Hip/Proximal Femur
- Alan Rogers
- Joseph S. Yu
The hip is a common site of trauma and when injured can have a significant impact on the patient’s well-being. Worldwide the total number of hip fractures is expected to exceed 6 million by the year 2050. In 2003 over 300,000 patients were hospitalized with hip fractures in the United States, accounting for 30% of all hospitalized patients according to data from the U.S. Agency for Healthcare Research and Quality. The cost of treatment of hip fractures is estimated at $10 billion to $15 billion per year in this country alone.
The hip is a large spheroidal (ball and socket) synovial joint. The relationships between the acetabulum, femoral head, labrum, and joint capsule make the hip an extremely stable joint. The acetabulum is formed at the union of the ilium, ischium, and pubic bones and is partially covered by a horseshoe-shaped lining of hyaline cartilage. The acetabular labrum is a fibrocartilaginous structure that is firmly adhered to the acetabular rim and transverse ligament that serves to effectively deepen the socket, increasing the coverage of the femoral head and adding stability. The synovial-lined capsule surrounds the hip joint with focal condensations forming the iliofemoral, pubofemoral, and ischiofemoral ligaments providing further stability to the joint.
The femoral head is a partial sphere articulating with the acetabulum. It is largely covered by cartilage except for a central depression termed the fovea capitis . The ligamentum teres attaches to the femoral head at the fovea and attaches to the transverse ligament and margins of the acetabular notch. The foveal artery courses through the ligamentum teres, providing a small contribution to the vascular supply of the femoral head. The main vascular supply to the femoral head arises from an extracapsular vascular ring around the base of the femoral neck. The greater and lesser trochanters serve as attachment sites for the gluteus medius and minimus muscles and iliopsoas, respectively.
Acetabular fractures are discussed in Chapter 16 . Fractures of the proximal femur can be broadly categorized as intracapsular—those involving the femoral head or neck—or extracapsular—those involving the greater or lesser trochanters, the intertrochanteric or subtrochanteric regions. Intracapsular fractures are more susceptible to complications of nonunion, malunion, or avascular necrosis (AVN) of the femoral head due to the tenuous nature of the blood supply.
Bone and Joint
Intracapsular Fractures
Femoral Head Fractures
Fractures of the femoral head are relatively uncommon but often are associated with a poor functional outcome. They are typically seen in the setting of high-velocity trauma that results in a hip dislocation, such as a motor vehicle accident. In a dashboard injury the hip is vulnerable to dislocation due to its flexed and adducted position. The fracture occurs either from impaction or shearing. Reportedly, femoral head fractures occur in 7% to 15% of posterior hip dislocation injuries and as high as 68% of anterior hip dislocations. Associated complications include injuries to the sciatic nerve, AVN of the femoral head, periarticular heterotopic ossification, and secondary osteoarthritis.
Although several classifications exist, the Pipkin classification, which is based on the location of the femoral head fracture and the presence of associated acetabular or femoral neck fractures, is most widely used. A type I fracture involves the femoral head inferior to the fovea capitis, and type II fractures involve the femoral head superior to the fovea capitis. A type III fracture is a type I or II injury associated with a fracture of the femoral neck. A type IV fracture is a type I or II fracture with an associated acetabular fracture ( Fig. 15-1 ). The Pipkin scheme does not differentiate the type of hip dislocation injury. A more comprehensive classification that includes anterior and central hip dislocations has been proposed by Brumback and colleagues. This scheme divides fractures into one of five categories with A and B subsets incorporating the position of the femoral head with respect to the acetabulum ( Fig. 15-2 ).
