Liver Biopsy
Percutaneous Liver Biopsy
Random
Brief Rationale.
The first percutaneous liver aspiration was performed by German physician Paul Ehrlich in 1883, but the technique became widely accepted only after the landmark publication by Menghini in 1958. It is likely that the intensive efforts to characterize and to quantify diffuse liver disease through magnetic resonance (MR) elastography, MR spectroscopy, and ultrasound elastography will at some point obviate the need for liver biopsy in many patients. However, liver biopsy currently remains the “gold standard” for parenchymal evaluation in the setting of diffuse hepatic disease. Current indications for nonfocal liver biopsy include the diagnosis and staging of cirrhosis, cholestatic liver disease, metabolic storage disease, and other infiltrative diseases.
Preprocedural Evaluation and Contraindications.
Patients should have nothing by mouth for 4 to 6 hours before a liver biopsy if intravenous sedatives are to be administered. Although there is wide variation in individual practices, the Society of Interventional Radiology has provided a consensus set of guidelines for periprocedural management of laboratory coagulation parameters and medications that recommends checking preprocedural international normalized ratio (INR) in all patients and activated partial thromboplastin time (aPTT) in patients receiving unfractionated heparin with subsequent correction to INR below 1.5 and aPTT of less than 1.5 times the control value. Although these guidelines do not recommend checking platelets before the procedure, they do recommend correcting platelets to more than 50,000 in patients with known thrombocytopenia. Warfarin as well as antiplatelet agents such as clopidogrel should be discontinued for at least 5 days before biopsy. Although there is no consensus, many practices restart antiplatelet therapy such as clopidogrel 48 to 72 hours and warfarin 24 hours after the biopsy. There also is no consensus addressing the alteration of heparin therapy, although others have suggested holding heparin for between 2 and 6 hours before the procedure and restarting heparin 12 hours after the procedure.
Although no absolute contraindications to liver biopsy exist, relative contraindications, such as the patient’s cooperation, extrahepatic biliary obstruction, and severe coagulopathy, may warrant consideration of transjugular liver biopsy in specific cases. However, correction of coagulation abnormalities can also be performed to proceed safely with percutaneous liver biopsy.
Guidance and Technique.
Percutaneous liver biopsy is a safe procedure that can be performed on an outpatient basis. Imaging guidance is becoming increasingly prevalent and has multiple advantages over palpation- or percussion-guided techniques, including a decreased complication rate and superior diagnostic yield. Ultrasound is the preferred imaging modality in most cases because of low cost, real-time guidance, multiplanar imaging, portability, visualization and avoidance of major blood vessels and lung, and lack of ionizing radiation. Imaging guidance with ultrasound has also been shown to decrease the number of major and minor complications. Although ultrasound-guided liver biopsies are slightly more costly on a per-procedure basis than biopsies guided by palpation, cost-effectiveness analyses have suggested that the other benefits of ultrasound guidance may reduce the overall cost of liver biopsy.
Ultrasound-guided biopsies can be performed through a subcostal or intercostal approach. A subcostal approach is generally favored over an intercostal puncture because of a lower risk of pneumothorax or intercostal artery injury. Sonographically guided interventions can be performed either by a freehand technique (which provides for greater freedom in needle placement) or with an attached biopsy guide (which provides greater accuracy). Local anesthetic should be liberally applied from the skin entry site down through the subcutaneous fat and peritoneum directly onto the liver capsule. If possible, the biopsy needle should be placed during a breath hold to reduce the risk of capsule laceration and to facilitate biopsy at the site of local anesthetic administration. Although the American Association for the Study of Liver Diseases (AASLD) recommends a 16-gauge biopsy needle of 2 or 3 cm in length for the diagnosis, grading, and staging of diffuse parenchymal liver diseases, the use of 18-gauge cutting needles is common in many institutions. The patients should be monitored for 2 to 4 hours after the procedure in a recovery unit before discharge ( Fig. 84-1 ).
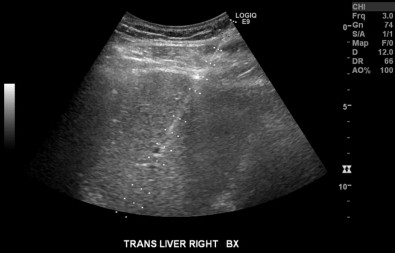
Complications.
The most common complication after liver biopsy is pain, the exact cause of which is unknown but may be due to a small bile leak or subcapsular hematoma. Most postbiopsy pain is readily managed with intravenous or oral analgesics. Bleeding is the most important complication after liver biopsy. Subclinical bleeding not requiring specific intervention occurs in up to 23% of liver biopsies, and severe hemorrhage requiring transfusion or other intervention occurs in 0.35% to 0.5%. Other potential complications include damage to adjacent organs, pneumothorax, hemothorax, peritonitis, and death. The reported mortality rate after liver biopsy is approximately 0.01%.
