IVIM MRI Analysis with Low Perfusion
This chapter reviews the application of intravoxel incoherent motion (IVIM) model in low-perfused tissues. Although more commonly applied in the abdominal organs, such as liver and kidneys, IVIM can also provide valuable information in the brain. Brain and many of its related pathologies are relatively low perfused compared to the abdominal organs, but the use of a noninvasive measure of perfusion would be advantageous. However, the application of IVIM in low- perfused tissues is challenging and requires careful consideration before it can provide reliable measures for routine clinical practice and clinical decision making.
24.1 Introduction
The application of IVIM model to multi-b-value diffusion-weighted magnetic resonance imaging (DWMRI) has garnered more interest in recent years. While originally applied in the brain [1], the IVIM model has been more commonly used in the abdomen, where relatively high-perfused organs, such as the liver and kidneys, have highlighted its potential [2–5]. However, the advantages of deriving quantitative parameters of both diffusion and perfusion with a single protocol are also of interest for the brain. The IVIM MRI has the potential to provide an assessment of perfusion in patients with renal failure or allergy, where administration of contrast agents is contraindicated. The recent reports of gadolinium deposits in the brain have also posed questions on the safety of gadolinium-based contrast agents, which are used in the “gold standard” perfusion MRI for the brain, the dynamic susceptibility contrast (DSC) imaging [6, 7]. In this chapter, the application of IVIM MRI in low-perfused tissues, such as those found in the brain, is reviewed.
24.2 Tissue Perfusion
This section briefly introduces the concept of tissue perfusion, why it is a valuable quantitative measure, and how it changes in pathological tissues.
Tissue perfusion, more commonly described as blood perfusion, has an important role in the delivery of oxygen and nutrients to cells in tissue [8]. The oxygenated arterial blood circulates in the microcapillary network, and it is delivered to the tissue via the blood– tissue exchange through the capillary walls. The same network is also responsible, along with lymphatics, for the transportation and removal of waste products resulting from tissue metabolism. These processes are affected by many different parameters related to the microvascular anatomy, blood circulation, and the exchange interface between blood and tissue. Therefore, a measure of perfusion can provide valuable information about the underlying microvascularity and the viability of the tissue.
The level—or degree—of tissue perfusion depends on the type of tissue and can vary to a great extent between different organs. The most highly perfused organ is the kidney, which comprises 20%–25% of the cardiac output, that is, the blood volume pumped by the heart per minute [9]. In comparison, the cerebral blood flow in the brain is approximately 15%–20% of the cardiac output, due to the high metabolic demand and brain’s inability to store energy [10]. Both of these organs are essential for life and hence sensitive to any disruptions in their respective blood supply. Autoregulation processes, which are intrinsic abilities of these organs, exist to maintain or adjust the blood flow due to any deviations. In the brain, these specialized processes manage the high metabolic demands of the brain and prevent the significant consequences that could result from cerebral ischemia [10].
24.2.1 Perfusion in Tissue Pathologies
An increase in the blood flow to specific brain regions is connected to the activation of cognitive functions [11]. This provides the link for perfusion to measure cognitive decline, for example, in diseases such as Alzheimer’s [12, 13]. In general, any disruption of blood supply and hence perfusion can result in malfunction of tissue and ultimately lead to tissue death [14]. In many brain diseases, such as traumatic brain injury [15, 16], ischemic stroke [17, 18], and brain tumors [19], perfusion changes occur due to the suppression of the previously mentioned autoregulation processes. Many of these disease states also present as low-perfused tissue, although for brain tumors, this is more characteristic of low-grade gliomas [20–22].
The vasculature found in tumors is complex and is known to be unevenly distributed with irregular branching of the tumor vessels, with poorly defined hierarchy [23]. As a result, the blood flow in tumors is not constant along a single direction and may follow different paths and even change direction through the same vessel. In contrast, the vasculature in healthy tissues is arranged in a hierarchy of well-differentiated components. The tumor perfusion provides an important marker of neoangiogenesis, which is characteristic of, for example, high-grade gliomas. Previously, the histopathological assessment of gliomas has showed that the increase in the number of blood vessels progressed with the glioma grade [24]. The increase in tumor perfusion, measured with DSCMRI–derived cerebral blood volume (CBV), was also shown to be a potential biomarker for the detection of malignant progression of low-grade gliomas [25]. These studies highlight the use of perfusion in gliomas, which is also one of the applications previously studied with IVIM MRI [26, 27].
