Fig. 1
156-channel fMEG device SARA II (SQUID Array for Reproductive Assessment, VSM MedTech Ltd., Port Coquitlam, Canada) installed at the fMEG Center in Tübingen (© University Hospital Tübingen)
3.1 Fetal Measurements
Before each fetal measurement, the head position of the fetus has to be determined. Therefore, an ultrasound is performed immediately before the measurement and fetal head position is marked on the maternal abdomen. After finding a comfortable position on the device, localization coils are attached as described above. During the entire measurement session, contact between the subject and the researcher is ensured through a camera and an intercom. Immediately after the measurement, a second ultrasound is performed to check for changes in the fetal position.
For auditory stimulation during a measurement, stimuli are produced by loudspeakers outside the shielded room and led through air-filled tubes to a balloon which is located directly above the maternal abdomen (Fig. 2a). For visual stimulation, light stimuli are produced by a panel of light emitting diodes (Fig. 2b).
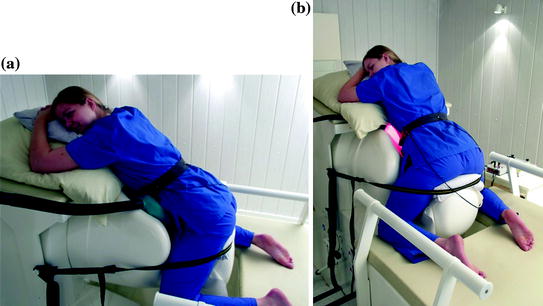
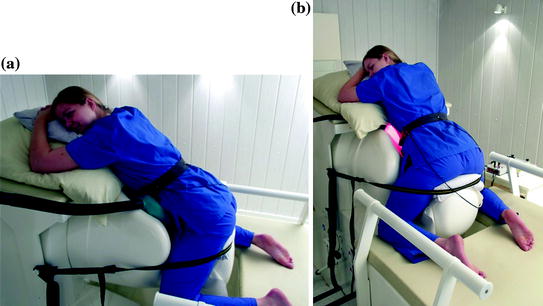
Fig. 2
Fetal measurement with (a) auditory and (b) visual stimulation. Tones are produced outside the shielded room and transmitted through air-filled tubes to a balloon located directly above the maternal abdomen. Light flashes are produced by a panel of light emitting diodes (© University Hospital Tübingen)
3.2 Neonatal Measurements
For neonatal measurements, a cradle is attached to the fMEG device, which ensures that the newborn is lying comfortably and safely during the measurement. Generally, measurements are performed while the newborn is sleeping or lying quietly.
For auditory stimulation, the newborn is lying on one side with its contralateral temporal lobe resting on the sensor array. Stimulation is produced outside the shielded room, conducted through air filled tubes and presented to the left ear using a headphone which is especially developed for neonatal measurements (Fig. 3). For visual stimulation, the newborn is lying on its back with its occipital lobe resting on the sensor array. The light pad is fixed at approximately 1 m above the neonatal head.
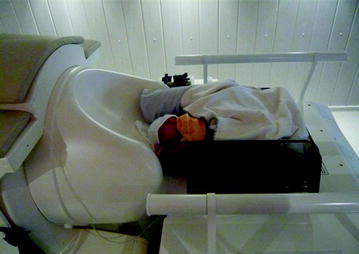
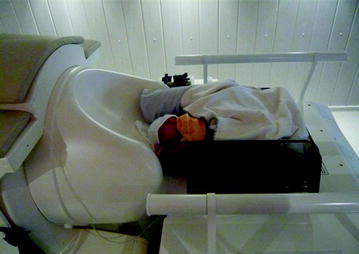
Fig. 3
Neonatal measurement with auditory stimulation. Tones are produced outside the shielded room and transmitted through air-filled tubes to small earphones especially designed for neonatal measurements (© University Hospital Tübingen)
4 State of the Art in Functional Fetal Brain Research Using fMEG
In the year 1985, the first fetal AERs were detected using a one-channel MEG device (Blum et al. 1985). Since then, the technology was improved and measurements with more channels were made possible. In the last decades, mainly auditory evoked responses (AERs) and visual evoked responses (VERs) were recorded and their change over gestational age was investigated (Holst et al. 2005; Eswaran et al., 2002a, b; Schleussner and Schneider 2004). Moreover, auditory change detection (e.g., change in frequencies) was evaluated (Draganova et al. 2005, 2007) and response decrement (i.e., habituation) after repetitive auditory and visual stimulation has been investigated (Sheridan et al. 2008; Matuz et al. 2012; Muenssinger et al. 2013).
