and Ashutosh Dash2
(1)
Nuclear Security and Isotope Division, Oak Ridge National Laboratory, OAK RIDGE, USA
(2)
Isotope Production and Applications Division, Bhabha Atomic Research Centre, Mumbai, India
13.1 Introduction
In the USA, for instance, skin cancer is the most common neoplasia with an estimated 3.5 million skin cancers in over two million people (Rogers et al. 2010). In fact, it has been estimated that there are more cases of skin cancer annually in the USA than the combined annual totals for breast, colon, lung, and prostate cancer. In the USA, it is estimated there are approximately ~700,000 cases of SCC and 2.8 million of the most common and typical BCC cases diagnosed annually. The BCCs generally grow slowly and are generally unlikely to spread. Some of these cancers are disfiguring and may metastasize, and for these and other reasons, the availability of effective treatment methods is important. Although relatively recently introduced and not yet widely used, the topical application of therapeutic radioisotopes for the irradiation of nonmelanoma skin cancer (NMSC) offers a practical and expected inexpensive and effective strategy for the therapeutic option. Such technologies are also useful for the treatment of hemangiomas and other superficial skin diseases. These tumors are generally radiosensitive and are thus often good candidates for topical treatment with radioisotopes.
13.2 Radioisotopes for Treatment of Skin Cancer
The use of such radioactive skin patches, molds, and application strategies involves individualized preparation and application to correlate with the patient-specific anatomical requirements. These radioactive materials are only temporarily applied on generally on an outpatient basis for time periods required to deliver the prescribed radiation dose. Although this technology may thus be designated as “topical brachytherapy,” the on-site preparation of these materials involving the use of unsealed source radioactive materials is generally conducted in a nuclear medicine facility, as compared to the broader use of sealed sources in brachytherapy which lies under the purview of radiation oncology.
13.3 Strategies for Treatment on NMSC
Although surgery is often the first-line strategy, often tumors cannot be readily removed because of anatomical location, etc., and alternative technologies such as external radiation therapy with neutrons, electrons and X-rays, brachytherapy, and photodynamic therapy, have been widely used. Traditional strategies for treatment of nonmelanoma skin cancers include surgical resection, use of fractionated external X-ray therapy with 116 keV X-rays, and traditional brachytherapy molds (Rustgi and Cumberlin 1993). Although these strategies focus on the therapy of nonmelanoma skin cancers, such approaches have also been used to treat cutaneous malignant melanoma using 106Ru plaques (Anteby et al. 1993) and 125I-seeds on gold plaque. As shown in Fig. 13.1, the maximal treatment depth is determined by the lesion dimensions, and based on the penetration depth of the radioactive emissions of the radioisotope patch used, dosimetry estimates then prescribe the contact time of the radioactive patch.
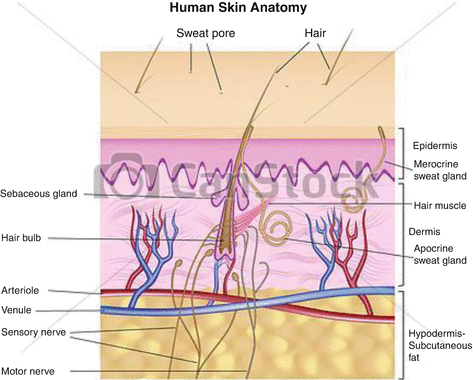
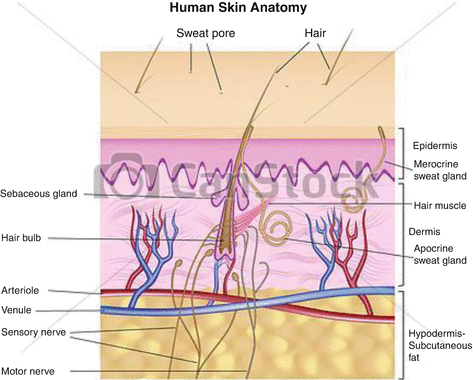
Fig. 13.1
Anatomical cross section illustrating the layers of skin
13.4 Topical Use of Radioisotopes for NMSC Therapy
As an attractive alternative to either surgery or invasive brachytherapy, the topical use of radioisotopes offers an effective, simple approach and expected cost-effective technology in many cases, especially where cosmetic results would be difficult to attain by traditional methods. In addition to radioisotope availability and cost, both total dose and dose rate are also important factors which must be evaluated for dose planning for consideration of using radioisotopes for this application. Because of health costs and other practical issues, the use of radioisotopes with physical half-lives which permit outpatient treatment would be expected to be of increasing interest. Numerous studies have thus established that the use of therapeutic radionuclides for the superficial treatment of skin cancers is an attractive simple and cost-effective strategy, especially when cosmetic issues are considered. Although the best radionuclide(s) of choice have not yet been established, it would be expected that the issue of costs and availability will be important factor which will determine which technology will move forward on a broader scale.
