183CHAPTER 12
Lung SBRT
“Stereotactic radiosurgery (SRS), a term originally coined by Dr. Lars Leksell at Karolinska Hospital in Stockholm, Sweden, is an approach to radiation therapy using multiple convergent beams, precise localization with a stereotactic coordinate system, rigid immobilization, and single-fraction treatment (1). In 1994, Karolinska Hospital was also the site of a paradigm shift in external beam radiation therapy (EBRT), when Lax, Blomgren, and colleagues published the first report of extracranial SRS (2, 3). Extracranial SRS is also referred to as stereotactic body radiation therapy (SBRT) or stereotactic ablative radiation therapy (SABR), and these terms are used interchangeably in this chapter. The application of SRS/SBRT/SABR to tumors in the thorax may offer an alternative to surgery in the management of patients with lung neoplasm (4–8).
Lung cancer is the most common cancer with nearly 2 million cases a year globally, and is the leading cause of death by cancer (9, 10). Surgical resection is considered the standard treatment of early stage non–small-cell lung cancer (NSCLC). However, patients with early stage NSCLC who are medically inoperable or unwilling to undergo surgery are not uncommon. These patients were historically treated with conventional EBRT with high rates of local progression (11). SBRT is an alternative in these patients (4). Because of the encouraging results of SBRT in medically inoperable patients with NSCLC, SBRT has also been applied in some operable patients with early stage NSCLC, patients with locally advanced NSCLC, patients with recurrent NSCLC, and patients with pulmonary oligometastatic disease (7, 8, 12–15).
Over time, SBRT has benefitted from the development of technology including dedicated accelerators and integrated motion management solutions (CyberKnife®, Vero®, Novalis®, TrueBeam®, Versa HD®, etc.). New technology brings new questions, and SBRT exemplifies this. SBRT presents specific concerns compared with conventional EBRT, such as a very high biological effective dose (BED) and toxicity. Other details of treatment, such as delineation of target volume, dose, fractionation, treatment planning, and motion management, are still in debate, and a recent report published by the International Commission on Radiation Units and Measurements (ICRU; ICRU Report 91) should help both physicists and physicians to compare studies and results (16). When evaluating the results of SBRT treatment presented in this chapter, the reader should keep in mind that dosimetric characteristics, such as radiation dose, fractionation schedule, dose calculation algorithms, and isodose, present large discrepancies. The ICRU 91 report should help simplify the comparisons of results from different studies moving forward. Finally, patients treated with SBRT present uncommon toxicities (sometimes fatal), and new image interpretation recommendations during follow-up are needed (17).
SRS/SBRT/SABR treatment of tumors in the thorax may offer an alternative in the management of patients with lung neoplasm (18–21). SBRT is attractive for many reasons. It is noninvasive and painless, has a short overall treatment duration, and facilitates an immediate return to activities. 184In this chapter, we review the potential indications for SBRT, patient selection, and results of SBRT treatment of lung neoplasms including primary NSCLC, recurrent lung cancer, persistent cancer in a previously irradiated field, and pulmonary metastases. In addition, we discuss the technical details of SBRT including motion management, dose fractionation and schema, results of treatment, quality of life (QOL), and follow-up imaging protocols.
INDICATIONS AND RESULTS
Lung SBRT may be indicated for several different presentations of lung cancer including NSCLC in medically inoperable patients, persistent tumors requiring reirradiation, recurrent tumors, and lung metastases.
Early Stage NSCLC
Medically-Inoperable Early Stage NSCLC
Surgical resection is the standard for operable patients with early stage NSCLC. SBRT is recommended only in patients with medically inoperable early stage NSCLC. Although surgery is considered a gold standard for early stage NSCLC (25% of patients), at about 20% of this population is unfit or unwilling to undergo surgery (22). This is especially observed in elderly patients, as well as patients with comorbidities such as chronic obstructive pulmonary disease (COPD). In medically inoperable patients, EBRT was considered the standard of care before the era of SBRT and other ablative modalities such as radiofrequency ablation. However, with poor outcomes noted with EBRT alone (11), SBRT has become a standard treatment in this patient population. Palma et al. demonstrated that SBRT led to a 12% decrease in the number of elderly patients with early stage NSCLC who go untreated with an accompanying improvement in overall survival (23).
Early results of SRS/SBRT/SABR were published from retrospective cohorts, yielding important findings that impacted clinical practice (7, 8, 18, 19, 24, 25). We presented our initial experience with SRS/SBRT for the treatment of lung neoplasm at the University of Pittsburgh Medical Center (UPMC) Hillman Cancer Center in 2005 (18). We treated 32 patients (27 with NSCLC and five with pulmonary metastases) with SRS using the CyberKnife system (18). Patients were treated with 20 Gy in a single fraction in this early series. Following SRS, an initial complete response was seen in seven patients (22%), partial response in 10 patients (31%), stable disease in nine patients (28%), and progression in five patients (16%). The probability of 1-year overall survival for the entire group was 78% (95% confidence interval [CI], 65%–94%), and the probability of 1-year overall survival for the patients with stage I NSCLC was 91% (95% CI, 75%–100%). This and other early studies demonstrated the safety and feasibility of SRS for the treatment of lung neoplasm.
Subsequently, we reported the results in 21 medically inoperable patients with stage I NSCLC treated with SRS at UPMC (19). With mean follow-up of 24 months, estimated 1-year survival probability was 81% (68% CI, 73%–90%). However, with a low dose of 20 Gy, local progression occurred in nine patients (42%). We concluded from this preliminary experience that SRS (median dose 20 Gy) is safe in this high-risk group of patients; however, it was associated with significant local progression. We and other investigators initiated prospective studies using SRS in multiple fractions and increased the BED to evaluate the efficacy of SBRT in high-risk patients with early stage NSCLC.
Timmerman et al. conducted a dose escalation study from 24 to 60 Gy in three fractions in 37 patients with stage I NSCLC treated with SBRT (5). At a median follow-up of 15 months, six patients had local failure; all had received less than 18 Gy per fraction. The disease-free and overall survival at median follow-up of 15 months were 50% and 64%, respectively. Onishi et al., in an analysis of outcomes in 245 patients with stage I NSCLC from 13 Japanese institutions, showed that increasing the BED was associated with improved local control (7). These authors reported a lower local recurrence rate when a BED more than 100 Gy was used. Although an increased dose delivered during SRS may improve local control, increased dose is also associated with increased toxicity, when treating central lesions (6). Therefore, dose modifications and modified treatment schema are required for central lesions, and this is described further, later in this chapter.
