(1)
Center for Electron Microscopy and Microanalysis (CEMMA), University of Souther California, Los Angeles, CA, USA
Abstract
Microwave processors can provide a means of rapid processing and resin embedding for biological specimens that are to be sectioned and examined by transmission electron microscopy. This chapter describes a microwave-assisted protocol for processing, dehydrating and embedding biological material, taking them from living specimens to blocks embedded in sectionable resin in 4 h or less.
Key words
Transmission electron microscopyResin embeddingThin sectioningImmuno-cytochemistryRapid processingMicrowave1 Introduction
1.1 History
Rapid processing of tissues for histology offers important advantages for rapid diagnosis, convenience and efficiency. The earliest methods for decreasing processing times involved heating specimens [1, 2]. Introduction of the microwave processor into the laboratory offered a more controlled approach to heating tissues and cells. By penetrating the biological material, microwaves overcame the limitation of poor heat conduction and thus produced more even heating throughout the processed specimens. Introduced in 1970 for histological processing [3] microwave fixation produced satisfactory results with few artifacts in mouse and fresh human postmortem tissues [3]. The idea of heating tissues in a constant volume bath of liquid to stabilize the temperatures inside the microwave chamber was introduced in 1978 [4] when Login performed a study to compare the effects of heating tissues in different solutions. Heating tissues in saline or Zenker’s solution (a fixative used by histologists that contains mercuric chloride and acetic acid) produced better results than if tissues were heated in formalin [4]. Enzyme reactivity and immunoreactivity was preserved in microwave-fixed tissues [5], microwave-assisted heating of sections was discovered to be an efficient method for antigen retrieval [6], and immunolabeling times could be shortened using microwaves [7–9]. Microwave irradiation was also found to decrease decalcification rates of human temporal bones with no detectable adverse effects on ultrastructure or antigenicity [10–13]. The microwave processor thus became a routine tool in the histology laboratory for heating biological specimens and speeding up processing times [14–18].
Introduction of microwave technologies to the electron microscopy laboratory were initially restricted to methods involving specimen heating [5, 19]. Although the microwave-induced heating produced useable preserved specimens, the ultrastructural damage was not suitable for critical work [5, 19, 20]. Microwave-induced heating in the presence of aldehyde gradually took over as a viable approach to rapid fixation for electron microscopy [20–23]. Microwave processing under more careful temperature control was achieved using water loads in the chamber of the processor, or by shielding the tissues with cooling jackets containing circulating water [24, 25]. Careful evaluation of microwave-assisted processing of biological material began to reveal improved morphology when compared with conventional processing methods performed at ambient temperature [26–30]. Examination of microwave-processed bone revealed improved ultrastructure and antigen retention [12, 31].
The technical developments that allowed biological material to be exposed to microwaves without being heated [29], opened a controversy that is still not completely settled, that of whether there is a “microwave-only effect” independent of temperature. Early studies suggested that specimen heating caused by exposure to microwaves was responsible for the decrease in preparation times and improved ultrastructure [20, 32]. However, more recent studies using more carefully controlled conditions seem to indicate a role for microwaves in the absence of heat in the improvements of processing times and ultrastructure [29, 33]. Electromagnetic effects of microwaves on cellular membranes, supporting a microwave-only effect, have been demonstrated in Escherichia coli bacteria [34].
1.2 Contemporary Uses of Microwave Processors in Biomedical Research
Microwave processors have become essential tools in the histology laboratory and have been incorporated into routine protocols. Microwaves are used to assist in specimen fixation [16, 35, 36], paraffin embedding [37, 38], antigen retrieval [6, 39, 40], staining [15, 41, 42], immunolabeling [7, 9, 39, 43–47], and in situ hybridization [48, 49]. Microwave processors are also being used for rapid bone decalcification [10–13, 33] (see Fig. 1).