As described in Table 13.1, a variety of radioisotopes, agents, and therapeutic strategies have been evaluated for topical treatment/contact brachytherapy of NMSC and other experimental and natural skin diseases. Although the chemical nature of the materials formulated with these radioisotopes is not sophisticated, the substrates to which the radioisotopes are attached are important to insure good radiological safety handling, with minimal contamination of patients and staff. Most recent efforts have focused on the use of 32P, 166Ho, and 188Re patches/creams for this application. Although this technology has not yet reached mainstream application, it would be expected that the benefits of these methods and similar approaches using other therapeutic radioisotopes would represent sufficient benefit to propel this technology forward (Fig. 13.2).
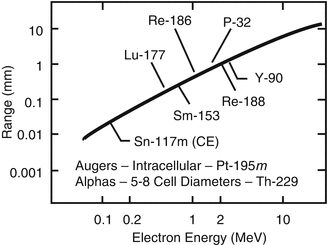
Table 13.1
Examples of therapeutic radioisotopes which have been evaluated for superficial brachytherapy of skin cancer
Radioisotope | T1/2, Eβmax, MeV Approx. mm average soft tissue penetrationa | Agent for application | Reference |
---|---|---|---|
Gold-198 | 2.7 days, β− max 0.96 ≈4.2 mm | Gold grains | Lock et al. (2011) |
Holmium-166 | 26.8 h, β− max 1.85 ≈0.8 mm | Polyurethane patch | Chung et al. (2000) |
Iodine-131 | 8.02 days, β− max 0.606 ≈4.2 mm | Metaiodobenzylguanidine (also emits high-energy gammas) | |
Iridium-192 | 73.8 days, β− max 0.672 ≈4.1 mm | Afterloader (also emits high-energy gammas) | Rustgi and Cumberlin (1993) |
Phosphorus-32 | 14.29 days, β− max 1.71 ≈8 mm | Patch | Gupta et al. (2009) |
Rhenium-188 | 16.9 days, β− max 2.12 MeV ≈9 mm | Cream | Sedda et al. (2008) |
Yttrium-90 | 64.1 h, β− max 2.3 MeV ≈10 mm | Ferric hydroxide Macroaggregates Immobilized on gauze | Mukherjee et al. (2002) Saxena et al. (2014) |
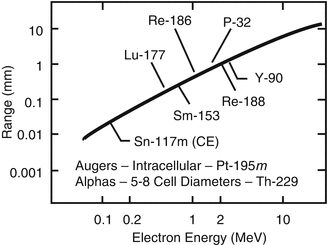
Fig. 13.2
Illustration of approximate maximal beta particle soft tissue penetration as a function of endpoint energy
A variety of animal studies and clinical trials have demonstrated the usefulness of using the topical brachytherapy approach for treatment of BCC and SCC for which other therapeutic strategies are either difficult or costly. These include cancers of anatomical regions which are not candidates for surgery for cosmetic reason, such as near the lips, eyes, and nose. A number of radioisotopes and applicator strategies have been reported for treatment of BCC and SCC and also for benign hemangiomas.
13.4.1 Holmium-166
The relatively short 26.8 h half-life of 166Ho is attractive for use for high-dose-rate outpatient therapy of skin cancers. Early evaluation of the potential use of 166Ho for skin cancer therapy utilized a patch attached to chemically induced skin cancers (Lee et al. 1997). The 166Ho-labeled patches were produced in situ by reactor irradiation of macroaggregated 165Ho particles embedded on adhesive tape at low thermal flux (1 × 1013 n/cm2s). This is one important example when sufficiently high specific activity can be obtained for therapeutic applications at relatively low thermal neutron flux. The patches were affixed to the squamous cell carcinomas and keratoacanthoma tumors induced in ICR and hairless mice with TDA. The 5-mm skin patches were attached to the tumors with adhesive tape for 2 h (n = 4; 22.2 MBq and 29.6 MBq) and for 1 h (n = 5; 44.4 MBq and 48.1 MBq). The radiation dose at the surface ranged from 42 to 45 Gy. One to two weeks after treatment, the tumors were destroyed and there was no damage to the underlying soft tissues. In the usual manner, ulceration was accompanied by tumor site infiltration by inflammatory cells, edema, and exudate, but complete healing was observed after 7 weeks. The dose–depth distribution from decay of 166Ho has also been for different shaped sources using the VARSKIN3 Code and has confirmed that radiation damage to underlying bone and other tissue is minimized by the rapid depth–dose falloff (Mowlavi et al. 2010).