Investigators from UPMC led a prospective multicenter study to evaluate CyberKnife SRS for the treatment of stage I NSCLC in medically inoperable patients. Patients were evaluated by thoracic surgeons and radiation oncologists and accrued to the study from 14 centers. Patients with peripheral lesions were treated with a high BED, which was delivered in three fractions (total 60 Gy). We reported the preliminary results from the peripherally located tumor arm of our study 185at the American Society for Therapeutic Radiation and Oncology (ASTRO) annual meeting in 2012 (26). We enrolled a total of 78 patients with peripheral NSCLC; 62 with stage IA NSCLC and 16 with stage IB NSCLC underwent SRS. There was no peritreatment mortality. During follow-up, there were 41 deaths, 15 because of cancer. The median follow-up for patients was 26 months. The probability of 2-year cancer-specific survival for the entire group was 79% (95% CI, 69%–90%). During follow-up, 26 patients had recurrence. In three patients (3.8%) recurrence was local only, and 23 patients (29%) had regional and/or distant progression. We concluded that the intermediate-term results of this prospective multicenter study of SRS for high-risk, medically inoperable patients with stage I peripheral NSCLC were promising. In particular, local control and disease-specific survival were encouraging.
One of the first prospective studies of SBRT for early stage NSCLC was the Radiation Therapy Oncology Group (RTOG) 0236 trial, a phase II study in which 55 patients were treated with SBRT for a total dose of 60 Gy in three fractions (27). This study demonstrated a 3-year actuarial overall survival of 56% associated with an early local control of 98% at 3 years. Similar results were obtained in other studies (28).
Operable Early Stage NSCLC
In patients with operable early stage NSCLC, surgical resection is the standard treatment and the debate is still open concerning the role of SBRT in this patient population. Although there are no completed randomized controlled trials comparing surgery with SBRT in operable patients with early stage NSCLC, SBRT results in some studies have seemed promising (7, 29).
Three phase III studies comparing surgery with SBRT for early stage NSCLC were opened for enrollment (RTOG1201/American College of Surgeons Oncology Group [ACOSOG] Z4099, ROSEL, and STARS trials); however, all three studies failed to accrue well and were closed. Chang et al. published an analysis of the data from the limited number of accrued patients to the STARS and ROSEL trials (29). This analysis of 58 patients did not show a statistically significant difference in local control between the two treatments. However, the study has several limitations including low accrual and has been criticized (30).
Onishi et al. (7) reviewed a Japanese multi-institutional SBRT study that included 93 operable patients who refused surgery and were treated with SBRT. These authors stratified the results on the basis of the dose of radiation and the BED delivered. They reported that 5-year overall survival was 88.4% for patients treated with BED more than 100 Gy and 69.4% for patients treated with BED less than 100 Gy. Multi-institutional phase II studies of SRS in the treatment of patients with potentially resectable stage I NSCLC have also been done in Japan (JCOG0403). There have been several retrospective studies using matched-pair analyses comparing surgery with SBRT; however, it is difficult to derive definitive conclusions because of the limitations of the retrospective nature of these studies, including selection bias (31). Palma et al. explored a new treatment strategy combining neoadjuvant SBRT and surgery for medically operable patients with early stage NSCLC, but only very early results are published with no postoperative mortality at 90 days (32).
Although surgical resection with lobectomy is the standard treatment for early stage NSCLC, some patients with early stage NSCLC who are not candidates for a lobectomy because of medical comorbidities are treated with a sublobar resection. Further studies are needed and are currently underway comparing sublobar resection with SBRT for stage I NSCLC. In 2016, there were at least four prospective studies comparing surgery with SABR: SABRTooTH (UK National Health System), POSTLIV (China), STABLE-MATES (University of Texas Southwestern Medical Center), and VALOR (U.S. Veterans Affairs). The results of these trials are eagerly awaited before recommendations can be made for the role of SBRT in operable patients with NSCLC.
Prognostic Factors
Some studies have examined prognostic factors after treatment of patients with early stage NSCLC with SABR that have included tumor characteristics, dose, and imaging biomarkers, such as maximum standardized uptake value (SUVmax). Matsuo et al. in a study of 101 patients who underwent SBRT for NSCLC described the impact of tumor diameter for both local control (p = .020) and overall survival (p = .013) 3 years after SBRT (33). Shaverdian et al. described location as a prognostic factor; patients with lower lobe, stage I NSCLC tumors had poor relapse-free (p = .04) and overall (p = .01) survival (34). Takeda et al. (35) found that a higher SUVmax was a worse predictive factor for both overall survival and disease-free survival at 3 years among 186152 patients treated with SBRT. Similar results were later confirmed by Horne et al. (36). Some authors have reported preliminary results on the prognostic value of radiomics and textural-features analysis, but further studies are warranted (37, 38).
Advanced NSCLC
For advanced inoperable NSCLC, the standard of care is chemoradiation; however, up to 50% of patients develop local (in-field) failure, so SBRT has been explored for advanced NSCLC using a few different approaches. For large tumors, Eriguchi et al. (39), in a retrospective study with 25 patients treated exclusively by SBRT, reported 2-year local control rates for T3N0 and T4N0 NSCLCs of 91% and 68%, respectively, with a low toxicity rate. This study should be regarded as a feasibility study and needs to be compared with results from Cho et al. who reported outcomes for patients with cT1-3N0 NSCLC treated with radiation therapy alone and a classical fractionation schedule (40). Some studies have reported preliminary results of SBRT treatment of patients with tumors involving the chest wall. Berriochoa et al. retrospectively reviewed 13 patients with chest wall involvement treated with SBRT with no grade 3 toxicity and a 1-year local control rate of 89% (41). In addition, some authors have explored the possibility of SBRT as a boost to both the primary tumor and the involved nodal area after conventional chemoradiotherapy. Hepel et al. (42) explored SBRT as a boost after concurrent chemoradiation for locally advanced NSCLC and reported 1-year local control of 78%. Given the limited data available, additional evaluation is warranted before consensus statements regarding the role of SBRT in advanced NSCLC will be forthcoming from ASTRO, the European Society for Radiotherapy and Oncology (ESTRO), the National Comprehensive Cancer Network (NCCN), and the European Society for Medical Oncology (ESMO).
Others Indications for SBRT in the Lung
Oligometastases
Hellman and Weichselbaum described the concept of oligometastases as a distinct intermediate between locally advanced cancer and widely disseminated metastases (13). Classic studies investigating lung cancer, including some nearly two decades ago, have documented long-term survival of selected patients who presented during follow-up with limited recurrent metastases and were treated with complete surgical resection or radiation (43). In addition, resection or radiation is applicable in patients with limited pulmonary metastases from other sites. In a study of outcomes in 5,206 patients using the International Registry for Lung Metastases (IRLM), the estimated 5-year survival after complete resection was 36%, and the median survival was 35 months (44). SBRT has also been used to treat patients with pulmonary metastases and recurrent lung cancer (8, 14, 15). Le et al. (45) reported the results of single-fraction radiosurgical treatment in 12 patients with metastatic or recurrent lung neoplasm. The 1-year overall survival was 56% in patients with pulmonary metastases. We previously published the results of 100 consecutive patients who were treated with SABR at UPMC (8). Nineteen of these patients had pulmonary metastases, with colorectal cancer being the most frequent primary site. The median overall survival was not reached (95% CI, 21 months—not reached) for patients with metastatic disease. The estimated 2-year overall survival by cancer type for metastatic disease was 84% (95% CI, 47%–96%).