Image-Guided Percutaneous Liver Mass Biopsy
Indications
Hepatocellular Carcinoma.
The recommendations for hepatocellular cancer screening have recently been updated by the AASLD, and surveillance for patients at high risk for the development of hepatocellular carcinoma (HCC) is recommended with ultrasound every 6 months. Routine serum α-fetoprotein is no longer recommended for screening or diagnosis because of lack of sensitivity and specificity. If a nodule larger than 1 cm is identified on screening ultrasound, contrast-enhanced multiphasic computed tomography (CT) or magnetic resonance imaging (MRI) is performed. Liver biopsy is rarely indicated in the evaluation for HCC, particularly in the setting of cirrhosis, because of the ability to diagnose most cases of HCC with imaging alone. In addition, the small but real risk of bleeding and tumor seeding generally outweighs the benefit of histologic diagnosis, given the extremely high pretest probability of HCC with imaging. The risk of tumor seeding along a biopsy track is estimated at 0.9% per patient per year. At most institutions, HCC is predominantly an imaging diagnosis, and patients are managed without histologic confirmation. There are currently two sets of imaging criteria for the diagnosis of HCC on CT or MRI, the Liver Imaging Reporting and Data System (LI-RADS) and the United Network for Organ Sharing (UNOS)/Organ Procurement and Transplant Network (OPTN) criteria. Both sets of criteria incorporate the presence or absence of arterial enhancement, washout, capsule, and growth to diagnose HCC. Percutaneous image-guided liver biopsy is reserved for lesions that do not meet strict imaging characteristics by MRI and CT. However, even biopsy is not 100% accurate for the diagnosis of small HCCs.
Other Focal Liver Lesions.
Targeted liver biopsies are frequently performed in the evaluation of focal hepatic abnormalities not suspected of being HCC. CT and MRI are often used for first-line characterization of focal hepatic abnormalities because of the ability to definitively diagnose many HCCs, hemangiomas, adenomas, and focal hepatic steatosis. Other lesions have a less characteristic imaging appearance and typically require biopsy for tissue diagnosis. As metastatic lesions are more common than primary hepatic malignant disease, the majority of targeted hepatic biopsies are performed in an effort to evaluate focal liver lesions in the setting of a known primary malignant neoplasm. Sampling the metastatic lesions in the liver (or elsewhere) can serve both to make a diagnosis and to stage the disease concurrently. Thus, liver biopsy is often performed, even when the site of extrahepatic primary malignant disease is suspected or known ( Fig. 84-2 ).
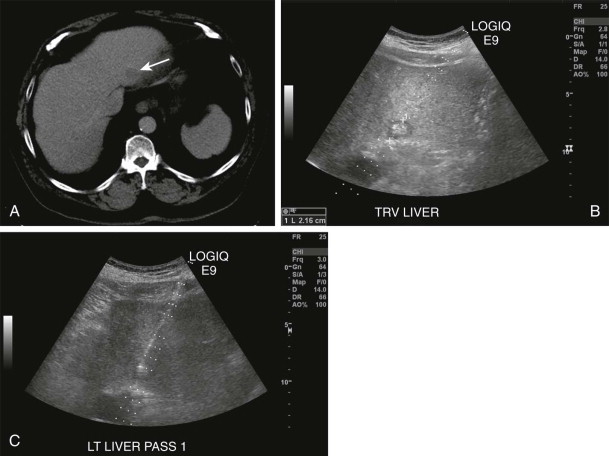
Preprocedural Evaluation.
The preprocedural evaluation for patients undergoing percutaneous liver biopsy for a focal mass lesion in the liver is similar to that described for image-guided random liver biopsy.
Guidance and Technique.
Most image-guided liver biopsies are performed under either ultrasound or CT guidance. Ultrasound is the preferred imaging modality worldwide for liver biopsy guidance for the reasons described in the preceding section. However, CT guidance has been proved to be effective and safe for experienced operators. The advantages of ultrasound include less expense, lack of radiation, and real-time imaging as well as ability to access lesions at the hepatic dome. The disadvantages of ultrasound include user dependence and difficulty of imaging large patients. The lack of inherent tissue contrast often makes identification of targets difficult by noncontrast CT. Both core needle and fine-needle aspiration techniques can be used for liver biopsy, but most studies favor obtaining a core needle specimen because of increased specificity and accuracy in diagnosis and subtyping of tumors, superiority in diagnosis of benign lesions, decreased biologic sampling errors, and preservation of tissue architecture. In biopsy of a focal liver lesion, every attempt should be made to perform the biopsy through a cuff of normal liver parenchyma to decrease the risk of bleeding. With larger masses, core samples should be taken from the periphery of the lesion to avoid central necrosis.