A small number of patients with histologically confirmed SCC (3), BCC (1), and Bowen’s disease (1) were also treated with the 166Ho topical patches embedded with 273.8–999 MBq (7.4–27 mCi) of 166Ho for periods from 30 min to 1 h (Lee et al. 1997). After a period of 8 weeks, skin biopsy samples from four patients were available for histological examination, and successful tumor destruction was observed in all cases. Acute radiation-induced effects of ulceration, etc., were observed but healed over a one-month period with no adverse reaction or tumor cell recurrence after 8–20-month follow-up. Another clinical study evaluated the efficacy of 166Ho therapy in patients with Bowen’s disease (intraepidermal basal cell carcinoma) by treatment of 29 sites in 8 patients (Chung et al. 2000). Informed consent and complete clinical work-up were completed. The applicator in this study involved dissolution of equal amounts of 165Ho(NO3)3•5 H2O (2.4 g) and polyurethane in 4 mL of dimethylformamide (DMF) at room temperature. The solution was “cast” on an aluminum dish and the DMF removed by evaporation to yield a patch containing uniformly distributed 165Ho from which pieces were removed for reactor irradiation at low thermal neutron flux (1.25 × 1013 n/cm2s) to produce the 166Ho-labeled patch material. The required size and shape of the patch for each individual tumor site could be readily obtained by cutting the urethane preparation. A total dose of 35 Gy (3500 rads) were delivered by attachment to the tumor surface for 30–60 min. The lesions spanned from 3 to 7.2 cm and post-therapy follow-up was from 10 months to 2 years.
13.4.2 Phosphorus-32
Many recent studies have described the development of various application technologies and clinical results of 32P for the treatment of basal and squamous cell carcinomas and hemangiomas. Advantages for use of 32P include its practical radionuclidic properties (Table 13.1) and availability of 32P phosphate and chromic phosphate as approved cGMP-produced radionuclide preparations. The 14.2-day physical half-life provides convenience for preparation of skin irradiation patches at a centralized site for distribution to clinical centers. In addition, the absence of gamma irradiation optimizes radiation protection issues to minimize radiation dose to both staff and patients. Finally, the emission of 1.7 MeV beta particles optimizes dose to the cutaneous dermal layer.
A variety of approaches have been evaluated for preparation of 32P dose applicators for treatment of BSC and SCC and other animal skin disease models. Radioactive “bandages” have been described in which 32P chromic phosphate particles were bound within Millipore filters which were then immobilized and sealed within nitrocellulose membranes (Pandey et al. 2008a; Mukherjee et al. 2003). The sealed membranes were then placed on adhesive bandages which were then placed on the superficial tumors of melanoma-bearing C57BL/6 mice for a 74 MBq single dose evaluation of tumor regression. Another approach focused on (Pandey et al. 2008b) adsorption of 32P orthophosphate on circular sheets of cellulose-based adsorbent paper. These preparations of various shapes and sizes could be readily prepared and contained 37–74 MBq/cm2 of 32P which were then immobilized/sealed between plastic sheets. The 32P was uniformly distributed on the paper adsorbent preparations which showed no activity leakage in either water or saline. Complete tumor regression was observed using these brachytherapy molds with a dose rate of 10 Gy/h in C57BL6 mice with cutaneous melanoma tumors. Evidently, this technology has not yet progressed to evaluation in humans presenting with skin cancers.