Another retrospective study comparing surgery with SBRT, published by Widder et al., did not report significant differences in either local control (85% for surgery vs. 83% for SBRT at 4 years) or overall survival (49% for surgery vs. 41% for SBRT at 5 years; 46). Franceschini et al. analyzed the outcome of 200 patients with colorectal cancer, sarcoma, or renal cell carcinoma with lung metastases treated by SBRT. The time interval between the treatment of the primary and the development of metastases, the performance status, histologic type, number of metastases, and size were all prognostic factors (47).
SBRT is recommended as an option by the NCCN and ESMO depending on patient and clinical history characteristics. Further studies are required to fully evaluate the role of SBRT for pulmonary metastatic disease.
Treatment of Recurrent or Persistent Lung Cancer
Recurrent Disease
After complete resection or treatment of primary NSCLC with definitive chemoradiation, a significant proportion of patients develop recurrent disease, which is associated with cancer-related mortality (48, 49). Innovative treatment options 187are essential in this patient population. We published the results of 100 patients with recurrent or persistent lung cancer who were then treated with SRS/SBRT/SABR at UPMC (14). The median interval from the treatment of the primary tumor (with surgery or chemoradiation) to the treatment of recurrent or progressive disease was 18 months. SRS was administered to treat the first recurrence in the majority of patients (70%). For patients who had received prior radiation, the median dose was 48 Gy. The primary end point we evaluated was overall survival. There was no mortality within 30 days after treatment, and morbidity was minimal. The estimated 2-year overall survival was 49% (95% CI, 40%–60%), and 5-year overall survival was 31% (95% CI, 23%–43%). We also examined the joint effects of covariates with a proportional hazards regression model. The regression model identified three significant factors that adversely affected survival: larger tumor size (p < .0001), whether SRS was applied to a second or subsequent recurrence (p = .0014), and being male (p = .0128). Hou et al. (50) reported the outcomes of SRS in 50 patients with recurrent disease who had undergone a prior lung resection, and reported a local control of 100% within 1 year, but this was associated with significant toxicity (12% of patients experienced toxicity ≥grade 2).
Persistent Disease After Prior Radiation or Chemotherapy
When lung cancer persists after radiation treatment, reirradiation of the field poses challenges and requires special caution because high toxicity rates and variable local control rates have been reported (40–70%; 51). However, median progression-free survival after SBRT reirradiation is nearly 1 year; therefore, patients may benefit from this strategy. Because data are limited by the small studies performed thus far, further studies are needed to determine patient selection criteria and treatment characteristics.
Recurrent or persistent disease after chemotherapy also presents a challenge to radiation oncologists. Acquired resistance because of epidermal growth factor receptor (EGFR) mutations can limit the utility of tyrosine kinase inhibitors (TKIs). Some patients present with oligoprogression, and some clinicians have explored the utility of combining SBRT with TKIs. The results of the UPMC series, discussed previously, included SBRT in patients who developed recurrent disease after prior chemotherapy or chemoradiation (14).
SRS/SBRT AND CONSIDERATION OF TUMOR CHARACTERISTICS
Tumor Location and Toxicity of Treatment
Tumor location, whether peripherally or centrally located in the lung, is an important factor in risk assessment for lung SABR, because SABR for peripheral lung lesions shows a lower toxicity profile. Toxicity of SABR to peripheral lesions includes the risk of chest wall toxicity and rib fractures. Apical lesions have specific toxicity profiles because of their location. Some authors have described an increased risk of brachial plexopathy with apical lesions (52).
SABR for centrally located lesions in the lung results in a higher toxicity (6). Some of the first investigations of SBRT for central lung lesions reported very high (even fatal) toxicity in some cases because of fistula, bronchial stricture, and massive hemoptysis, so initially high-dose SBRT was contraindicated for central tumors. In a phase II study of 70 medically inoperable patients with stage I NSCLC treated with SRS using 60 to 66 Gy in three fractions, local control of 95% and an estimated 2-year overall survival of 54% were reported (6). However, there was an increased toxicity noted in central tumors, with a 2-year freedom from severe toxicity of 54% in central lesions compared with 83% in peripheral lesions. During a median follow-up of 17.5 months, six of the 70 patients (8.6%) died of treatment-related causes. The authors concluded that this SABR regimen should not be used for patients with tumors near central airways. These investigators originally described a “no-fly zone” within 2 cm of a major or lobar bronchus as a contraindication to high-dose SBRT. The Advanced Radiation Technology Committee of the International Association for the Study of Lung Cancer (IASLC) further detailed that the zone within 2 cm in any direction of any mediastinal critical structure should be considered a forbidden zone, or “no-fly zone,” for SABR (53). Chang et al. cautioned that the delivery of high ablative doses of radiation for central lung lesions with exposure of critical structures, such as the bronchial tree, esophagus, major blood vessels, heart, and brachial plexus and phrenic nerve, can lead to severe toxicity. These authors suggested a modified dose schema, optimized planning, and normal tissue dose–volume constraints when treating centrally located lung lesions (53).
188Fortunately, advances in motion management and modifications in the dose schema have allowed treatment of centrally located lung lesions with lower toxicity rates. At UPMC, we typically use a dose of 48 Gy in four fractions for central lesions. We delineate the target with care to protect adjoining critical central structures. In addition, we typically avoid SBRT for endobronchial lesions visualized on bronchoscopy. Davis et al. (54) published a retrospective analysis of 111 patients with 114 centrally located lung tumors (48 T1-2N0M0- NSCLC and 66 lung metastases). SBRT dose varied from 16 to 60 Gy (median 48 Gy) and was delivered in one to five fractions. They found a low toxicity rate with no grade 3 or higher (acute or late) toxicity and 2-year overall survival of 79% for patients with T1 tumors, 32% for patients with T2 tumors, and 49% for patients with metastases. Other authors have also published their results of treatment of centrally located tumors (55). We are still waiting for the results from the phase I/II studies of the RTOG 0813 trial (ClinicalTrials.gov Identifier: NCT00750269) to determine the best dose for both tumor control and limiting toxicity in patients with centrally located lung tumors.
Histologically Unproven Nodule
When faced with a histologically unproven nodule, our approach at UPMC is to obtain a biopsy of the lung lesion, if possible, prior to SBRT treatment. In some patients, the risks of biopsy may be very high, or the results of the biopsy are not conclusive. Various algorithms have been described to estimate the probability of malignancy in a solitary lesion (56). These include not only lesion radiological characteristics but also patient characteristics such as age, history of smoking, and history of cancer (57). Except in rare circumstances where tissue confirmation is not possible, our standard approach at UPMC is to obtain a biopsy of the lung lesion in all patients prior to treatment.