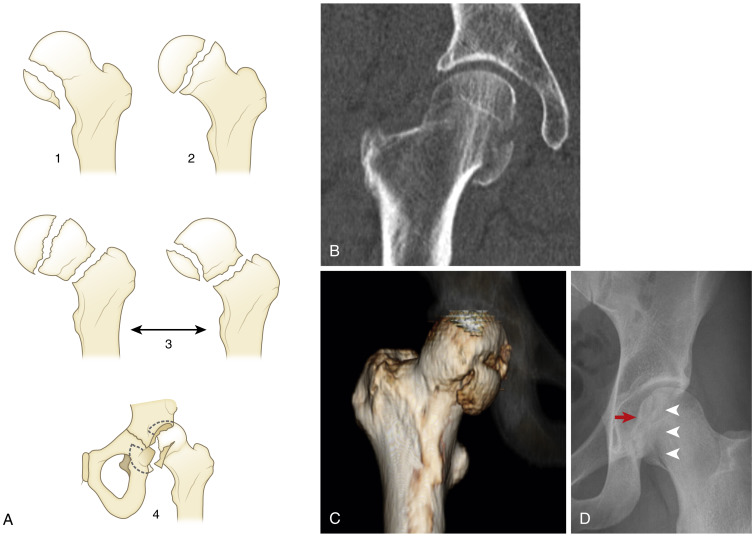
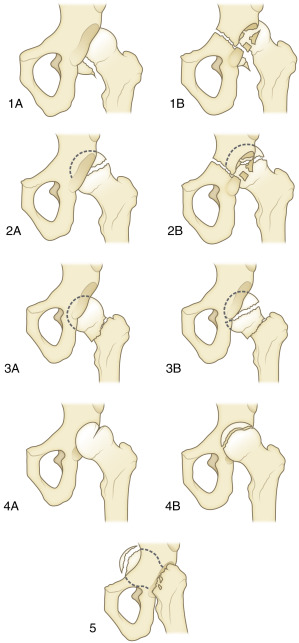
Radiographically an alteration in the normal contour of the femoral head denotes a femoral head fracture (but this may require computed tomography [CT] for diagnosis). Any fracture that creates a step-off greater than 2mm constitutes a surgical lesion. It is also essential to evaluate for a concurrent femoral neck fracture. Additional observations on CT that may be seen in patients with a femoral head fracture include the presence of intra-articular fragments, congruency of the articular surfaces, and the integrity of the acetabulum.
Femoral Neck Fractures
Femoral neck fractures occur most commonly in older adults. Several theories related to the mechanism of these fractures have been postulated, including direct trauma to bone that is weakened by osteoporosis, rotational forces incurred on the femoral neck at the time of a fall, or the completion of a fatigue fracture that subsequently causes a fall. Femoral neck fractures in patients under 50 years of age are relatively rare and are typically the result of high-energy trauma such as a motor vehicle accident or fall from height. Most femoral neck fractures occur just below the femoral head at the junction of the femoral head and neck (subcapital), although fractures may also occur through the midportion of the femoral neck (transcervical) or at the base of the femoral neck (basicervical). The medial and lateral femoral circumflex arteries supply 75% of the blood to the femoral head by way of the medial and lateral epiphyseal arteries and the inferior metaphyseal artery. Additional blood supply comes from the obturator internus artery and the artery of the ligamentum teres. The circumflex vessels may be disrupted when femoral neck fractures become displaced, resulting in AVN of the femoral head.
Evaluation of a patient suspected of having a hip fracture begins with radiographs. Proper technique is critical to accurately detecting femoral neck fractures. Anteroposterior radiographs of the pelvis should be performed with the hip in internal rotation when possible. Oblique frog-leg or cross-table lateral views are useful for delineating displacement of a fracture. As in pelvis fractures, it is useful to compare symmetry with the contralateral hip. When radiographs are negative or inconclusive for a fracture but there remains high clinical suspicion (i.e., unwillingness to bear weight), then magnetic resonance imaging (MRI) is the modality of choice for further study. The reported sensitivity and specificity of MRI for detection of radiographically occult fractures is nearly 100%, and it also has the added benefit of surveying the surrounding soft tissues for additional injuries.
Subcapital femoral neck fractures may be complete or incomplete. In addition, complete fractures may be impacted or displaced. There are numerous available classification systems for describing subcapital fractures, but the Garden classification, which consists of four stages, is perhaps the most recognizable. A stage I fracture is an incomplete (or occasionally complete) fracture with lateral impaction resulting in a valgus angulation. A type II fracture is a complete nondisplaced fracture that is impacted along the entire length of the fracture. A type III fracture is a complete fracture with partial displacement, usually with varus angulation. A type IV fracture is a completely displaced fracture with displaced fragments caused by proximal migration of the femoral shaft ( Fig. 15-3 ). Subcapital fractures may be extremely subtle, and a combination of a sclerotic line caused by trabecular overlap, cortical buckling or irregularity, and trabecular angulation should suggest this diagnosis.