Results and Complications.
Image-guided biopsy of focal liver lesions has been shown to have a high diagnostic accuracy (94.5%-100%), even for lesions between 0.5 and 1.0 cm. In patients with a known primary malignant neoplasm with a focal liver lesion, a second malignant neoplasm was diagnosed in 5% of cases, and a benign entity was identified in another 3.4%. The complications associated with liver biopsy of a focal liver lesion are similar to those for random liver biopsy. The risk of tumor seeding after biopsy of HCC is high (0.76%-2.7%) and is a catastrophic complication, potentially causing removal from the transplant list ( Fig. 84-3 ).
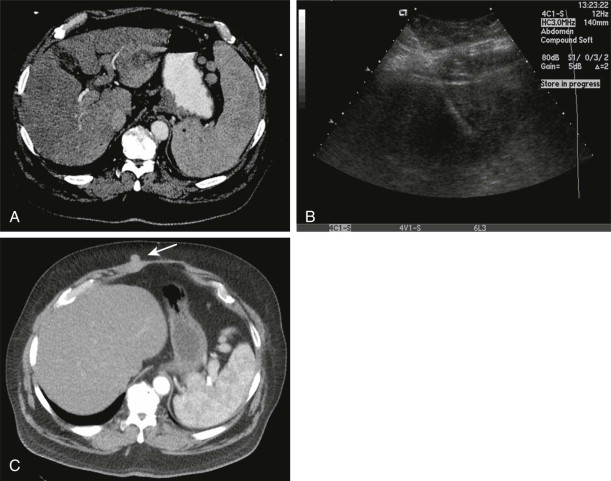
Because most HCCs can be diagnosed on the basis of imaging findings without the need for tissue, biopsy of masses in cirrhotic patients should be rare and reserved for atypical cases that do not meet LI-RADS or AASLD criteria. Tumor seeding after biopsy of metastatic liver lesions is rare and is usually associated with a generalized rapid growth in all tumor sites.
Transjugular Liver Biopsy
Brief Rationale and Indication
Transjugular liver biopsy was first described in humans in 1970 and is the preferred technique for obtaining random tissue samples of the liver in patients with uncorrectable coagulopathies. The most common indications for performing transjugular liver biopsy are coagulopathy, massive ascites, and in conjunction with other procedures such as measurement of hepatic venous pressure gradient or hepatic/caval venography. Acute liver failure, early postoperative liver transplants, and congenital clotting disorders are also scenarios in which transjugular liver biopsy has been shown to be of benefit.
Guidance and Technique
The right internal jugular vein is accessed under ultrasound guidance, and the right hepatic vein is cannulated through the inferior vena cava with an angled catheter. Continuous electrocardiographic monitoring throughout the procedure is recommended to detect arrhythmias induced by passage of the catheter through the heart. The right hepatic vein is preferred because of its angle with the inferior vena cava and the larger size of the right hepatic lobe. Hepatic venography is performed to confirm appropriate positioning. Peripheral punctures should be avoided because of the risk of capsular puncture. The number of biopsy passes is operator dependent; however, an increased number of passes (four vs. three) has been shown to produce longer specimens and a greater number of complete portal tracts for histologic interpretation.
Results and Complications
Transjugular liver biopsy has been shown to have a high success rate with a tissue adequacy rate of approximately 96%. Technical failures are most commonly due to failure to cannulate the hepatic veins. Minor and major complication rates have been reported as 6.5% and 0.56%, respectively. The most common major complication is intraperitoneal hemorrhage secondary to perforation of the liver capsule. Mortality after transjugular liver biopsy is reported as approximately 0.1% and in most cases is due to intraperitoneal hemorrhage or ventricular arrhythmia.
Liver Aspiration and Drainage
Hepatic Abscess
Epidemiology and Symptoms
The mortality from pyogenic liver abscess has decreased from approximately 40% to 6% in the modern era as a result of advances in cross-sectional imaging, antibiotic therapy, and percutaneous image-guided therapy. The incidence of pyogenic liver abscesses has increased in Western countries and is now at a rate of 1.1 to 3.6 per 100,000 people. In Eastern countries such as Taiwan, a higher rate of liver abscesses (17.6 per 100,000) is due to increased rates of cholangitis and parasitic infections. Clinically, patients often present with vague signs and symptoms such as fever, chills, nausea, abdominal pain, and leukocytosis. Most liver abscesses are diagnosed and monitored with CT, MRI, or ultrasound.