Nodal Staging
Nodal metastasis is an important prognostic factor in NSCLC. The combination of CT scan and PET-CT scan has improved our evaluation of the nodal status. In medically inoperable patients with early stage NSCLC who are being considered for SBRT, patients with mediastinal lymph nodes less than or equal to 1 cm and without increased fluorodeoxyglucose (FDG) uptake in the mediastinal lymph nodes can be considered as node negative. However, it should be noted that PET-CT is not as accurate for nodal staging as invasive mediastinal staging. A prospective multi-institutional ACOSOG study evaluated the role of PET scanning in lung cancer (58). PET was significantly better than CT for the detection of N1 and N2/N3 disease (42% vs. 13%, and 58% vs. 32%, respectively). The negative predictive value of PET for mediastinal node disease was 87%. Distant metastatic disease was indicated in 19 of 287 patients (6.6%), but was subsequently shown to be benign. The authors concluded that PET should not be relied on as a sole staging modality for mediastinal staging, and positive findings should be confirmed by mediastinoscopy. Although mediastinoscopy is considered the standard, more recently, endobronchial ultrasound (EBUS)–guided biopsy has also been used for nodal staging.
Large Tumors
Few results are specifically available on the use of SBRT for very large lung tumors. Verma et al. (59) explored the outcomes of SBRT in 92 patients with NSCLC ranging in size from 5 to 7.5 cm and showed local control of 73% at 2 years. They reported 2-year disease-free and overall survival of 53% and 46%, respectively, which may be lower than seen with SBRT for smaller tumors. Ricco et al. (60) confirmed the negative impact of increasing lesion size in a large retrospective cohort of 577 patients treated for lung metastases with SBRT. A survey from the German Society of Radiation Oncology (Deutsche Gesellschaft für Radioonkologie [DEGRO]) placed a consensus limit on lung tumor size for SBRT at 4 to 5 cm with a protracted regimen for bigger tumors (61).
Lung Cancer With Multiple Primary Tumors
The incidence of multiple synchronous primary lung cancers can be as high as 8%. SBRT can be considered as feasible in some of these patients depending on their comorbidities, and the volume irradiated in patients with more than one target (62). Local control and toxicity ratings are excellent depending on the organ-at-risk (OAR) volume irradiated.
189PATIENT CHARACTERISTICS AND CONSIDERATION FOR SBRT
Patient characteristics must be taken into account when considering SBRT for NSCLC, particularly the patient’s age and any comorbidities. Advanced age has been associated with worse toxicity in some but not all studies. Hayash et al. compared the outcomes of SBRT among the elderly and found that octogenarians experience similar efficacy of SBRT, as indicated by local control, but with a higher rate grade 2 toxicity (63). Patients with significant COPD, with reduced forced expiratory volume (FEV) or reduced diffusing capacity of the lungs for carbon monoxide (DLCO), and who are medically inoperable are candidates for SBRT. Guckenberger et al. (64) found a limited loss of less than 10% of FEV1 or DLCO within 24 months of SBRT without clinical impact. However, Ueki et al. found that a history of active interstitial lung disease (ILD) is a significant prognostic factor for grade 5 toxicity after SBRT (65). Nevertheless, in patients with ILD who are unfit for other treatment strategies, SBRT may still be an option.
Concomitant Treatment or Adjuvant Treatment
None of the oncology societies recommend standard concomitant or adjuvant chemotherapy with SBRT (66). Verma et al. studied chemotherapy with concomitant SBRT for large tumors (≥5 cm) and observed an effect on the overall survival (p = .027), but overall survival was poor (23 months vs. 30 months depending on chemotherapy) (59). Iyengar et al. published a small, phase II trial with 24 patients who were treated by SBRT and erlotinib (67). Each of the patients had fewer than six metastases and had progressed through platinum-based chemotherapy. Treatments with SBRT and erlotinib were well tolerated and resulted in high progression-free survival (median 14.7 months) and overall survival (median 20.4 months).
TREATMENT PLANNING
Delineation of the Target Lesion
With CT scanners, the optimal threshold for target delineation of lung tumors is a “lung window”—window level of 600 Hounsfield units (HU) and a window width of plus or minus 1,600 HU. The optimal window for target delineation for mediastinal lesions is a window level of 20 HU and a window width of plus or minus 400 HU. Historically, only gross tumor volume (GTV) was delineated using CT without clinical target volume (CTV). Because tumors and atelectasis can be difficult to separate, Hanna et al. recommend using PET-CT with caution to improve accuracy and the reproducibility of delineation (68). Moreover, several authors, such as Fleckenstein et al. (69), explored the impact of MRI for lung delineation using T2-weighted images without reporting clinical outcomes.
Internal target volume (ITV) using four-dimensional CT is needed to delineate the target volume on the maximum inspiration, expiration, and the mean. This is then fused, verifying that lung tumors are still delineated on each phase (0%, 25%, 50%, 75%, and 100%). However, this delineation depends on the motion management strategy. Planning target volume (PTV) depends on the type of motion management and accelerator, and margins range from 1 to 5 mm, but no study has compared the impact of the PTV margin. A survey published by Redmond et al. found a consensus PTV margin of 5 mm (70). At the University of Pittsburgh, we use at least a 5-mm margin, with consideration for the critical adjacent structures, when planning the target volume. Figure 12.1 shows the delineation using CT, PET-CT, and MRI.
Dose and Fractionation for Target Volume and Organ at Risk
Because of heterogeneity in dose, fractionation schedule, target definition, motion management, and reporting, it is clear that results for physical characteristics should be taken with caution. The primary feature of SBRT is ablative doses of radiation through hypofractionation. With the limits of the linear quadratic model and because a single one-point BED will not be representative of the biological effect, the correlation between the BED and the local control using an α/β ratio has been studied (71). BED10 greater than 100 Gy causes standard damage but also prevents repopulation and induces specific vascular damage. In a large, multi-institutional retrospective study of 257 patients, Onishi et al. found a significant difference for 5-year local control depending on the BED10, with 70.8% versus 30.2% (p < .001) for a threshold of 100 Gy (25). Moreover, one meta-analysis published by Zhang et al. suggested that regimens exceeding a BED of 146 Gy are associated with inferior survival (72). Another limi-tation highlighted in this meta-analysis was that authors did not consider various dose calculation algorithms. With regard to SRS treatment of pulmonary metastases, Ricco et al. found a 3-year local control of 77.1% versus 45% (p = .001) using a BED10 greater than 100-Gy threshold (60).
190
FIGURE 12.1 Use of multimodality evaluation for GTV delineation. Upper left: CT scanner; upper right: PET-CT scanner; lower left: MRI; and lower right: final treatment planning.
GTV, gross tumor volume.
With more than 25 different protocols and isodose prescriptions, the best dose fractionation schedule is still unclear and needs further study. Thus, it is more common to prescribe between 80% and 100% isodose line that encompasses 95% of the target volume. No consensus or guidelines are well described. There have been a few studies evaluating the optimal number of fractions for lung SBRT. However, recommendations have been published by an ICRU committee: ICRU Report 91, Prescribing, Recording, and Reporting of Stereotactic Treatments With Small Photon Beam. A recent study suggests that multifraction SBRT could be superior to single fraction but this was not confirmed by the RTOG 0915 trial published in 2015 (73). In the RTOG 0915 trial, two different schedules (34 Gy in one fraction vs. 48 Gy in four consecutive daily fractions) were compared with no significant difference in tumor control (97% and 92.7%, respectively) (73). Alite et al. retrospectively reviewed 107 patients treated with SBRT and described better local control with nonconsecutive versus consecutive fractionation (p = .001), which confirms a currently used fractionation schedule of three times a week (74).