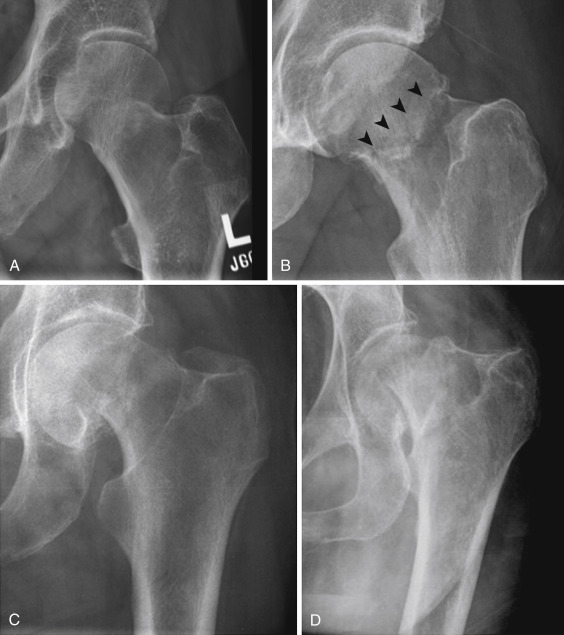
True transcervical femoral neck fractures are rare and are treated in the same way as patients with subcapital fractures. Basicervical fractures occur at the base of the femoral neck at its junction with the trochanters ( Fig. 15-4 ). When a basicervical fracture occurs, the possibility of a completed stress fracture or a pathologic fracture should be considered.
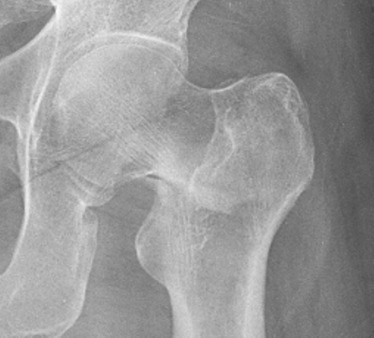
There are two types of stress fractures, fatigue and insufficiency. Fatigue stress fractures result from abnormally increased stress placed on normal bone, which occurs frequently in athletes and military recruits. Insufficiency stress fractures are the result of normal stress placed on abnormal bone occurring, for instance, in the settings of osteoporosis, renal osteodystrophy, or chronic steroid use. In the femoral neck, stress fractures may affect the compressive side or the tensile side. The majority of stress fractures are compressive, involving the inferomedial cortex of the femoral neck. Radiographically the appearance of these fractures is a linear area of sclerosis perpendicular to the cortex with or without an associated linear lucency through the cortex. Surrounding endosteal sclerosis and periosteal reaction may be observed as well ( Fig. 15-5 ). Tensile stress fractures typically are insufficiency type of fractures occurring in the superolateral cortex of the femoral neck just inferior to the femoral head. The characteristic radiographic appearance is an ill-defined linear area of sclerosis or simply a break in the cortex ( Fig. 15-6 ). These fractures may progress to a complete subcapital fracture if left untreated.
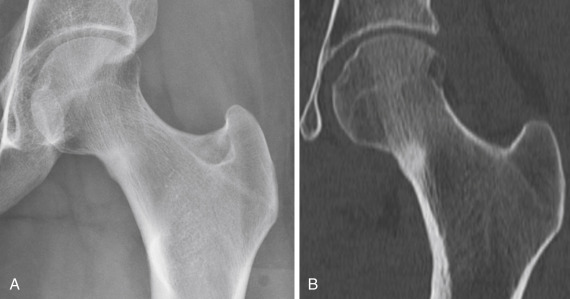
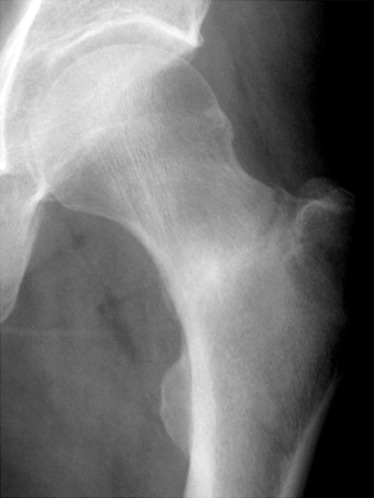
Extracapsular Fractures
Trochanteric and Intertrochanteric Fractures
Intertrochanteric fractures are common in older adults. Isolated trochanteric fractures are less common and can affect people in any age-group. Isolated greater trochanteric fractures are often the result of a fall or direct trauma ( Fig. 15-7 ). They may be difficult to detect radiographically, particularly in osteoporotic or thin patients. The treatment of greater trochanteric fractures is conservative unless the greater trochanter is severely displaced. It is important to characterize the fracture as not part of a more extensive intertrochanteric fracture. If the radiographic findings are equivocal, MRI is recommended to accurately define the extent of the fracture. In skeletally immature patients, avulsion fractures of the greater trochanter may occur owing to sudden forceful contraction of the gluteal muscles on the apophysis. These are treated conservatively if displacement is less than 1 cm and displacement does not increase on sequential follow-up radiographs. Isolated fractures of the lesser trochanter are usually the result of tension by the iliopsoas tendon on the enthesis and characteristically occur in children or adolescent athletes. In skeletally mature people, isolated lesser trochanteric fractures often are pathologic fractures, indicative of an underlying bone neoplasm or metastasis ( Fig. 15-8 ).