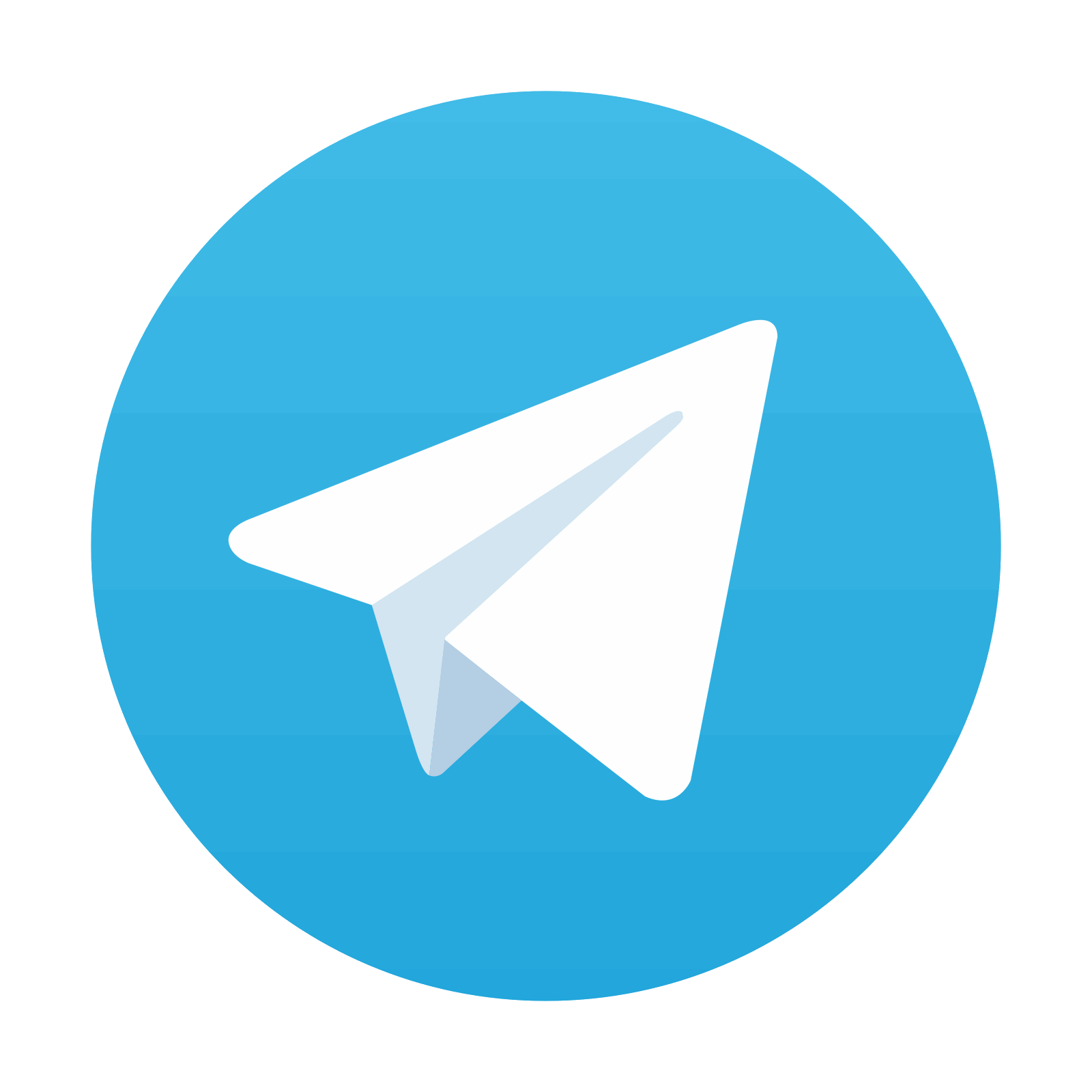
Stay updated, free articles. Join our Telegram channel

Full access? Get Clinical Tree
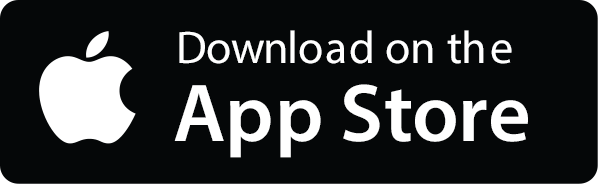
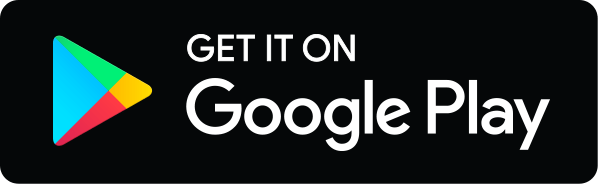