Many authors recommend a more protracted dose fractionation schedule in patients with a central tumor or a tumor adhered to the chest wall or diaphragm without high-level clinical evidence. This is based on studies reporting mild-to-moderate side effects as compared with the side effects previously reported using nonprotracted schedules. Recently, the ESTRO Advisory Committee on Radiation Onco-logy Practice (ACROP) consensus guideline on implementation and practice of SBRT for peripherally located early stage NSCLC was published (75). The UK SABR consortium (76) suggests using 54 Gy in three fractions as the standard fractionation, 55 to 60 Gy in five fractions if the volume touches or extends into the ribs or pleura, and 60 Gy in eight fractions if the OAR constraints cannot be met at three or five fractions. Table 12.1 shows common dose regimens and BED.
Dose constraints also need further study. Given that typical patients with NSCLC have poor respiratory function, it is clear that the risk of radiation pneumonitis and fibrosis as a result of the SABR should be minimized. The most common constraints for ipsilateral lung are a V20 10% or less and V12.5 less than 15%, principally on the basis of work by Timmerman et al. (27). They also described the impact of the V40 and mean lung dose as predictive for pneumonitis. The relationship between the dose and changes in pulmonary function is less well studied.
191
For chest pain and skin toxicity, many authors report different results, such as a V30 threshold of 70 cm3 of the chest wall volume for chest pain and 50 mL for skin toxicity but without confirmation by large-data analysis. A significant increase in the rate of rib fractures appeared for BED greater than 132 Gy (11% vs. 5%, p = .007) (77). In patients with pleural involvement, Kimsey et al. described a 10% risk level for grade 2 or more for D70 mL of 16.2 Gy in four fractions and 65.1 Gy for the 50% risk level. In a same way, they also described, using D2 mL, 10% and 50% risk levels for toxicity grade 2 or more that were 43 and 87.9 Gy, respectively, in four fractions (78). Brachial plexus and nerve injuries are uncommon, and only dosimetric studies for brachial plexopathy described a threshold with a BED3 more than 100 Gy. The main significant risk factor is the apical location of the tumor (52).
For other constraints, data are available only for central tumors, and no specific data are available for peripheral lung SBRT. A meta-analysis published by Senthi et al. (55) estimated a normal-tissue BED3 (α/β ratio of 3) threshold of 210 Gy for limiting toxicities and treatment-related mortality. Duijm et al. (79) described a normal-tissue complication probability (NTCP) model, with, for example, a risk of 50% to occlude segmental bronchi if 0.5 mL of tissue is irradiated with up to 50 Gy in five fractions. They also defined a 50% risk level of grade 1 side effects, for a five-fraction Dmax of 55 Gy midbronchi and 65 Gy for mainstem bronchi. Concerning the aorta and major vessels, Xue et al. (80), on the basis of RTOG 0813 and the findings of Timmerman, reported a maximum dose (Dmax) range from 45 Gy (three fractions) to 52.5 Gy (five fractions) for a grades 3 to 5 toxicity rate inferior to 2.5%, but other authors report a higher threshold of 120 Gy. Figure 12.2 shows an example of treatment planning. Table 12.2 shows the results from the survey published by Redmond et al. about OAR constraints (70).
Physics Specificities and Motion Management
Impact of Accelerators
Currently, many accelerators for SBRT exist with dosimetric and treatment duration differences; they have not been demonstrated to differ in clinical outcomes. However, the volume exposed to very low radiation doses is more important if intensity-modulated radiation therapy, helical tomotherapy, or volumetric-modulated arc therapy (VMAT) is used. Thanks to high-dose-rate, flattening, filter-free (FFF) radiation therapy, which allows a dose rate of up to 2,400 monitor units/min, treatment time progressively decreased without compromising treatment delivery (81). However, FFF VMAT could present an interplay effect without proven clinical impact (82). The development of the MRI–linac, coupling MRI imaging with a medical linear accelerator (83), seems promising, because it may be able to track more nonfiducial tumors. With only limited data and no clinical outcomes reported, further studies are needed.
192
FIGURE 12.2 Example of treatment planning using CyberKnife® (Accuray).
Image courtesy of Accuray Incorporated–©2018 Accuray Incorporated. All Rights Reserved.
193
Impact of Lung Characteristics
Tumor Heterogeneity
Lung SBRT is a challenge for medical physicists for two primary reasons: tissue heterogeneity and tumor motion. The management of electron density discrepancies may vary greatly. Correction for changes in electron transport or lateral scatter disequilibrium is essential in lung; however, only the type B dose algorithm or Monte Carlo algorithm takes it into account. Haedinger et al. (84) reported that absorbed dose at the lung–tumor interface was different depending on the dose calculation algorithm applied. In addition, the normalization of optimization techniques and reporting is important and needed to compare treatments. Lacornerie et al. (85) published a major study of the physics of lung SBRT. They suggest robust optimization using type A algorithms to target the PTV and then calculation using the type B algorithms and rescaling the prescription to the GTV median dose (85). This method was validated through a clinical retrospective comparative cohort of 205 patients who were treated by SBRT (86). Discrepancy in the dose calculation between the two algorithms was major in small peripheral targets and reflected that the type A algorithm does not faithfully reflect the dose received by the target volume, but this did not have any clinical impact. Song et al. reported the clinical outcomes for 47 patients treated by SBRT for lung tumors with planning using either Ray-Tracing (22 targets) or Monte Carlo (25 targets) algorithms. Although response rate was lower in the Ray-Tracing group (p = .008), no significant differences were found in local control or toxicity (87). These results may lead to studies evaluating the impact of reducing doses for protocol compliance using the Monte Carlo algorithm (88). Figure 12.3 shows the differences between Ray-Tracing and Monte Carlo algorithms. Some authors also report an important GTV shrinkage during SBRT, even in five fractions. This finding should lead to exploration of the potential for adaptive SBRT because of the modification of electron transport (89).
194
FIGURE 12.3 Differences between Ray-Tracing and Monte Carlo algorithms for an extreme case (GTV in red, PTV in blue, lung in light blue, Ray-Tracing in solid lines, Monte Carlo in dashed lines).
GTV, gross tumor volume; PTV, planning target volume.
Tumor Motion
Management of tumor motion is essential, as several authors have reported tumor motion of up to 1 cm for both the central and the peripheral lesion. There are a few frequently used approaches to account for lung tumor motion: large PTV, ITV, gating, tracking with or without fiducial markers, and ventilation techniques. Using a large PTV is the easiest technique. The second easiest approach is a simple four-dimensional CT scan with delineation of an ITV (Figure 12.4). However, this leads to an increase in the volume of the normal lung irradiated and should be recommended only for small tumors with little motion. In a same way, it is also possible to delineate only on a time-weighted average CT dataset with a large PTV.