Another common pathology with characteristically low perfusion is the ischemic stroke. Multiple disease mechanisms can result in a stroke, although they all share the aspect of disruption occurring in the cerebral blood flow [10]. In an ischemic stroke, the lack of blood flow due to a narrowed or blocked artery can result in cell death. Perfusion MRI has the potential to support the rapidly required treatment decisions for acute stroke patients. However, the relative ease of applicability of DWMRI, and its extraordinary ability to confirm the diagnosis of a stroke, has meant that DWMRI has become the “gold standard” for its detection [28]. Nevertheless, the diagnosis of transient ischemic attack, where an artery is temporarily blocked, was not always detectable by DWMRI, while changes were observed in a perfusion MRI [29, 30]. This presents a favorable role for the use of IVIM MRI, which is able to derive parameters of both diffusion and perfusion in a single protocol.
Low perfusion is also presented in breast tissue and breast cancer. MRI has become an important tool in the characterization of breast cancer, and in particular the dynamic contrast–enhanced (DCE) MRI has shown promise in being highly sensitive in its detection [31, 32]. Similar to the brain gliomas, the vascularity found in the less aggressive lesions or more specifically, benign lesions, has been found to be lower compared to the malignant cases [33]. However, previous studies have shown that the use of DCE MRI can result in false positives, and DWMRI has been applied to increase the specificity [34, 35]. Once again, the combined use of diffusion and perfusion MRI provides a more beneficial scenario and a more complete investigation.
The vasculature in tumors and other diseased states provides a complicated microenvironment for investigation. The use of imaging methods, such as IVIM MRI, unique with noninvasive measures of both perfusion and diffusion, can offer invaluable information in the different steps of clinical routine, from diagnosis to the assessment of treatment response.
24.3 IVIM MRI Studies in Low-Perfused Tissues
The IVIM model has provided an alternative tool for the assessment of perfusion in tissues, while also providing a quantitative measure of diffusion [1, 36]. The tissues often considered to be low perfused in “IVIM terms” have been characterized with perfusion fraction (f) values of f ≈ 0.1 or lower. This accounts for approximately 10% of the diffusion MRI signal arising from the microvascular compartment [37]. The other perfusion parameter provided by the IVIM model is the pseudodiffusion coefficient (D*), which describes the incoherent movement of blood in the microvascular compartment. The ratio of D* to the tissue diffusion coefficient (D) determines the degree of the biexponential behavior in the tissue and provides an important quantity in the context of applying a biexponential fitting model to the data. Examples of IVIM parameters reported for healthy tissues of low perfusion are listed in Table 24.1, including both brain and breast tissue.
Table 24.1 Examples of previously reported IVIM parameters in low-perfused healthy tissues
Note: D and D* are in units of ×10−3 mm2/s. Standard deviations (±) or quartile ranges (lower quartile, higher quartile) are reported.
The complex nature of the tissue vasculature of low-perfused tumors and other disease states has been previously investigated with the IVIM model. Some of the previous studies and the reported IVIM parameters are listed in Table 24.2. In the brain-related studies of stroke and gliomas, the IVIM parameters D and f have generally been found to be the most clinically valuable. For example, the IVIM f has shown value in differentiation of low- and high-grade gliomas [26,27,42,43], in differentiation of recurrent tumor from treatment effects in glioblastoma, [44] and in being a prognostic biomarker in glioma patients [45]. In the detection of stroke, significantly decreased f values have been observed in the infarcted regions [20,21,46,47], together with a decrease in the D parameter, which agrees with the expected pathophysiology of a stroke [21].
Table 24.2 Examples of previously reported IVIM parameters (±standard deviation) in pathological tissues of low perfusion
Note: D and D* are in units of ×10−3 mm2/s.
# IVIM-D estimated using the kurtosis IVIM model.
† Deviation reported as an interquartile range.
The most common glioma type in adults is the high-grade anaplastic astrocytoma (grade III) and the glioblastoma multiforme (grade IV). However, in pediatrics the low-grade pilocytic astrocytoma (grade I) is the most common glioma type and the high-grade gliomas are much rarer [48]. This means that most of the glioma population in pediatrics is characterized by low perfusion. An example of a pediatric low-grade glioma (grade I) with IVIM D and f maps is presented in Fig 24.1 and for a comparison, a highly perfused choroid plexus tumor is also shown. The low-grade glioma appears hypoperfused compared to the surrounding brain tissue, while the choroid plexus tumor indicates the high vascularity commonly associated with these types of tumors [49].
Figure 24.1 Example cases of pediatric brain tumors with low and high perfusion and the corresponding IVIM parameter maps D and f. (a) Low perfusion shown in a low-grade glioma (pilocytic astrocytoma) and (b) high perfusion in an atypical choroid plexus papilloma (grade II). The arrows indicate the location of the tumor, and the units of D are reported in ×10−3 mm2/s.