4.1 State of the Art in Auditory fMEG Research
As described above, the first human auditory experiences can be expected at 20 weeks GA. In fMEG studies using pure tone stimulation, AERs were detected reliably at a GA of 28 weeks (Lengle et al. 2001; Schleussner and Schneider, 2004; Eswaran et al. 2002a). While response detection rates were highly variable, an AER detection rate of around 80 % could be reached in fetuses between 28 and 40 weeks GA (Schleussner and Schneider 2004; Holst et al. 2005) and 30 and 40 weeks GA (Eswaran et al. 2002a). Moreover, longitudinal studies evaluated the development of AER responses over GA. Therefore, fetuses between 27 and 40 weeks GA were included and measured at least twice with an interval of approximately 2 weeks between measurements (Holst et al. 2005). Results showed that the AER latencies decreased with increasing GA, indicating a gradual maturation of auditory processes and therefore an increase in the speed of auditory signal processing during the last trimester of pregnancy (Holst et al. 2005). These results are also in accordance with those of Schleussner and Schneider (2004), who showed decreasing latencies of the P2 pm and N2 pm components with increasing GA. These findings are first steps toward the understanding of healthy brain maturation in utero and might in the future be helpful in detecting deviant brain development. Moreover, in addition to pure sound detection, fetuses in the last trimester of pregnancy are also able to detect changes in sound frequencies (Draganova et al. 2005). To investigate this ability, an oddball paradigm was used. 500 Hz (88 %) tones were intermixed with 750 Hz (12 %) tones and mismatch negativity responses (MMN), which are an indicator for change detection (in this case a change in frequency), were evaluated. It could be shown that in 48 % of the fetal recordings, an MMN response was found. In a follow-up study, detection rates of MMN responses increased to 66 % in fetuses between the GA of 28 and 39 weeks and 89 % in newborns (Draganova et al. 2007). These results strongly indicate that the fetal brain in the last trimester of pregnancy is able to process auditory stimuli and detect changes in stimulus frequencies. This is an important prerequisite for language development and processing. Also concerning habituation, the most basic form of learning, an auditory fMEG study was performed (Muenssinger et al. 2013). Fetuses were measured using an auditory short-term habituation paradigm consisting of trains of tones including five 500 Hz tones, one 750 Hz tone (dishabituator) and another two 500 Hz tones each. After response sensitization resulting in a response increment between tones one and two, the expected response decrement for the four repetitively presented 500 Hz tones could be observed (Fig. 4).
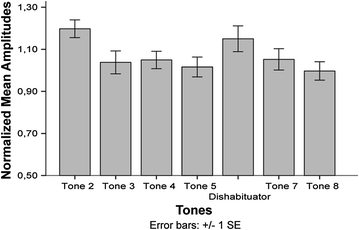
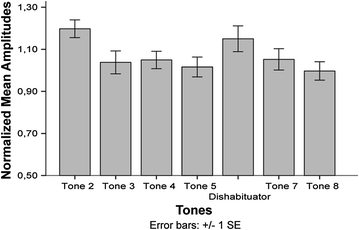
Fig. 4
Normalized fetal amplitudes to tones 2–8 of an auditory habituation paradigm. Mean and standard error are displayed. Figure with permission from Muenssinger et al. (2013)
This response decrement could either be due to sensory adaptation (fatigue) or to habituation. Therefore, not only dishabituation (response increment between last tone before and first tone after the dishabituator) but also stimulus specificity (response increment between last tone before dishabituator and dishabituator itself) were evaluated. Additionally, MMN responses between the last tone before the dishabituator (standard) and the dishabituator itself (deviant) have been investigated. Both stimulus specificity and the presence of MMN responses would be an indicator for habituation as reason for response decrement, because sensory fatigue would be stimulus independent. Significant stimulus specificity was found and MMN responses were detected in 50 % of the fetuses (Fig. 5). This indicates that already fetuses in the last trimester of pregnancy are able to show habituation, a basic form of learning.