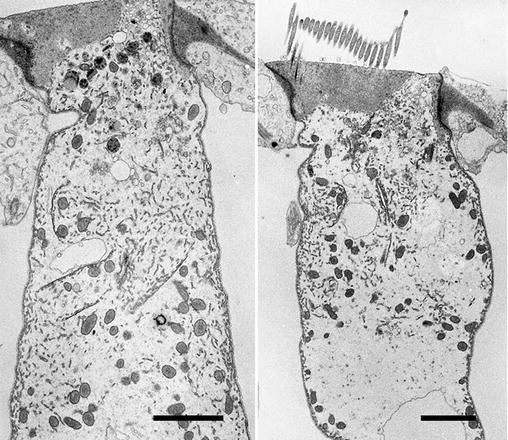
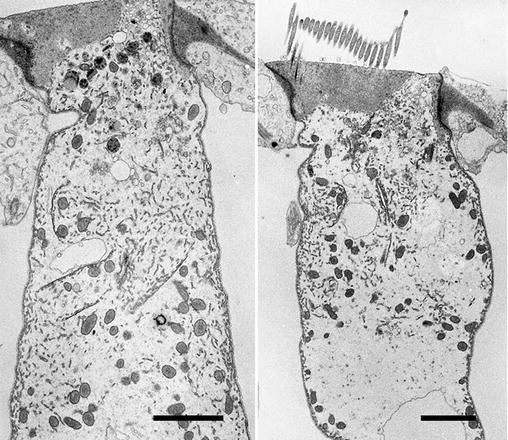
Fig. 1
Outer hair cells from the mouse inner ear. The whole inner ear, or cochlea was removed after perfusion fixation and then decalcified in 125 mM EDTA in a microwave processor. The whole cochlea was embedded in epoxy resin following the microwave-assisted protocol detailed in this chapter. The tissues were embedded in resin using heat polymerization and thin sections were examined in a TEM. Scale bars = 2 μm
Similar reports of microwave-assisted processing for electron microscopy indicate rapid processing times, improved specimen morphology and increased antigenicity of specimens being used for immunocytochemical experiments [21, 26, 28, 29, 31, 47, 50–54]. Microwave assisted resin polymerization has been used to embed thawed, thin cryosections [55], and diluted antibodies have been microwave irradiated to increase their labeling efficiency for EM immunocytochemistry [46]. As a result of these reports, and with the inclusion of microwave processing in the curricula of practical courses, electron microscopy laboratories are adapting the microwave processor for use in routine protocols.
1.3 A Routine Microwave-Assisted Processing Protocol for Electron Microscopy
The protocols described in this chapter have been extensively tested in different laboratories using different microwave processors and have been shown to yield reproducible results. The only variable we have discovered is with the Epon substitute available from different suppliers. The Spurr-Epon recipe we have provided will polymerize differently if Eponate 12 is not used. However, it appears that all Epon substitutes can be used, but the final result may require some adjustment to optimize the hardness of the resin. We recommend using complete kits from a single supplier, and not mixing ingredients from different suppliers. We also recommend testing all resin mixtures before using them to embed specimens.
The complete protocol can be applied as is, or, as is the case in my laboratory, parts of the protocol can be incorporated into existing long protocols. For example, we occasionally perform dehydration and resin infiltration using the microwave processor but perform the final resin polymerization at 60 °C in a regular oven. We also use rapid resin polymerization to re-embed polymerized specimens that need reorienting for sectioning. Examples of microwave assisted processing can be examined in Figs. 2, 3, and 4 in this chapter. All were processed into epoxy resin using microwave-assisted fixation, dehydration, and resin infiltration using the protocol outlined below and annotated in Table 1(a). All specimens were infiltrated using the epoxy resin formulation documented in Table 2(c). The specimens illustrated in Fig. 2 were polymerized in resin using the microwave protocol described in Subheading 3.6. Specimens illustrated in Figs. 3 and 4 were polymerized in resin using an overnight exposure to 60 °C.
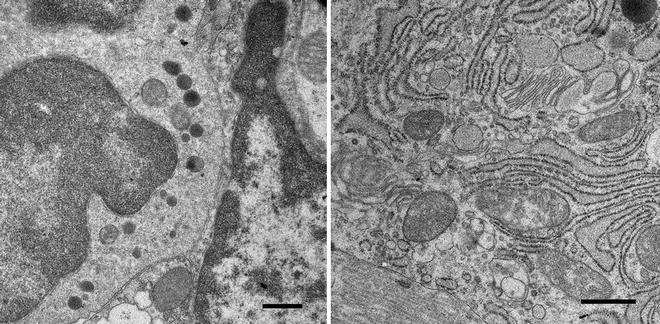
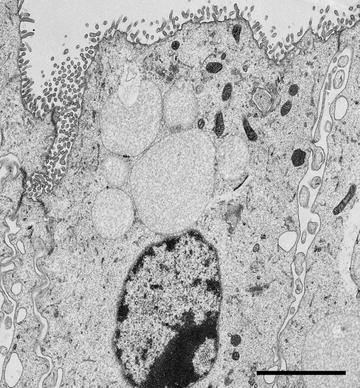