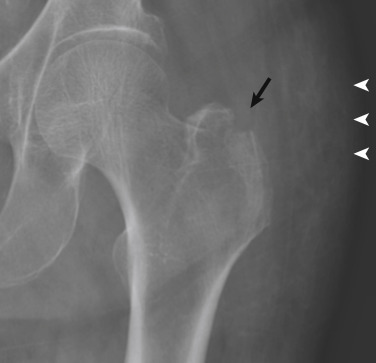
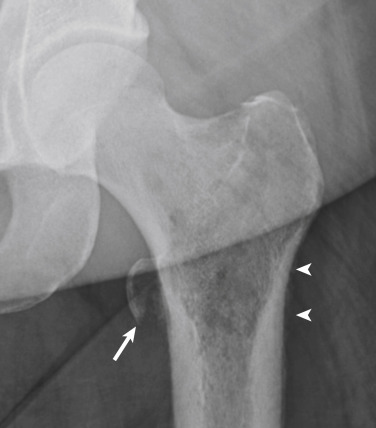
Intertrochanteric fractures extend from the superolateral aspect of the greater trochanter to the inferomedial aspect of the lesser trochanter along the intertrochanteric ridge. Comminution is common, and as many as 50% are considered unstable. The integrity of the calcar femorale, posterior and medial cortices, number of fragments, and displacement determine stability.
There are several classifications available for characterizing intertrochanteric fractures. A common method employed is the Evans classification, which subdivides intertrochanteric fractures into six types ( Fig. 15-9 ). A type I is a simple two-part nondisplaced fracture that parallels the intertrochanteric line. A type II is a two-part fracture that is displaced. Both of these are considered stable. A type III fracture is a three-part fracture with displacement of the greater trochanter, resulting in loss of posterolateral support. A type IV fracture is also a three-part fracture but with displacement of the lesser trochanter, resulting in loss of medial support. Both type III and type IV Evans fractures are considered unstable and difficult to reduce. A type V fracture is a four-part fracture with displacement of both the greater and lesser trochanters. The final type is termed R and denotes a fracture with reversed obliquity, with the fracture line beginning in the lesser trochanter medially and extending inferolaterally to exit below the lesser trochanter. These fractures are inherently unstable due to the unopposed traction of the abductors on the greater trochanter and adductors on the lesser trochanter and femoral shaft. It is noteworthy, however, that these fractures may be nondisplaced at presentation ( Fig. 15-10 ).
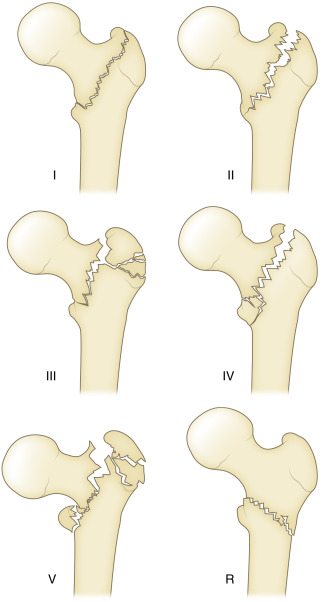
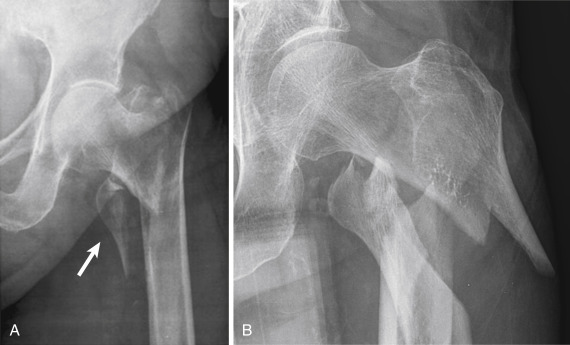
Subtrochanteric Fractures
Subtrochanteric fractures occur between the lesser trochanter and the proximal 5 to 7 cm of the femoral shaft and account for about 10% to 34% of all hip fractures. These fractures have a bimodal age distribution. In younger patients they are typically the result of high-energy trauma resulting in comminution and significant soft tissue injuries. In older patients the fractures are often the result of low-energy trauma, and diminished overall bone density is an important contributing factor. Radiographically, fractures in older adults tend to be linear or spiral and only minimally displaced.