Most pyogenic liver abscesses in Western countries are due to biliary disease. Although pyogenic abscesses historically were most commonly due to Escherichia coli , more recent data suggest that Klebsiella pneumoniae is now the most common pathogen causing pyogenic liver abscesses in Western countries. Other causative organisms in the West commonly include Streptococcus and Staphylococcus species, although polymicrobial infections are common. Culture and antimicrobial sensitivity results should always assist in guiding antimicrobial coverage. Other potential causes include septic pylephlebitis related to appendicitis, diverticulitis, and other inflammatory conditions of the intestine that are transmitted to the liver through the portal vein as well as postsurgical or post-traumatic injury, direct extension from contiguous organs, and after interventional oncologic treatments in patients with incompetent sphincters of Oddi. Primary or metastatic liver malignant neoplasms can also rarely be manifested as liver abscesses.
Guidance and Technique
Minimally invasive image-guided percutaneous treatments such as needle aspiration and catheter drainage have supplanted surgical therapy for the treatment of liver abscesses, with significant decreases in hospital stay, overall cost, and morbidity. Surgery still plays an important role for the treatment of recalcitrant abscesses and in the setting of malignant disease. Abscesses smaller than 3 cm are usually treated successfully with parenteral antibiotics alone; however, aspiration may be requested for microbial identification. Image-guided needle aspiration has been shown to be highly effective for unilocular abscesses smaller than 5 cm. Multiple aspiration sessions may be required for complete success. Image-guided percutaneous catheter drainage is preferred for abscesses larger than 5 cm, multiloculated abscesses, or those in direct continuity with bile ducts or bowel.
Preprocedural assessment should include evaluation of coagulation parameters with a target INR below 1.5, aPTT of less than 1.5 times the control value, and platelet count above 50,000/µL. Ultrasound or CT guidance can be used for aspiration or catheter drainage on the basis of the operator’s preference. In choosing a puncture path, the least amount of hepatic parenchyma should be traversed, and care should be taken to avoid damaging adjacent organs or traversing the pleura because of the risk of empyema. Needle aspiration is usually performed with an 18-gauge needle; catheter drainage may be performed by the Seldinger or trocar technique with placement of a multi–side hole, locking catheter. Drainage is usually continued until the patient demonstrates clinical improvement and drainage output is less than 10 to 20 mL/day. A fluoroscopic sinogram can be obtained before catheter removal to assess the residual size of the cavity and the presence of fistulization to the bowel or biliary system ( Fig. 84-4 ).
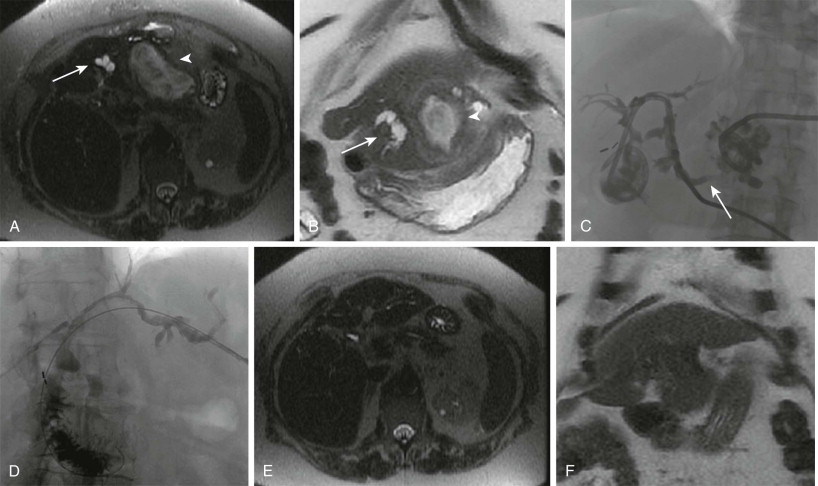
Results and Complications
Success rates for image-guided needle aspiration of simple pyogenic liver abscesses smaller than 5 cm approach 100% with minimal complications. Catheter drainage success rates have varied significantly in the literature from 66% to 100%, probably secondary to abscess and patient factors. Higher failure rates have been associated with the presence of advanced malignant disease, particularly necrotic infected tumors, and the presence of fistulization to an obstructed biliary system. The risk of complications is minimal; complications such as pneumothorax, intraperitoneal hemorrhage, and mild pain are the most frequently reported.