Other techniques that address important motion (>1 cm in the craniocaudal direction) have been studied, such as breath-hold techniques or abdominal compression to decrease the tumor motion. Solutions exist to reduce the PTV margin. First, there is an active breath-hold technique using a spirometer-closed scissor valve at a predefined volume. Next, a gating system, such as the ExacTrac® for the Novalis Tx system (Brainlab AG, Feldkirchen, Germany), can synchronize the time when the external beam is switched on with the respiratory phase. Underberg et al. described the benefits of this technique with PTV reduced by 15% and mean volume of normal tissue irradiated reduced by 18% when the respiration-gated technique was used (90).
However, dynamic tracking using fiducial markers or tumor visualization represents the best strategy to decrease normal irradiated tissue volume and achieve superior tumor-dose coverage (91). CyberKnife® (Accuray, Inc., Sunnyvale, California) is one of the most commonly used commercial systems, and uses an x-ray imaging device to detect and continuously monitor the tumor position during treatment thanks to the six-axis accelerator (Figure 12.5). CyberKnife® XSight Lung can also track without a fiducial marker if the target can be seen on an x-ray device. Bahig et al. tested 113 targets and found that tumor diameter, volume, and density are predictive of the ability to track without fiducial markers (92). Finally, the CyberKnife system can use both external and fiducial markers to increase the accuracy of the tracking system or perform double tracking using vertebrae and fiducial markers.
FIGURE 12.4 Motion management using ITV delineation.
ITV, internal target volume.
195
FIGURE 12.5 Examples of tracking with CyberKnife® (Accuray): (A) using fiducial markers (Synchrony®) and (B) without fiducial markers (XSight Lung®).
Image courtesy of Accuray Incorporated-©2018 Accuray Incorporated. All Rights Reserved.
When fiducial markers are used, they are inserted 1 to 2 weeks before the treatment planning. The main complication encountered with fiducial markers is pneumothorax, but patients can also present with fiducial migration, pain, or hematoma. Van der Voort van Zyp et al. (93) found that although median marker displacement is small (1.3 mm), displacements greater than 5 mm occurred for 12% of the markers. Therefore, multiple markers are needed to compensate for those that present important displacement. Numerous solutions are in development to improve tumor marking, such as electromagnetic navigational bronchoscopy-guided fiducial marker placement or intrabronchial coils (94).
QUALITY OF LIFE AND TOXICITY
Toxicity
Toxicities from SBRT are low, but depend on the tumor size, tumor localization, and patient characteristics. Thus, the first studies of SBRT on central tumors reported some fatal complications (fistula, bronchial stricture, heart failure, pneumothorax, pneumomediastinum, and hemoptysis) or important toxicity (brachial plexopathy), but these complications have been reduced by adapting the dose and fractionation. Major tumor cavitation and suspicion of endobronchial involvement are considered risk factors for hemorrhage and hemoptysis.
Currently, the toxicities reported for lung SBRT are pneumonitis (≥G3, 4%–25%), fibrosis (12%–15%), chest pain (5%–25%), rib fracture (2%–3%), and fatigue. Grade 1 or 2 toxicities are reported in up to 100% of cases. Reports of grade 3 or 4 toxicities range between 2.7% and 27%, but late radiological changes are seen in 50% to 80% of patients (77). Interestingly, Alite et al. explored the impact of angiotensin-converting enzyme inhibitors (ACEi) on SBRT toxicity and found a lower rate of clinical pneumonitis for patients using ACEi (p = .04) (95). Some cases of organizing pneumonia, vertebral fracture, or pseudosclerodermatous panniculitis have been reported following lung SBRT.
UPMC Multicenter Quality of Life Study
The impact of SRS on the QOL in medically inoperable patients with NSCLC has not been fully evaluated. We reported preliminary results regarding the QOL after SRS in a prospective multicenter study, led by UPMC, that evaluated outcomes following SRS for stage I NSCLC in medically inoperable patients (96). The dose prescribed ranged from 48 to 60 Gy in three to four fractions. The general QOL was assessed by the SF-36 questionnaire (physical component score [PCS]/mental component score [MCS]; normal mean score 50). Dyspnea was evaluated with the validated University of California, San Diego (UCSD) Shortness of Breath Questionnaire. These were administered at baseline and after treatment (4–6 weeks; 3–6 months). There was early preservation of QOL after treatment with no significant changes in the PCS/MCS or dyspnea score after SRS. Further investigations with longer follow-up are required to evaluate the durability of 196this preservation in QOL following treatment with SRS. Videtic (97) reported no change in QOL even if the lung volume was significantly decreased after SBRT (mean 10%). There were also no significant pulmonary function changes after SBRT in that study.
FOLLOW-UP AFTER TREATMENT
After SBRT, follow-up with a CT scan at least every 6 months for 3 years is needed in accordance with the ESMO clinical guidelines in patients who may be candidates for salvage treatment. The scheduling of follow-up CT scans should be tailored for other patients. At UPMC, patients are followed routinely in the thoracic surgery and/or radiation oncology clinic every 4 to 6 months and undergo physical examination in addition to imaging. At UPMC, we routinely obtain a chest CT scan during follow-up and obtain a PET scan selectively in some patients.
During follow-up with CT scanning, only 20% of patients will present a complete response with a disappearance of the tumor, but nearly 90% of cases present CT density changes after 2 years (Figure 12.6) (98). It is important to recognize some high-risk radiological features in serial CT scans of local relapse: enlarging opacity, history of enlargement (sequential or late), craniocaudal growth, pleural effusion, condensation increasing, bronchiectasis disappearance, and margin modification. Peulen et al. (99) validated the ability of these features to diagnose local recurrence and saw a specificity and sensitivity of up to 100% in patients with four or more high-risk radiological features seen on CT scanning. At UPMC, we have used a modified response evaluation criteria in solid tumors (RECIST) system incorporating both CT scanning and PET scanning to evaluate response to treatment and tumor progression (8). Some authors have also explored radiomic features indicative of recurrence and progression, but little data have been published, and only high-risk validated clinical features are used in clinical practice (100).
FIGURE 12.6 Chest CT scan before SBRT (left panel) and after 2 years of follow-up (right panel).
SBRT, stereotactic body radiation therapy.
CONCLUSIONS
SBRT/SABR/SRS for lung tumors, including primary NSCLC, recurrent lung cancer, and pulmonary metastases, is already a reality. Encouraging outcomes have been reported for medically inoperable patients with early stage NSCLC or recurrent lung cancer, and in selected patients with oligometastatic lung disease. SBRT is considered to be a standard treatment for early stage NSCLC in medically inoperable patients. However, many questions need to be resolved, such as optimal patient selection, optimization of treatment, dose schema, and standards for reporting results. Studies are ongoing to further evaluate the role of SBRT in lung cancer treatment including prospective multicenter studies evaluating SRS/SBRT/SABR for stage I NSCLC, randomized trials comparing SBRT with sublobar resection in high-risk operable patients with stage I NSCLC, and trials of SBRT in patients with oligometastatic disease or recurrent disease.