Numerous classifications for subtrochanteric fractures have been proposed. The AO classification is a complicated three-part scheme with multiple subtypes often used in research. The Seinsheimer classification consists of eight subgroups based on stability of the medial cortex. The Russell-Taylor classification is useful in determining the mode of fixation required based on involvement of the piriformis fossa. Type I fractures do not involve the piriformis fossa. Type I fractures are subdivided into type IA, which occur below the lesser trochanter, and type IB, which involve the lesser trochanter. Type II fractures involve the piriformis fossa. Type IIA fractures demonstrate a stable medial cortex, and type IIB denotes involvement of the piriformis fossa with lack of stability of the medial femoral cortex. Management is determined by the extent of the fracture, with type I fractures being treated with intramedullary devices whereas type II fractures require open reduction and internal fixation ( Fig. 15-11 ). The Fielding classification divides subtrochanteric fractures into three zones. Zone 1 includes fractures that involve the lesser trochanter, zone 2 occurs 1 to 2 inches distal to the lesser trochanter, and zone 3 occurs 2 to 3 inches distal to the lesser trochanter ( Fig. 15-12 ).
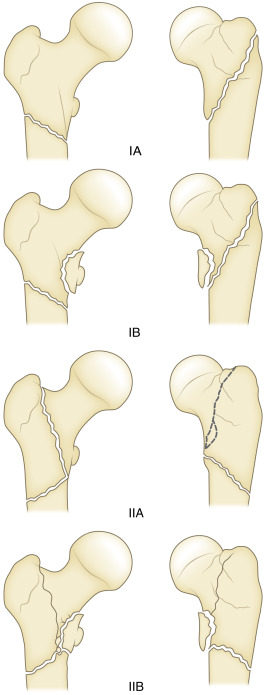
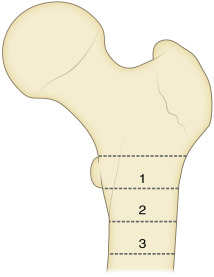
Hip Dislocation
Hip dislocations are severe injuries caused by high-energy trauma and account for 5% of all joint dislocations. Dislocation of the femoral head is dictated by the position of the lower extremity and hip at time of injury, as well as the vector or direction of force. There are three types—central, posterior and anterior—based on the terminal position of the dislocated femoral head.
Although assessment of hip dislocations begins with radiographs, CT is critical in the management of hip dislocations. Long-term disability may be caused by an injury to the sciatic nerve; AVN, which occurs in up to 17% of hip dislocations; and osteoarthritis. The risk for AVN increases proportionally with the length of time that the hip is dislocated. Osteoarthritis is the most common complication, occurring in 16% of dislocations without associated fractures and up to 80% when there is a concomitant acetabular fracture. Computed tomography is ideal for determining congruency of the joint after reduction, identifying other fractures of the acetabulum or femoral head, and detecting trapped intra-articular fracture fragments. The role for MRI is currently reserved for chronic or persistent pain that may be caused by underlying injuries to the ligamentum teres, acetabular labrum, or articular cartilage.
Central dislocations occur in patients with acetabular fractures that disrupt the quadrilateral plate, produced when a lateral force strikes an adducted femur. In this injury the femoral head displaces medially and the joint space may narrow medially, superiorly, or in both directions. Computed tomography is critical for preoperative planning because the acetabular fracture is often complex. Most surgeons advocate that CT be performed within the first 10 days of the injury for optimal results ( Fig. 15-13 ).
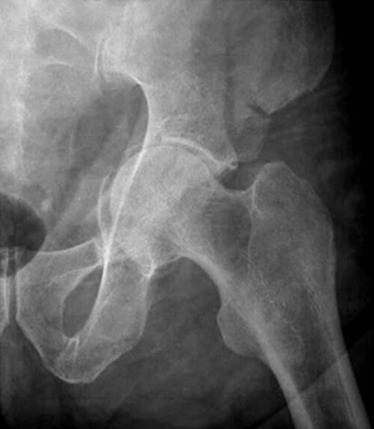
Posterior dislocations account for 80% to 85% of all hip dislocations. The most common mechanism of injury is an anterior-to-posterior force directed to the lower extremity with the hip held in flexion (i.e., a dashboard injury). In this dislocation the femoral head displaces superiorly to the acetabulum and the femur rotates internally ( Fig. 15-14 ). Associated fractures of the posterior wall of the acetabulum are common and should be evaluated with CT because fractures that affect greater than 60% of the articular surface are likely unstable ( Fig. 15-15 ). Fractures of the femoral head are relatively less common and are the result of impaction or a shearing when the femoral head strikes the posterior wall of the acetabulum. Note that posterior dislocations may also occur with fractures of the ipsilateral femoral shaft.