Hepatic Venous Pressure Gradient
Brief Rationale and Indications
Portal hypertension is a complication of chronic liver diseases and is responsible for many of the most severe clinical consequences of cirrhosis. Whereas noninvasive measurements of portal pressure such as elastography are currently being developed, direct measurement of the hepatic venous pressure gradient is the current gold standard for estimating the degree of portal hypertension.
First described in 1951, hepatic venous pressure gradients can aid in the diagnosis and classification of portal hypertension; assessment of prognosis in patients with cirrhosis and portal hypertension–related clinical events including ascites, spontaneous bacterial peritonitis, hepatic encephalopathy, and variceal bleeding; monitoring of response to pharmacologic therapy; and preoperative evaluation in patients with cirrhosis selected for hepatic resection. The hepatic venous pressure gradient is obtained by introducing a balloon occlusion catheter into a hepatic vein and measuring the difference between the occluded venous pressure and free venous pressure. The occlusion of a hepatic vein blocks blood flow in the distal hepatic veins and in the sinusoids; thus catheter pressure measured in this position reflects the pressure in the sinusoids, which in turn reflects portal pressure in sinusoidal or postsinusoidal portal hypertension. Pressure changes within the central venous system are corrected for by subtracting the free hepatic venous pressure from the wedged hepatic venous pressure. This results in a hepatic venous pressure gradient, with clinically significant portal hypertension defined as more than 12 mm Hg.
Hepatic vein pressure gradient ( HVPG ) = Wedged hepatic venous pressure ( WHVP ) − Free hepatic venous pressure ( FHVP )
Guidance and Technique
By use of a transjugular approach and fluoroscopic guidance, a mean pressure measurement is first recorded in the retrohepatic inferior vena cava. All pressure measurements should be recorded with the transducer in a fixed position in the midaxillary line at the level of the right atrium. An occlusion balloon catheter is then advanced into either the right or middle hepatic vein, and the free hepatic venous pressure is measured in the hepatic vein 2 to 4 cm from its opening into the inferior vena cava. The balloon catheter should then be positioned in the middle third or at the transition zone between the middle and distal thirds of the hepatic vein and inflated until complete occlusion is observed by deformation of the balloon. The pressure recording should be allowed to stabilize for 45 to 60 seconds, and then a mean pressure is recorded for the wedged hepatic venous pressure. This process should be repeated at least three times, and finally a wedged hepatic venogram is obtained to evaluate for any venous-to-venous shunting to another hepatic vein that would result in underestimation of the wedged hepatic venous pressure. If venous shunting is identified, the balloon catheter should be placed distal to the shunting; if this is not technically feasible, another hepatic vein should be selected for interrogation.
Results and Complications
The use of balloon occlusion catheters has been shown to correlate more accurately with directly measured portal pressures during placement of transjugular intrahepatic portosystemic shunts (TIPS) compared with the use of an end-hole catheter wedged into a hepatic venule. Technical success rates have been reported above 95%, and failure is usually the result of hepatic venous occlusion. Severe postprocedure complications are exceedingly uncommon.
Transjugular Intrahepatic Portosystemic Shunt
Brief Rationale and Indications
TIPS is a percutaneous image-guided procedure that has proved to be beneficial for the treatment of complications of portal hypertension. First described in an animal model by Rösch and coworkers in 1969, a TIPS is a constructed channel within the liver connecting a portal vein branch to a hepatic vein with the goal of creating a portosystemic shunt to decrease portal venous pressure. A TIPS is created under fluoroscopic guidance by placing a stent graft from a hepatic vein (most commonly the right hepatic vein) through the liver parenchyma into an intrahepatic portal venous branch (most commonly the right portal vein). Another variation is a direct intrahepatic portosystemic shunt, which was first described in 2001. A direct intrahepatic portosystemic shunt is created with use of intravascular ultrasound, passing a needle from the inferior vena cava through the caudate lobe into the portal vein. Subsequent stent placement is performed under fluoroscopic guidance in a fashion similar to TIPS placement. The strongest evidence of TIPS efficacy has been established for secondary prevention of variceal bleeding and treatment of refractory ascites. Other indications for TIPS creation include refractory acute variceal bleeding, portal hypertensive gastropathy, hepatorenal syndrome (types 1 and 2), Budd-Chiari syndrome, hepatic hydrothorax, hepatic veno-occlusive disease, and hepatopulmonary syndrome.