In addition to brain studies, the IVIM model has also been applied in breast cancer, where the IVIM-derived perfusion fraction has indicated relatively low perfusion in some studies, particularly for benign lesions. The focus in many of these studies was the discrimination of malignant lesions from benign ones [33,40,52,53], which was typically possible with the D and f parameters. However, in most cases, the D* parameter was shown to have high variability, similarly to the previous brain studies, and was not able to discriminate between the tumors [27,33,37,52]. More recently, the IVIM parameters have also shown potential as prognostic biomarkers of the treatment response to neoadjuvant treatment (NAT) [54, 55].
While the application of IVIM in clinical studies of the brain and breast has shown promise in both diagnosis and prognosis, the results have almost solely relied on the IVIM parameters D and f. In the following section, some of the challenges contributing toward the unreliability of D* and the IVIM analysis in low-perfused tissues are discussed.
24.4 Application of IVIM MRI in Low-Perfused Tissues
The use of IVIM or any quantitative technique as a clinical application requires it to be sufficiently robust in the process of image acquisition and processing, and data fitting and analysis. Previous studies have demonstrated that the reliable derivation of IVIM parameters depends on the chosen b values and hence the DWMRI protocol, as well as the methods used for postprocessing [20,37,56,57]. In this section, the IVIM analysis applied to low-perfused tissues and the technical challenges of its use are reviewed.
24.4.1 IVIM MRI Analysis
IVIM fitting is commonly performed using nonlinear least-squares approaches; and, more recently, the Bayesian probability methods have been used [56,58,59]. The nonlinear least-squares fitting can be constrained to different extents, and the fitting for the parameters can be performed simultaneously or in a stepwise fashion. As most of the image data is influenced by noise, the simultaneous fitting is rarely feasible due to its high degree of freedom and hence higher requirement for the signal-to-noise ratio (SNR). In previous studies focusing on the abdominal regions, the stepwise fitting, also known as the segmented approach, has been found to compute the most robust or reliable IVIM parameters [59, 60]. This was also found to be the case in a simulation study investigating low-perfused tissues of pediatric low-grade gliomas and healthy gray matter [37]. The IVIM parameters derived in this study with the segmented method were found to be significantly different to those derived with the simultaneous fitting. The segmented fitting also produced a reduced number of outliers and improved the accuracy and reproducibility of the IVIM parameters. This was particularly the case at lower SNR levels (≤ 40).
24.4.1.1 Estimation of the pseudodiffusion coefficient D*
The low-b-value region is critical for the evaluation of the IVIM perfusion parameters, that is, the pseudodiffusion coefficient D* and the perfusion fraction f. However, as indicated in many previous studies, the determination of IVIM D* has proved challenging [37,38,59]. Depending on the characteristics of the signal, and in particular its degree of biexponential behavior, the IVIM D* can be derived with some level of robustness. When D* >>D by at least an order of magnitude, the biexponential fitting is relatively stable [61]. However, for cases where the difference between the two diffusion coefficients is smaller, the fitting scenario becomes more challenging and leads to a poor estimation of D* [37, 38]. In the brain, a previous study by Wu et al. [38] reported the average error of estimating D* to be 100% and 300% at an SNR = 30, for simulated tissues of gray matter and white matter, respectively [38]. In the same study, the errors were estimated to be 5% in gray matter and 10% in white matter for the perfusion fraction parameter. These results agree with other studies performed in the brain and also in previous studies of breast [60, 62]. Therefore, the overall indication is that the D* parameter is not sufficiently robust for use in clinical practice with the current least-squares fitting approaches in low-perfused tissues. An example of a young adult volunteer is presented in Fig 24.2, showing the DW signal data in gray matter and white matter and the estimated IVIM parameter maps. The signal decay for the white matter shows the characteristically weak “IVIM effect” compared to that for the gray matter.
Figure 24.2 An example volunteer case analyzed with the IVIM model. The diffusion-weighted images of (a) b = 0 and (b) b = 1000 s/mm2; the IVIM parameter maps of (c) D, (d) D*, and (e) f; and the diffusion-weighted signal decay in (f) gray matter and white matter. The IVIM parameters maps were estimated using the segmented fitting approach with b-value distribution: 0, 20, 40, 80, 110, 140, 170, 200, 300, 500, and 1000 s/mm2. Both D and D* are in units of mm2/s.