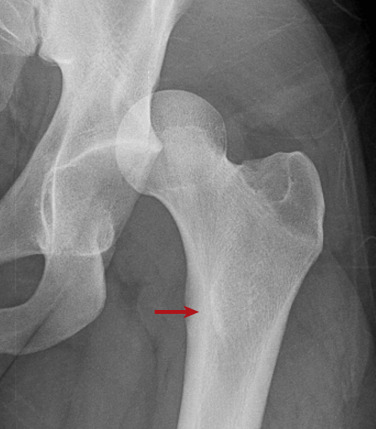
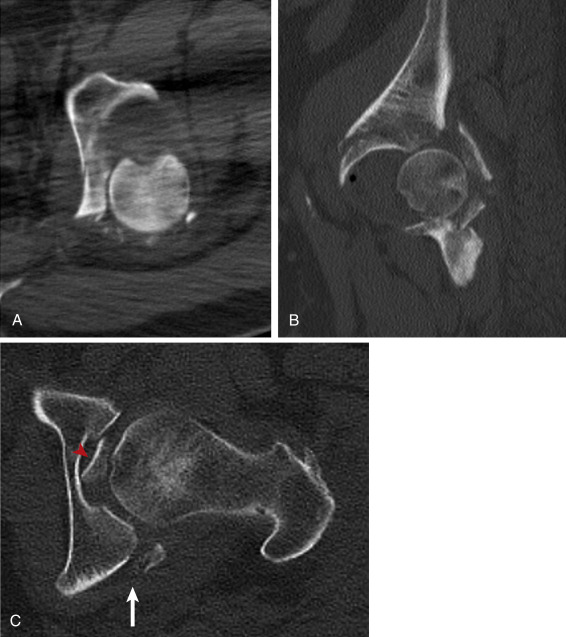
Anterior dislocations account for 10% to 15% of hip dislocation injuries. The dislocation occurs when a posteriorly directed force is applied to the inner aspect of the knee while the hip is abducted and externally rotated. There are two types, obturator and subspinous, and the degree of hip flexion determines the type of dislocation that will occur. An obturator dislocation is more common, with the femoral head displacing inferomedially while the femur abducts and externally rotates ( Fig. 15-16 ). In a subspinous dislocation the femoral head displaces superiorly beneath the spine of the iliac bone, and the femur extends and abducts. The terminal position of the femoral head, which is superior and lateral, may be confused for a posterior dislocation injury. The key to differentiating between these dislocations is the femoral position. In an anterior dislocation the femur is locked in external rotation, and the femur will also be abducted or neutral in position. In a posterior dislocation the femur is adducted and fixed in internal rotation ( Fig. 15-17 ).
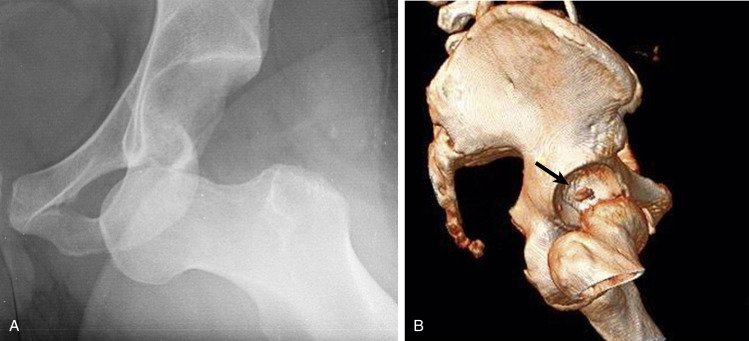
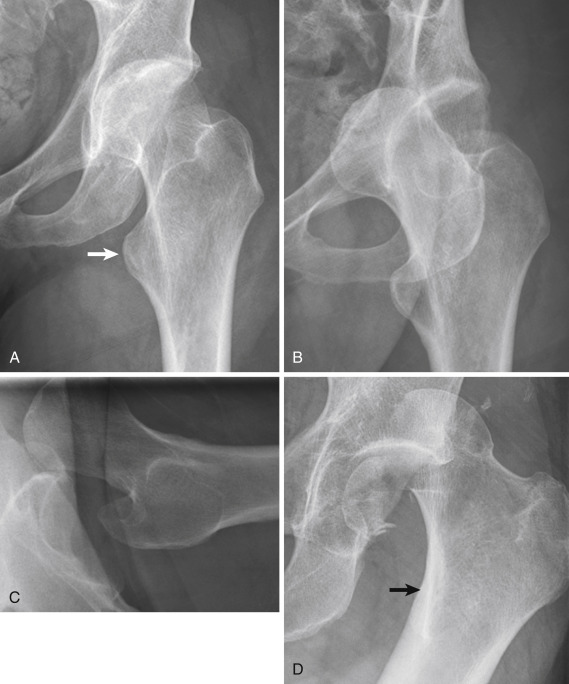
Septic Arthritis
Septic arthritis of the hip is more prevalent in children than adults. Adult populations at increased risk include intravenous drug abusers; patients with chronic liver disease or kidney disease, diabetes, or rheumatoid arthritis; and patients requiring long-term corticosteroid use. Patients with joint replacements are also at increased risk.