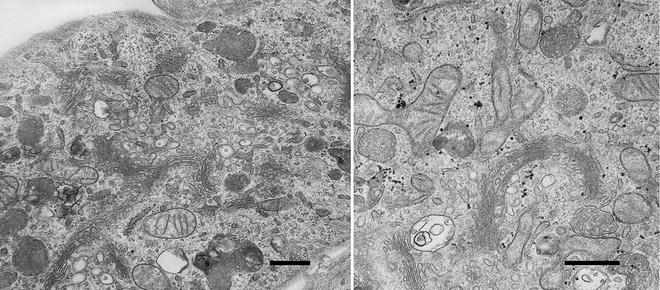
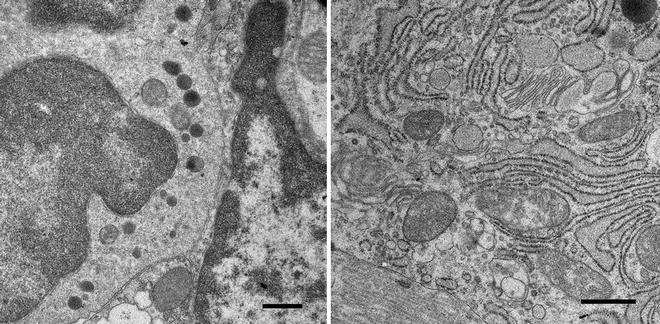
Fig. 2
Cells in the rat round window membrane, a membrane, three-cell layers thick, that separates the middle ear cavity from the inner ear, or cochlea, in mammals. The ability of drugs to pass through this membrane is important for delivery of therapeutics to the inner ear for treating hearing disorders. The membrane was removed from a freshly dissected cochlea and immersion fixed in 2.5 % glutaraldehyde buffered with 100 mM sodium cacodylate (pH 7.2). The tissue in fixative was irradiated in the microwave processor following the protocol described in this chapter. The membrane was cut into pieces and further processed following this protocol and finally embedded in resin, which was polymerized overnight at 60 °C. Thin sections were examined in a TEM. Scale bars = 500 nm
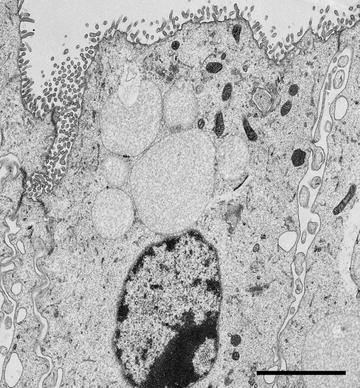
Fig. 3
A TEM image of a Hensen’s cell dissected from a mouse cochlea. A row of Hensen’s cells runs alongside the organ of Corti in the mammalian inner ear and assist in protecting the sensory cells of the auditory organ by secreting annexin A1in response to corticosteroid treatment [30]. A strip of cells was dissected from a fresh mouse cochlea and immersion fixed in 2.5 % glutaraldehyde buffered with 100 mM sodium cacodylate (pH 7.2). The cells were further processed using the microwave-assisted protocol described in this Chapter. Scale bar = 2 μm
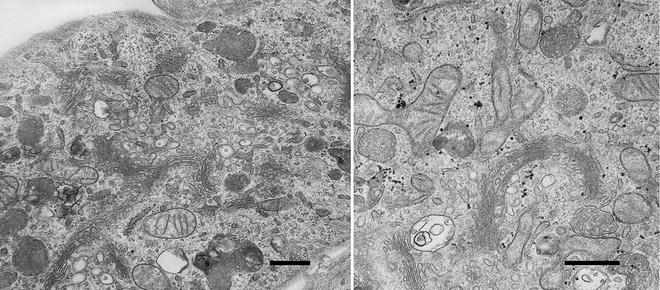
Fig. 4
Two images of cells used in a study examining the uptake and intracellular fate of nanoparticles with therapeutic applications [63, 64]. The cells were grown in vitro, incubated with nanoparticles and fixed by immersion in 2.5 % glutaraldehyde buffered with 100 mM sodium cacodylate (pH 7.2). The cells were then scraped, centrifuged into a pellet and processed using the protocol described in this chapter. The images show regions of the cell containing the Golgi complex. Mitochondria and membrane-bound organelles can also be observed. Scale bars = 500 nm
Table 1
Annotated microwave embedding protocols
Unless noted otherwise, all microwave irradiations are carried out with the processor operating at full power and containing a full water load | |||
(a) Embedding in Epoxy Resin (for specimens already chemically fixed). Use resin formulations A or B from Table 2 | |||
Time | Solution | Microwave | Temp limit |
10 s | Fixative | Yes | 30 °C |
20 s | Fixative | No | n/a |
10 s | Fixative | Yes | 30 °C |
5 min | Buffer | No | n/a |
40 s | 1 % osmium tetroxide | Yes | 37 °C |
30 s | Water | No | n/a |
40 s | 50 % Acetone | Yes | 37 °C |
40 s | 70 % Acetone | Yes | 37 °C |
40 s | 80 % Acetone | Yes | 37 °C |
40 s | 90 % Acetone | Yes | 37 °C |
40 s | 100 % Acetone | Yes | 37 °C |
40 s | 100 % Acetone | Yes | 37 °C |
15 min | 1:1 Acetone–resin | Yes | 45 °C |
15 min | 100 % resin | Yes | 45 °C |
15 min | 100 % resin | Yes | 45 °C |
10 min | Resin | Yes | 101 °C |
80 min | Resin | Yes (50 % Power) | 101 °C |
(b) Embedding in LR White Resin (for specimens already chemically fixed). Use LR White Resin (formulation C from Table 2) | |||