SUMMARY OF DOSE CONSTRAINTS (TABLE 12.3)
TABLE 12.3 Dose Constraints for Lung Primary and Metastases Using SBRT
Unresected Target | 54 Gy (Three Fractions) 48 Gy (Four Fractions) 50 Gy (Five Fractions) |
Critical Structures | Suggested Dose Limits |
Chest wall | V30 ≤70 mL |
Ipsilateral lung | V20 ≤10%, V12.5 <15% |
Brachial plexus | BED3 <100 Gy, ≤24 Gy (three fractions) |
Aorta; main vessel | Dmax 45 Gy (three fractions) or 52.5 Gy (five fractions) |
Central bronchial tree | <30 Gy (three fractions); 15.6 Gy <4 mL |
Spinal cord (canal) | Dmax 18 Gy (three fractions) Dmax 20 Gy (five fractions) |
Stomach | Dmax 30 Gy (three fractions) Dmax 35 Gy (five fractions) |
Skin | ≤24 Gy in three fractions |
BED, biological effective dose; Dmax, maximum dose; SBRT, stereotactic body radiation therapy.
197REFERENCES
1. Song DY, Kavanagh BD, Benedict SH, et al. Stereotactic body radiation therapy: rationale, techniques, applications, and optimization. Oncology (Williston Park). 2004;18: 1419-1430. PubMed PMID: 15609470.
2. Lax I, Blomgren H, Näslund I, et al. Stereotactic radiotherapy of malignancies in the abdomen: methodological aspects. Acta Oncol. 1994;33:677-683. PubMed PMID: 7946448.
3. Blomgren H, Lax I, Näslund I, et al. Stereotactic high dose fraction radiation therapy of extracranial tumors using an accelerator: clinical experience of the first thirty-one patients. Acta Oncol. 1995;34(6):861-870. PubMed PMID: 7576756.
4. Whyte RI, Crownover R, Murphy MJ, et al. Stereotactic radiosurgery for lung tumors: preliminary report of a phase I trial. Ann Thorac Surg. 2003;75:1097-1101. PubMed PMID: 12683544.
5. Timmerman R, Papiez L, McGarry R, et al. Extracranial stereotactic radioablation: results of a phase I study in medically inoperable stage I non–small cell lung cancer. Chest. 2003;124:1946-1955. PubMed PMID: 14605072.
6. Timmerman R, McGarry R, Yiannoutsos C, et al. Excessive toxicity when treating central tumors in a phase II study of stereotactic body radiation therapy for medically inoperable early-stage lung cancer. J Clin Oncol. 2006;24:4833-4839. doi: 10.1200/JCO.2006.07.5937
7. Onishi H, Araki T, Shirato H, et al. Stereotactic hypofractionated high-dose irradiation for stage I nonsmall cell lung carcinoma: clinical outcomes in 245 subjects in a Japanese multiinstitutional study. Cancer. 2004;101:1623-1631. doi: 10.1002/cncr.20539
8. Pennathur A, Luketich JD, Heron DE, et al. Stereotactic radiosurgery for the treatment of lung neoplasm: experience in 100 consecutive patients. Ann Thorac Surg. 2009;88(5): 1594-1600. doi: 10.1016/j.athoracsur.2009.05.020
9. Siegel R, Naishadham D, Jemal A. Cancer statistics 2012. CA Cancer J Clin. 2012;62(1):10-29. doi: 10.3322/caac.20138
10. Stewart BW, Wild CP, eds. World Cancer Report 2014. Lyon, France: IARC Press; 2014:350-352.
11. Sibley GS, Jamieson TA, Marks LB, et al. Radiotherapy alone for medically inoperable stage I non-small-cell lung cancer: the Duke experience. Int J Radiat Oncol Biol Phys. 1998;40:149-154. PubMed PMID: 9422571.
12. Karam SD, Horne ZD, Hong RL, et al. Dose escalation with stereotactic body radiation therapy boost for locally advanced non small cell lung cancer. Radiat Oncol. 2013;8:179. doi: 10.1186/1748-717X-8-179
13. Hellman S, Weichselbaum RR. Oligometastases. J Clin Oncol. 1995;13:8-10. doi: 10.1200/JCO.1995.13.1.8
14. Pennathur A, Luketich JD, Heron DE, et al. Stereotactic radiosurgery/stereotactic body radiotherapy for recurrent lung neoplasm: an analysis of outcomes in 100 patients. Ann Thorac Surg. 2015;100:2019-2024. doi: 10.1016/j.athoracsur.2015.04.113
15. Shultz DB, Filippi AR, Thariat J, et al. Stereotactic ablative radiotherapy for pulmonary oligometastases and oligometastatic lung cancer. J Thorac Oncol. 2014;9(10):1426-1433. doi: 10.1097/JTO.0000000000000317
16. ICRU Report 91: Prescribing, recording, and reporting of stereotactic treatments with small photon beams. J ICRU. 2014;14(2):1-160. doi: 10.1093/jicru/ndx017
17. Chi A, Nguyen NP, Komaki R. The potential role of respiratory motion management and image guidance in the reduction of severe toxicities following stereotactic ablative radiation therapy for patients with centrally located early stage non-small cell lung cancer or lung metastases. Front Oncol. 2014;4:151. doi: 10.3389/fonc.2014.00151
18. Pennathur A, Luketich JD, Burton S, et al. Stereotactic radiosurgery for the treatment of lung neoplasm: initial experience. Ann Thorac Surg. 2007;83(5):1820-1824. doi: 10.1016/j.athoracsur.2006.11.077
19. Pennathur A, Luketich JD, Heron DE, et al. Stereotactic radiosurgery for the treatment of stage I non-small cell lung cancer in high-risk patients. J Thorac Cardiovasc Surg. 2009;137(3):597-604. doi: 10.1016/j.jtcvs.2008.06.046
20. Banki F, Luketich JD, Chen H, et al. Stereotactic radiosurgery for lung cancer. Minerva Chir. 2009;64(6):589-598. PubMed PMID: 20029356.
21. Christie NA, Pennathur A, Burton SA, et al. Stereotactic radiosurgery for early stage non-small cell lung cancer: rationale, patient selection, results, and complications. Semin Thorac Cardiovasc Surg. 2008;20(4):290-297. doi: 10.1053/j.semtcvs.2008.12.001
22. Raz DJ, Zell JA, Ou SH, et al. Natural history of stage I non-small cell lung cancer: implications for early detection. Chest. 2007;132(1):193-199. doi: 10.1378/chest.06-3096
23. Palma D, Visser O, Lagerwaard FJ, et al. Impact of introducing stereotactic lung radiotherapy for elderly patients with stage I non-small-cell lung cancer: a population-based time-trend analysis. J Clin Oncol. 2010;28:5153-5159. doi: 10.1200/JCO.2010.30.0731
24. Zimmermann FB, Geinitz H, Schill S, et al. Stereotactic hypofractionated radiation therapy for stage I non-small cell lung cancer. Lung Cancer. 2005;48(1):107-114. doi: 10.1016/j.lungcan.2004.10.015
25. Onishi H, Shirato H, Nagata Y, et al. Hypofractionated stereotactic radiotherapy (HypoFXSRT) for stage I non-small cell lung cancer: updated results of 257 patients in a Japanese multi-institutional study. J Thorax Oncol. 2007;2(suppl 3):S94-S100. doi: 10.1097/JTO.0b013e318074de34
26. Pennathur A, Loo BW, Heron DE, et al. Stereotactic radiosurgery for stage I NSCLC in medically inoperable patients: a prospective multicenter phase II study. American Society for Therapeutic Radiology and Oncology (ASTRO) 54th Annual Meeting, Boston, MA, 2012.