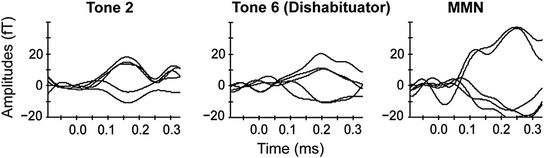
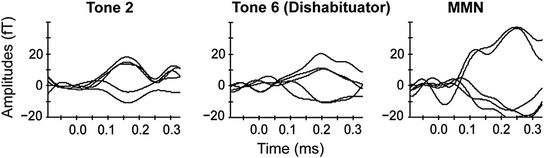
Fig. 5
Amplitude example of tone 2, tone 6 (dishabituator) and the MMN response of one fetus at the gestational age of 36 weeks. The five channels with the highest amplitudes are shown. Figure with permission from Muenssinger et al. (2013)
4.2 State of the Art in Visual fMEG Research
Similar to AERs, VERs have been detected in fetuses as early as in the 28th week GA. In their preliminary study, Eswaran et al. (2002b
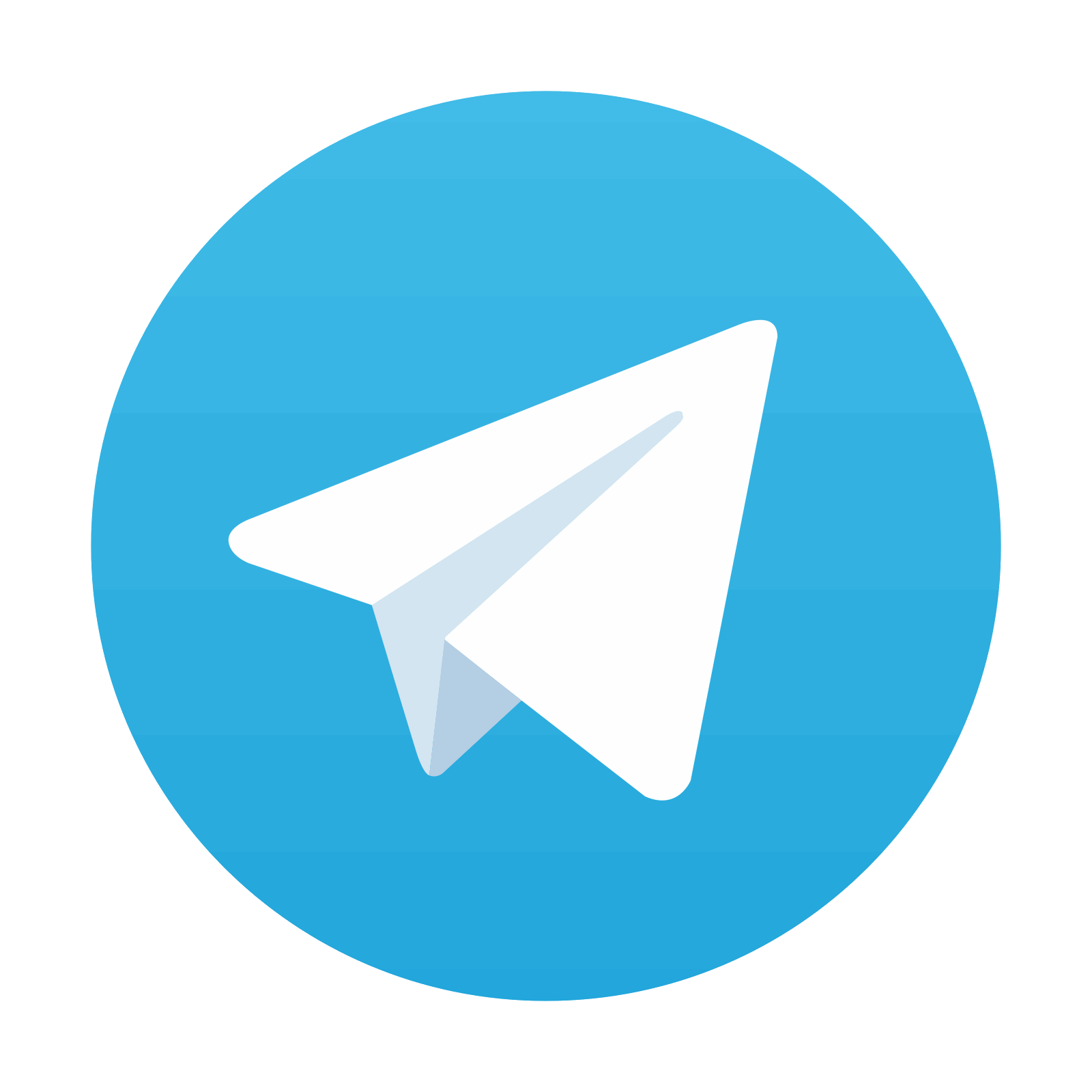
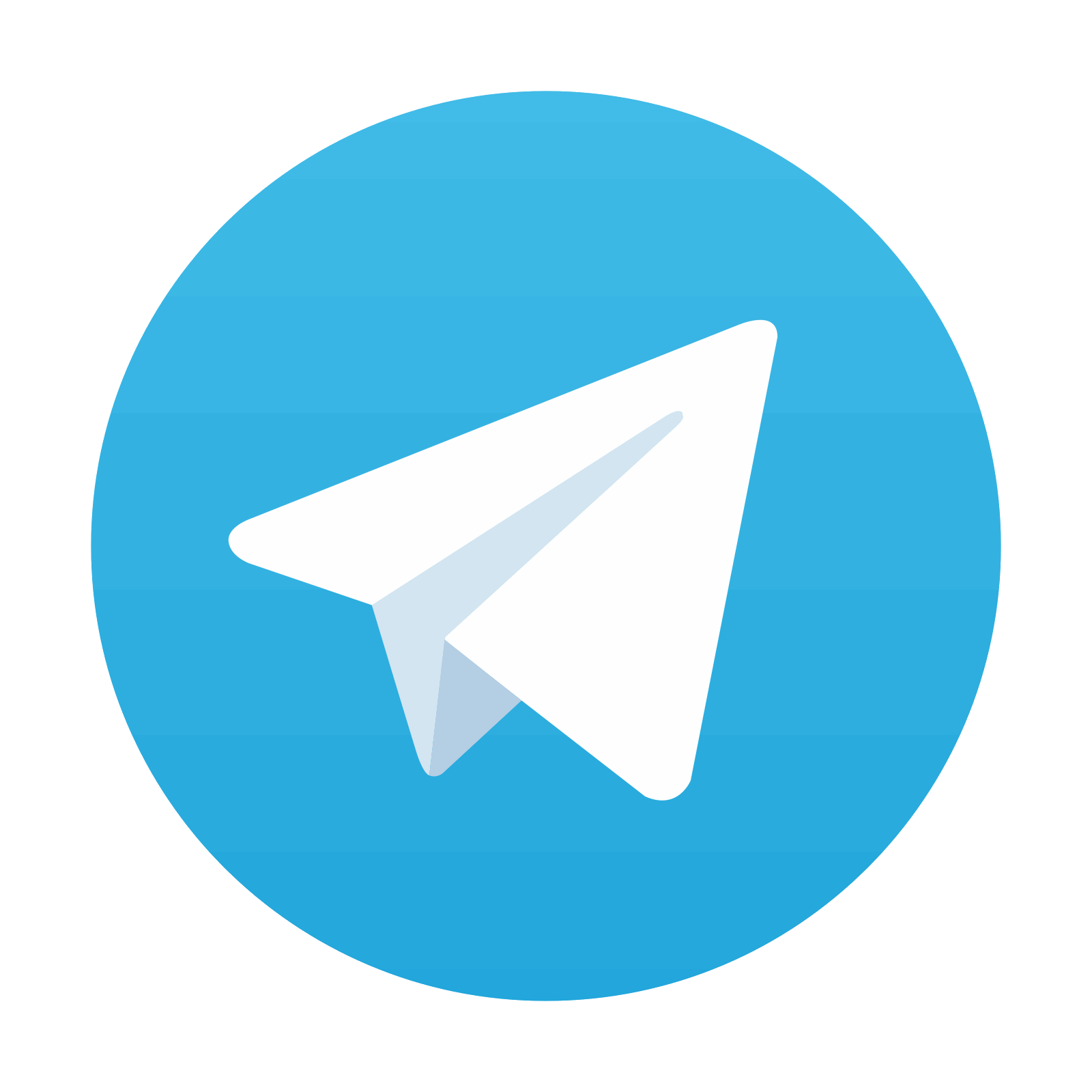
Stay updated, free articles. Join our Telegram channel

Full access? Get Clinical Tree
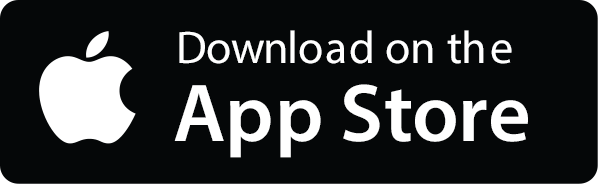
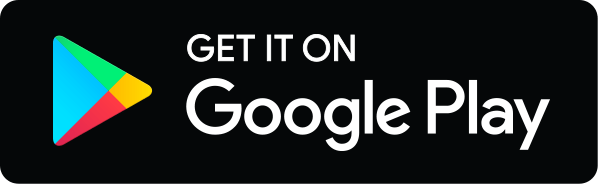