Radiographically, early findings tend to be relatively nonspecific, but as the infection progresses, the joint space narrows because of destruction of the cartilage. Periarticular osteopenia, marginal erosion, and osteolysis of the cortex are also important observations ( Fig. 15-18 ). Synovial inflammatory arthropathies share some similar findings, but rapidly progressive destructive changes should prompt an expeditious search for an infectious cause. Joint aspiration is useful in confirming the diagnosis and providing critical information regarding the source.
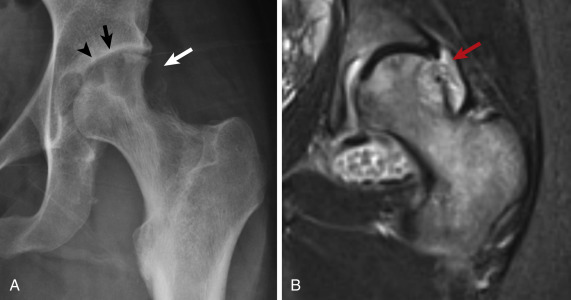
Soft Tissue Emergencies
Bursitis
A bursa is a fluid-filled sac lined with synovial cells located in areas of friction between tendons or against bony surfaces. Repetitive friction and overuse, infection, direct trauma, and arthritis can cause inflammation of various bursae, which then fill with fluid. Common presenting symptoms include regional pain and tenderness. Clinically bursitis may mimic joint-, tendon-, or muscle-related pain. The main bursae in the hip region are the iliopsoas, obturator externus, ischial, and trochanteric bursae. The iliopsoas bursa, located between the anterior hip capsule and the distal iliopsoas tendon, is the largest bursa in the hip. It communicates with the joint in 10% to 15% of people. The obturator externus bursa is located between the obturator externus and the ischium and may also communicate with the joint in up to 10% of people. A bursa exists between the greater trochanter and each of the gluteal tendons. The largest of these is the trochanteric or subgluteus maximus bursa located lateral and superior to the greater trochanter. The ischial bursa is situated between the hamstring tendons and the adjacent ischium.
Magnetic resonance imaging or ultrasonography is the preferred modality for evaluating bursitis because radiographs are usually normal. The sonographic appearance of a distended bursa is an anechoic fluid-filled structure lined by a hyperechoic wall. On MRI, bursitis characteristically appears as lobulated fluid-containing (T1 hypointense, T2 hyperintense) structure ( Fig. 15-19 ) that demonstrates a thin rim of peripheral enhancement after intravenous contrast administration. Enhancement extending beyond the bursa into the surrounding soft tissues is atypical and could indicate an infectious cause.
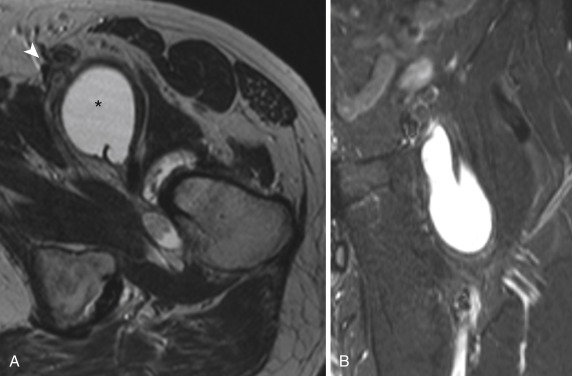
Tendon Injuries
Injuries of the proximal thigh musculature and/or tendons are painful conditions that may mimic other pathologic processes. The quadriceps, hamstrings, adductors, or gluteal muscles are frequent injury locations among young athletes. Most acute injuries are diagnosed clinically; however, imaging can be useful in cases in which the history and physical examination are inconsistent. Radiographs can confirm pathologic conditions in the bones such as stress fractures, neoplasia, or avulsion injuries. Ultrasonography provides a way to dynamically evaluate the muscles and tendons and is useful in detecting intramuscular hematomas. Avulsion occurs in predictable locations in the pelvis ( Fig. 15-20 ). Computed tomography is particularly useful for avulsion fractures that are radiographically occult. Magnetic resonance imaging is both sensitive and specific for diagnosis of muscular and tendinous injuries due to superior spatial resolution and optimal contrast between soft tissues. Contusions, hematomas, and partial or complete tendon ruptures are readily characterized as well.