27. Timmerman R, Paulus R, Galvin J, et al. Stereotactic body radiation therapy for inoperable early stage lung cancer. JAMA. 2010;303(11):1070-1076. doi: 10.1001/jama.2010.261
28. Fakiris AJ, McGarry RC, Yiannoutsos CT, et al. Stereotactic body radiation therapy for early-stage non-small-cell lung carcinoma: four-year results of a prospective phase II study. Int J Radiat Oncol Biol Phys. 2009;75(3):677-682. doi: 10.1016/j.ijrobp.2008.11.042
29. Chang JY, Senan S, Paul MA, et al. Stereotactic ablative radiotherapy versus lobectomy for operable stage I non-small-cell lung cancer: a pooled analysis of two randomised trials. Lancet Oncol. 2015;16:630-637. doi: 10.1016/S1470-2045(15)70168-3
30. Meyers BF, Puri V, Broderick SR, et al. Lobectomy versus stereotactic body radiotherapy for stage I non-small cell lung cancer: post hoc analysis dressed up as level-1 evidence? J Thorac Cardiovasc Surg. 2015;150(3):468-471. doi: 10.1016/j.jtcvs.2015.06.086
31. Crabtree TD, Denlinger CE, Meyers BF, et al. Stereotactic body radiation therapy versus surgical resection for stage I non-small cell lung cancer. J Thorac Cardiovasc Surg. 2010;140:377-386. doi: 10.1016/j.jtcvs.2009.12.054
32. Palma DA, Nguyen TK, Kwan K, et al. Short report: interim safety results for a phase II trial measuring the integration of stereotactic ablative radiotherapy (SABR) plus surgery for early stage non-small cell lung cancer (MISSILE-NSCLC). Radiat Oncol. 2017;12(1):30. doi: 10.1186/s13014-017-0770-7
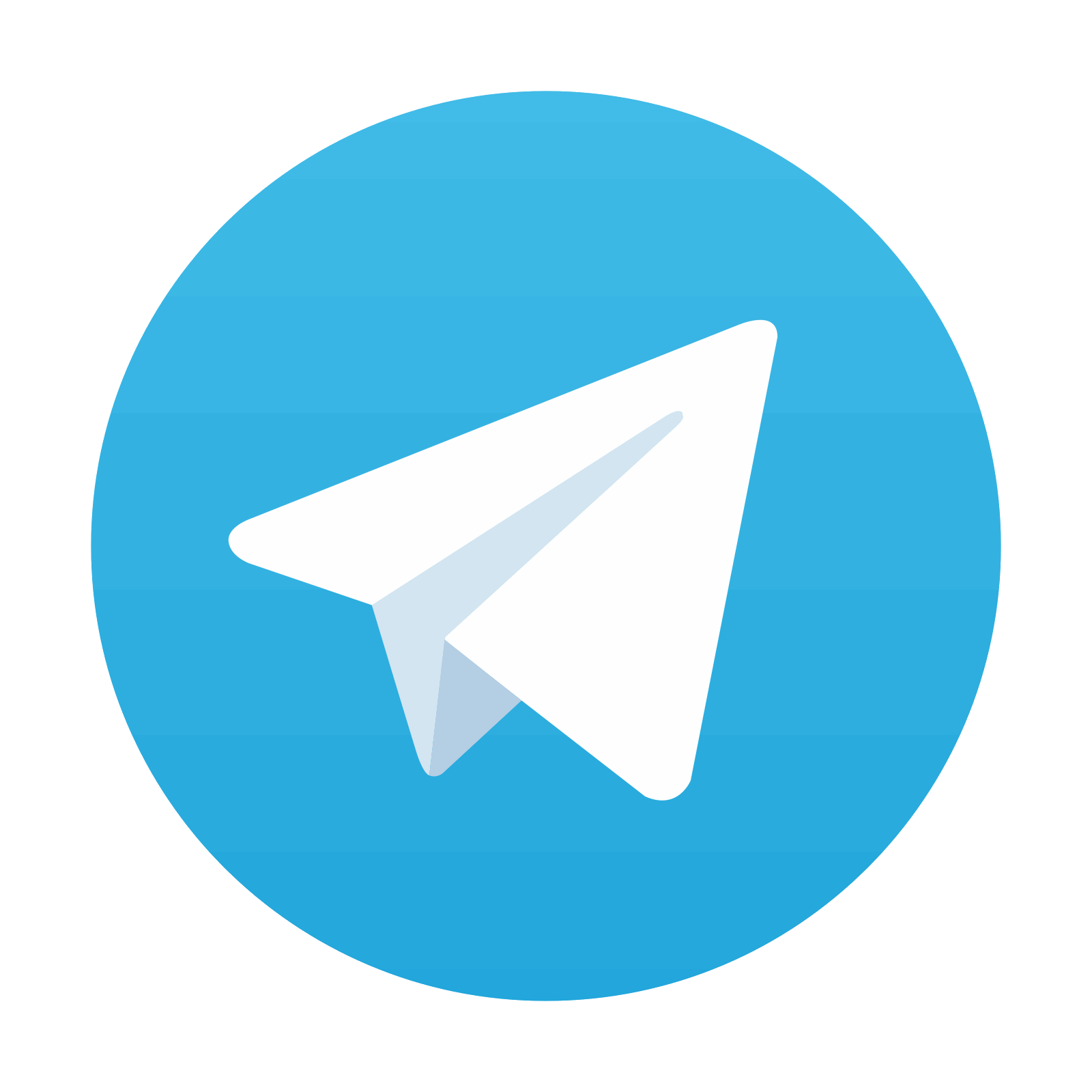
Stay updated, free articles. Join our Telegram channel

Full access? Get Clinical Tree
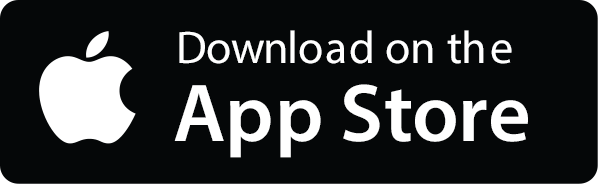
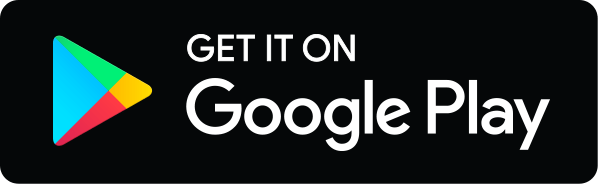