiac contractions and respiratory excursions.
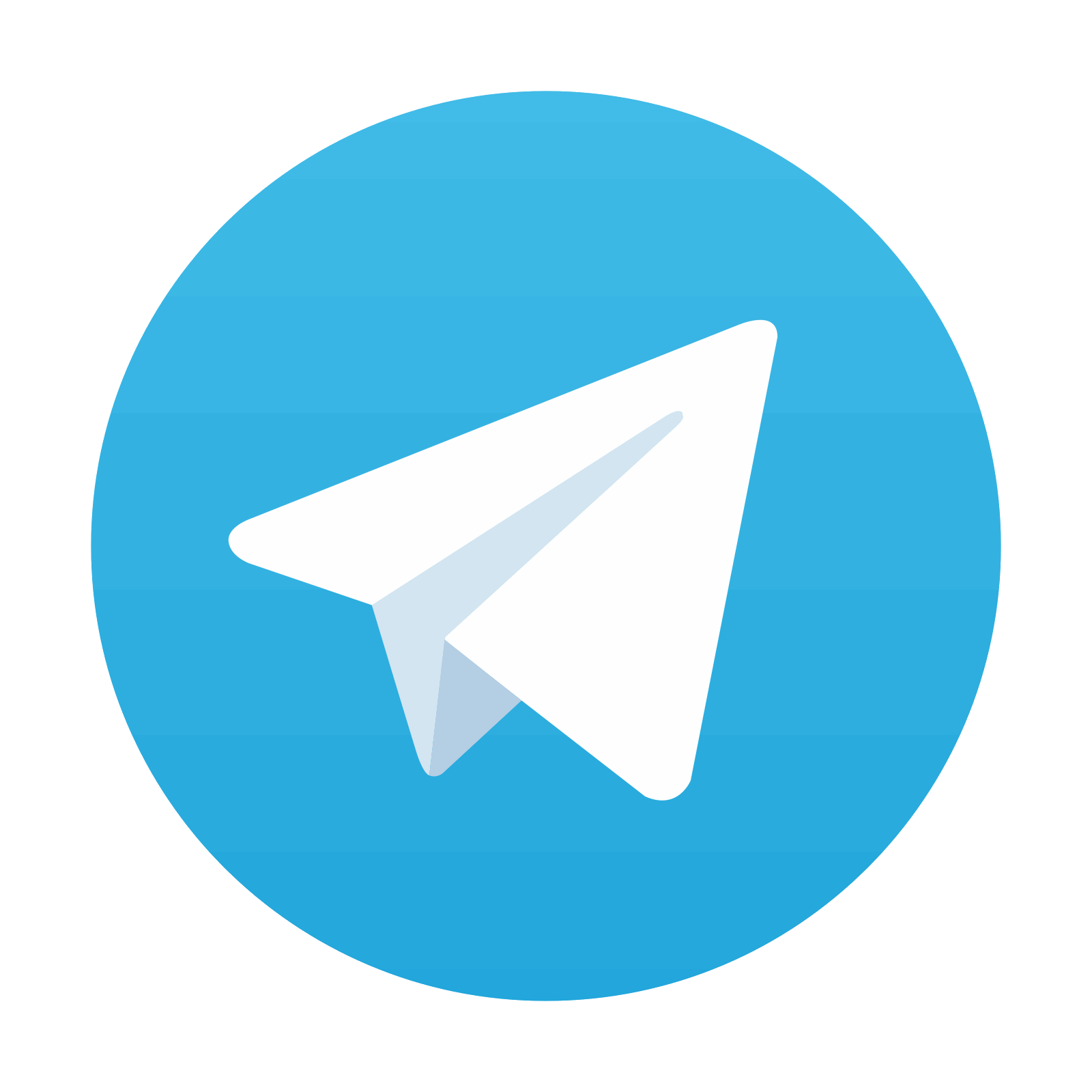
Stay updated, free articles. Join our Telegram channel

Full access? Get Clinical Tree
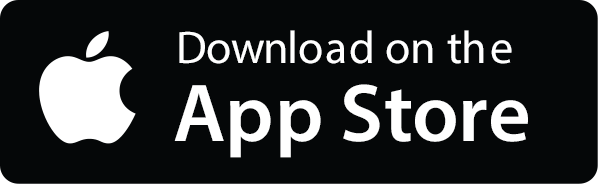
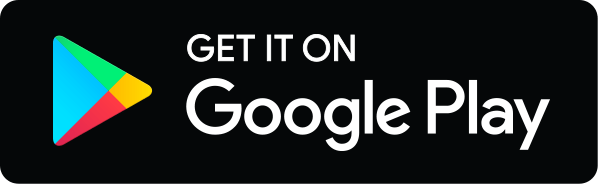