Relative and Absolute Contraindications
Absolute contraindications to TIPS placement include congestive heart failure, severe tricuspid regurgitation, severe pulmonary hypertension (mean pulmonary pressure >45 mm Hg), uncontrolled systemic infection or sepsis, and unrelieved biliary obstruction. Relative contraindications include anatomic issues that can reduce the technical success of shunt placement, such as polycystic liver disease, extensive primary or metastatic malignant disease (especially centrally near the porta hepatis), and obstruction of the hepatic veins or thrombosis of the portal venous system. Hepatic artery thrombosis, severe coagulopathy or thrombocytopenia, and the presence of hepatic encephalopathy may also significantly increase the risk of postprocedure complications.
Preprocedure evaluation includes complete laboratory work-up consisting of a complete blood count, coagulation panel, and comprehensive metabolic panel. Significant thrombocytopenia (platelet count <50,000 cells/µL), anemia (hematocrit <25%), or coagulopathy (INR > 1.5) should be corrected and arrangements made for crossmatched blood products. Recent cross-sectional imaging within 1 month should be reviewed to assess for vascular patency and anatomic considerations. If recent imaging is unavailable or an abrupt deterioration of hepatic function occurs, an urgent or emergent ultrasound with Doppler evaluation of the hepatic vasculature may be warranted to assess vascular patency. Echocardiography is also recommended to assess cardiac function and to specifically assess for pulmonary arterial hypertension.
Guidance and Technique
TIPS procedures are mostly performed by use of general anesthesia with endotracheal intubation. The right hepatic vein is accessed through the right internal jugular vein, and balloon occlusion hepatic venography using carbon dioxide can be performed to visualize the course and flow in the portal venous system. Fluoroscopically guided needle passes are then made to achieve portal venous access. Direct portal venous pressure is measured, and a portosystemic pressure gradient is calculated as the pressure difference between the main portal vein and right atrium. A stent graft is deployed so that the uncovered caudal portion of the stent remains in the portal vein, with the cranial end terminating near the junction of the hepatic vein and inferior vena cava. Polytetrafluoroethylene (PTFE)–covered stent grafts have become the standard of care for de novo TIPS placement compared with bare metal stents because of a lower rate of TIPS dysfunction, higher rate of primary patency, lower rate of clinical relapse, and decreased incidence of hepatic encephalopathy. After deployment, trans-TIPS portal venography and pressure measurements in the main portal vein and right atrium are repeated. In patients with variceal bleeding, a post-TIPS portosystemic gradient below 12 mm Hg should be achieved to prevent rebleeding. Retrospective studies have shown that variceal embolization with coils, cyanoacrylate, or sclerosing agents such as sodium tetradecyl sulfate in addition to TIPS placement significantly decreases the risk for recurrent variceal bleeding. For patients with refractory ascites, the Society of Interventional Radiology and the AASLD guidelines recommend reducing the portosystemic gradient to below 8 mm Hg. A portosystemic gradient below 5 mm Hg has been associated with an increase in the risk of liver failure and severe hepatic encephalopathy requiring an intervention such as TIPS reduction ( Fig. 84-5 ).
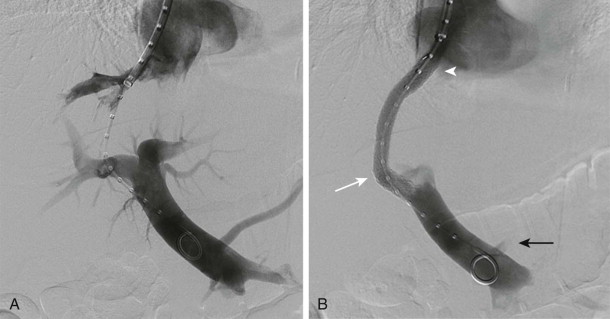
Results
Success after TIPS placement can be classified as technical, hemodynamic, and clinical. Technically successful creation of a shunt from the hepatic vein to the portal vein and hemodynamic success in reduction of a portosystemic gradient to below 12 mm Hg with nonfilling of gastroesophageal varices should be achieved in more than 95% of cases. Clinical success for the separate indications for TIPS placement varies among studies because of different inclusion and evaluation criteria. Meta-analyses evaluating TIPS for secondary prevention of variceal bleeding compared with various forms of endoscopic therapy found a threefold decrease in the risk of recurrent bleeding after TIPS with similar all-cause mortality rates. However, a more than twofold increased risk for development of hepatic encephalopathy was noted after TIPS. A multicenter randomized controlled trial in high-risk patients with acute variceal bleeding demonstrated a statistically significant decrease in treatment failure and mortality for patients undergoing TIPS placement compared with those treated with repeated endoscopy and pharmacotherapy.