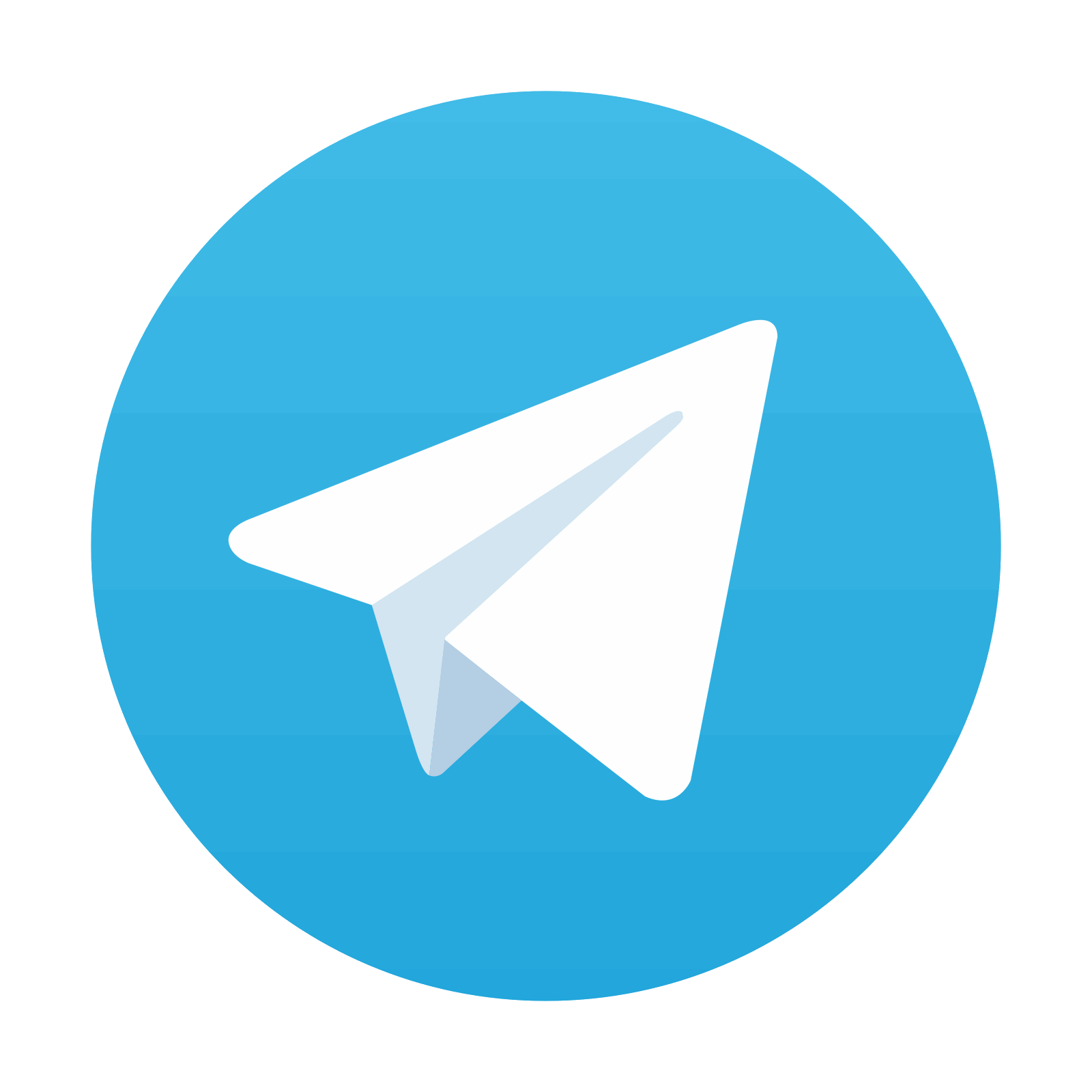
Stay updated, free articles. Join our Telegram channel

Full access? Get Clinical Tree
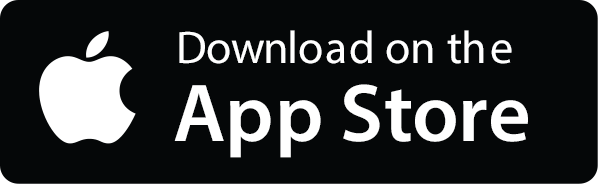
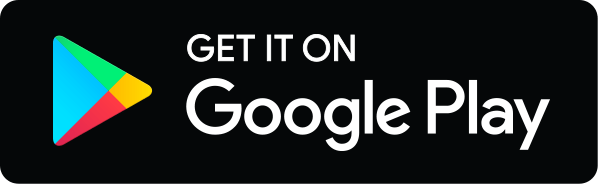