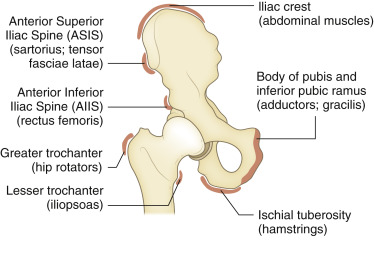
The severity of tendon injury can be ascertained on MRI. Generally a grade 1 injury demonstrates an intact tendon with interstitial edema and hemorrhage at the injury site characterized by high-signal intensity around the tendon on fluid-sensitive sequences. There may be a feathery appearance in the adjacent muscle. A grade 2 injury is partial disruption of the tendon. A hematoma may be present in the acute setting. A grade 3 injury is a complete disruption of the tendon, which may be retracted because of muscle spasm. An avulsion fracture is characterized by edema at the enthesis of the tendon with a donor defect in the adjacent bone. Most soft tissue injuries are treated conservatively; however, complete tendon ruptures and osseous avulsions are indications for surgical intervention, particularly in active people and athletes. Surgical intervention may be required to evacuate a large hematoma in the muscle and to treat compartment syndrome. Avulsion of the hamstring tendon from the ischium is an important injury. It is caused by abrupt tensile forces that overload the enthesis or musculotendinous junction during eccentric contraction of the muscle. Important observations include the length of the tendon gap, presence of a hematoma, and perineural edema around the sciatic nerve ( Fig. 15-21 ).
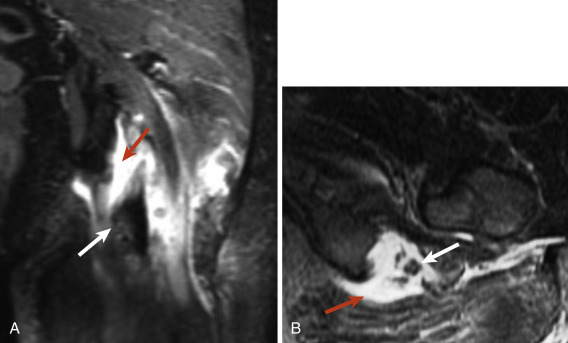
Knee
- David M. Melville
- Jon A. Jacobson
Knee radiography is the most common radiographic study performed for trauma in the emergency department, with nearly 75% of patients with acute knee injury undergoing evaluation with radiographs; however, radiographs obtained for acute knee trauma do not consistently depict all important injuries, and 25% of radiographic findings do not correlate with clinical presentation. Twisting injuries are responsible for three quarters of all knee injuries, but the majority of knee fractures result from blunt trauma. Knee radiographs should be carefully scrutinized for features suggestive of acute knee injury and further evaluation with cross-sectional imaging, including CT and MRI, should be recommended in cases with suggestive history or findings.
Knee Joint Effusion
Knee joint effusion may result from a variety of causes, including infection, inflammation, and trauma. The presence of a knee joint effusion should prompt a search for an underlying cause. Conventional radiographs may detect as little as 4 to 5 mL of fluid. An effusion may be detected radiographically by increased density in the suprapatellar recess located between the anterior suprapatellar and prefemoral fat pads on a lateral projection with slight knee flexion ( Fig. 15-22 ).
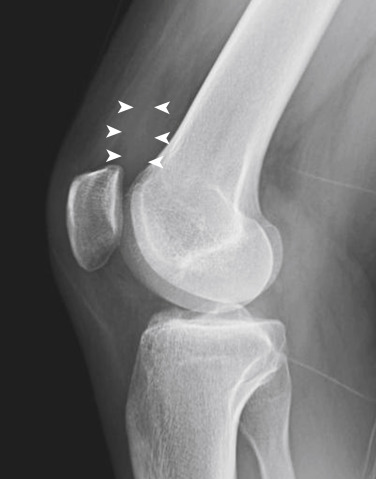
Posttraumatic hemarthrosis is considered an indicator of serious ligamentous injury, but there are no typical radiographic features to distinguish acute hemorrhage from simple knee joint effusion. The time course of effusion development may suggest cause because an effusion developing within the first several hours following a traumatic event is typically related to hemarthrosis, whereas reactive effusions appear 12 to 24 hours following injury. The presence of fat within a joint effusion, termed lipohemarthrosis , is a reliable indicator of intra-articular fracture because it represents the sequelae of cortical disruption and extrusion of marrow fat, although occasionally it may also be seen with cartilage, ligamentous, or synovial injury in the absence of fracture ( Fig. 15-23 ).