In the treatment of refractory ascites, a meta-analysis of randomized controlled trials demonstrated a 7.1-fold reduction in the risk of recurrent ascites, with rates of improvement ranging from 38% to 84% after TIPS compared with 0% to 43% after large-volume paracentesis. No significant survival advantage for TIPS was found in early studies. A more recent randomized controlled trial in a select group of patients with preserved hepatic and renal function did demonstrate a significant survival advantage after TIPS placement. The rate of hepatic encephalopathy in this group of patients was 2.2-fold higher after TIPS. TIPS also is likely to lead to survival prolongation in patients with Budd-Chiari syndrome, with 1- and 5-year liver transplant–free survival of 88% and 78%, respectively, with a median Model for End-Stage Liver Disease (MELD) score of 17. At least partial improvement in clinical symptoms is experienced in 68% to 82% of patients with hepatic hydrothorax, and complete resolution of the hydrothorax occurs in 57% to 71% after TIPS creation.
Complications
Patients with a MELD score above 18 have a significantly higher mortality 3 months after TIPS than that of patients with a MELD score of 18 or less. Technical complications such as transcapsular puncture may occur in up to 33% of cases but result in significant intraperitoneal hemorrhage in only 1% to 2%. Stent migration or misplacement into the inferior vena cava or portal vein may occur in up to 10% to 20% of cases. Creation of the shunt results in diversion of portal blood flow past the metabolic filtering effect of the hepatic parenchyma, which can lead to new or worsening hepatic encephalopathy in 10% to 44% of patients. Patients of advanced age, with a past history of encephalopathy, and with advanced liver disease are at increased risk for development of hepatic encephalopathy. However, encephalopathy usually responds to medical treatment, and only rarely is TIPS reduction or occlusion necessary. Other potential complications, such as hepatic infarction, creation of a biliary-venous or hepatic artery–portal vein fistula, hemolysis, sepsis, and TIPS infection, are much rarer.
The introduction and widespread use of PTFE-covered stent grafts for TIPS creation has led to a dramatic improvement in long-term TIPS patency. The incidence of shunt dysfunction with bare metal stents ranges from 18% to 78%, probably secondary to intimal hyperplasia protruding through the stent interstices. Primary patency rates at 1 year with PTFE-covered stent grafts have been reported at 81% to 86% ( Fig. 84-6 ).
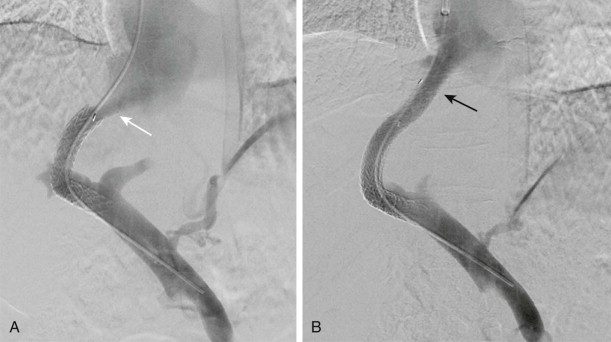
Follow-Up and TIPS Revision
Despite the decrease in TIPS dysfunction after implementation of PTFE-covered stent grafts, regular shunt surveillance is recommended. One protocol for surveillance uses Doppler ultrasound at 1, 3, 6, and 12 months after TIPS creation and every 6 to 12 months thereafter, depending on the patient’s clinical status. A significant degree of variability regarding the sensitivity and specificity of Doppler ultrasound for detection of TIPS dysfunction has been reported. Measured velocities throughout the course of the shunt should range between 90 and 190 cm/s. Direction of flow within the left portal vein should be hepatofugal (toward the shunt) after TIPS placement. Left portal vein hepatopetal flow (toward the liver) is therefore indicative of shunt dysfunction. Temporal changes in the peak velocity greater than 50 cm/s have also been recommended as an indication for venography and pressure measurements, which are the gold standard for assessing TIPS dysfunction. Patients with symptoms suggestive of recurrent portal hypertension in the setting of normal findings on Doppler ultrasound also warrant further investigation with venography. In the setting of TIPS dysfunction secondary to stenosis, repeated stenting has been shown to lead to better patency than angioplasty alone. Patients with a bare metal stent requiring reintervention should be revised with a PTFE-covered stent graft.
Interventional Oncology in the Liver
Liver-directed locoregional therapies for unresectable primary and metastatic malignant neoplasms continue to evolve with advances in ablative and endovascular technologies and techniques. The goal of liver-directed therapy in patients with hepatic malignant neoplasms can be multifaceted and vary from allowing a patient with unresectable disease to become a resection or transplant candidate to curative treatment in nonsurgical candidates or improvement of survival and quality of life in a palliative setting. The choice of treatment modality depends on multiple factors including the presence of underlying liver dysfunction, tumor type, overall tumor burden, number and location of tumors, and patient factors such as performance status and functional liver reserve. The most common locoregional therapies currently being used are portal vein embolization (PVE), radiofrequency (RF) and microwave (MW) ablation, transarterial chemoembolization with or without drug-eluting beads (TACE and DEB-TACE), and transarterial radioembolization. Other liver-directed therapies, such as percutaneous ethanol ablation, cryoablation, and other ablative technologies such as laser-induced interstitial thermotherapy, high-intensity focused ultrasound, irreversible electroporation, hepatic arterial infusion chemotherapy, and transarterial bland embolization, have also been reported and play a lesser role in current treatment algorithms. The latter techniques either are still in development or are of historical interest and are beyond the scope of this chapter.
Preoperative Portal Vein Embolization
Brief Rationale
Surgical resection of both primary malignant neoplasms and liver metastases remains the mainstay of curative therapy. Continued advances in surgical techniques have led to improved overall patient outcomes; however, major hepatic resection places patients at risk for liver insufficiency. Determination of the volume of liver that remains after surgery, the future liver remnant (FLR), has been shown to be a strong independent predictor of postoperative complications. In patients with an inadequate FLR, PVE of the lobe to be removed can cause hypertrophy to the FLR and atrophy of the embolized segment. PVE has been most commonly used in patients with colorectal metastatic disease, intrahepatic cholangiocarcinoma, and HCC. Whereas the exact mechanisms of hepatocyte regeneration are unknown, studies have demonstrated that in addition to redistribution of portal venous blood flow, PVE also induces an increase in hepatic growth factor and transforming growth factors α and β. FLR hypertrophy rates have been noted to be impaired in patients with underlying cirrhosis or fibrosis and in diabetic patients.
Indications
Patients undergoing major hepatic resection may benefit from preoperative determination of the FLR. CT volumetry is used to measure the volume of the FLR. Calculations with either the measured total liver volume minus the tumor burden volume or the estimated total liver volume by use of body surface area are performed to assess percentage of FLR after resection. PVE is recommended in patients with preserved liver function and an FLR of less than 20%, patients with either hepatic steatosis or significant exposure to hepatotoxic chemotherapy and an FLR of less than 30%, and patients with well-compensated cirrhosis and an FLR of less than 40%. Relative contraindications to PVE include extrahepatic metastases, uncorrectable coagulopathy, extensive tumor burden precluding safe access into the portal vein, and tumoral invasion into the portal vein.
Guidance and Technique
Multiple different approaches and techniques have been described for PVE. Historically, PVE was performed through an intraoperative transileocolic approach. Currently, the most commonly used approach is the percutaneous ipsilateral approach. This approach is favored over the contralateral approach because of the avoidance of potential damage to the FLR during access and catheter manipulation. However, if there is no safe route through the ipsilateral liver because of tumor burden, a contralateral approach remains an acceptable alternative. With use of either conscious sedation or general anesthesia, a peripheral branch of the portal venous system to be embolized, most commonly the right, is accessed with a micropuncture needle under ultrasound guidance. Once access is secured, portal venography is performed, and the portal venous system is embolized, depending on the planned surgery. Most commonly, this involves embolizing segments V to VIII of the liver for a right hepatectomy. For an extended right hepatectomy, some authors have advocated embolizing segment IV as well to obtain a greater hypertrophy rate in segments II and III, although the risk of nontarget embolization to the FLR is theoretically greater. The choice of embolic agent is at the discretion of the operator and includes n -butyl cyanoacrylate mixed with Lipiodol and polyvinyl alcohol particles or gelatin sponge and coils. A meta-analysis suggested that the use of n -butyl cyanoacrylate results in a higher percentage of FLR volume increase. After embolization is complete, the sheath track is embolized with either gelatin sponge or coils.
Results and Complications
Technical success rates of PVE are approximately 99%. Most technical failures result from an inability to cannulate the portal system because of altered anatomy or unexpected thrombosis of the portal system due to tumor progression or invasion. After embolization, follow-up CT is performed at 4 weeks for recalculation of the FLR as liver regeneration tends to plateau after 3 weeks. The clinical success rate (defined as sufficient FLR hypertrophy to allow resection) is 96.1% with a mean increase in the FLR volume of 37.9%, with a range of 20.5% to 69.4% ( Fig. 84-7 ). However, approximately 20% of patients do not undergo the originally planned liver resection secondary to local intrahepatic tumor progression, new tumor in the FLR, extrahepatic tumor spread, insufficient hypertrophy of the FLR, complications of PVE leading to nonresectability, or refusal of the patient for further treatment. Some select patients may benefit from combination treatment with either transarterial or ablative treatments to achieve curative resection.