10 s | Fixative | Yes | 30 °C |
20 s | Fixative | No | n/a |
10 s | Fixative | Yes | 30 °C |
5 min | Buffer | No | n/a |
30 s | Water | No | n/a |
40 s | 50 % Ethanol | Yes | 37 °C |
40 s | 70 % Ethanol | Yes | 37 °C |
40 s | 90 % Ethanol | Yes | 37 °C |
40 s | 100 % Ethanol | Yes | 37 °C |
40 s | 100 % Ethanol | Yes | 37 °C |
15 min | 1:1 ethanol–resin | Yes | 45 °C |
15 min | 100 % resin | Yes | 45 °C |
15 min | 100 % resin | Yes | 45 °C |
45 min | Resin | Yes | 95 °C |
Table 2
Resin formulations for microwave-assisted polymerization
It is possible to prepare the following resin formulations without adding the catalyst. The resin mixtures can be stored in small aliquots at −20 °C for extended periods of time without the risk of the resin polymerizing (see Note 23). When the resin is ready to use warm an aliquot to room temperature and add catalyst dropwise from glass Pasteur pipettes. The numbers of drops to be added has been calibrated using glass Pasteur pipettes (1 drop = 0.01 g) | |
(a) SPURR-EPON Resin (from [51]) | |
To make approx 20 ml mix the following together in a tube: | |
ERL 4206 | 2.5 g |
DER 736 | 1.0 g |
NSA | 6.5 g |
Eponate 12 | 6.25 g |
DDSA | 3.25 g |
NMA | 3.0 g |
Store aliquots frozen in glass or polypropylene tubes | |
Before use, thaw and add: | |
DMAE | 6 drops |
DMP-30 | 8 drops |
(b) EPON Resin (from [50]) | |
Prepare and mix: | |
Solution A: Epon 812 (1.86 g) + DDSA (2.4 g) | |
Solution B: Epon 812 (2.48 g) + NMA (2.17 g) | |
Mix equal amounts of solutions A and B and add BDMA, 4 drops per ml | |
(c) Epon Resin (a recipe for making large batches) | |
Mix the following in a large glass jar: | |
NMA | 110 ml |
DDSA | 130 ml |
Eponate 12 | 230 ml |
Mix the ingredients well and pour the resin into small tubes in aliquots of 4–6 ml, and store frozen | |
To use, warm an aliquot of resin and add 4 drops of BDMA per ml. Use immediately and discard unused resin | |
Preparing and storing resin this way will ensure that resin ingredients are not wasted and all the tubes will have resin of the same consistency. The mixture can be tested before use by polymerizing one tube of resin. All subsequent tubes of resin will have the same qualities as this first tube, so long as the same amount of catalyst is added | |
(d) LR White | |
This resin is supplied premixed. Warm to room temperature before use |
2 Materials
2.1 Equipment
1.
2.
An ultramicrotome for thin sectioning resin-embedded biological specimens.
3.
A transmission electron microscope (TEM) operating at 80 kV for examining thin sections.
4.
A 60 °C oven is recommended for heat polymerization of resin.
2.2 Consumables
1.
Glass or plastic specimen vials.
2.
Eppendorf tubes (1 ml and 0.5 ml size).
3.
Two 500 ml glass beakers.
4.
Rubbermaid sandwich boxes.
5.
Plastic Pasteur pipettes.
6.
Ice buckets.
7.
BEEM capsules.
8.
Teflon capsule holders or Eppendorf tube racks.
9.
ParafilmM® (Parafilm)
2.3 Chemicals
1.
2.5 % glutaraldehyde in 100 mM sodium cacodylate buffer (pH 7.2).
2.
4 % formaldehyde in 100 mM HEPES buffer (pH 7.0).
3.
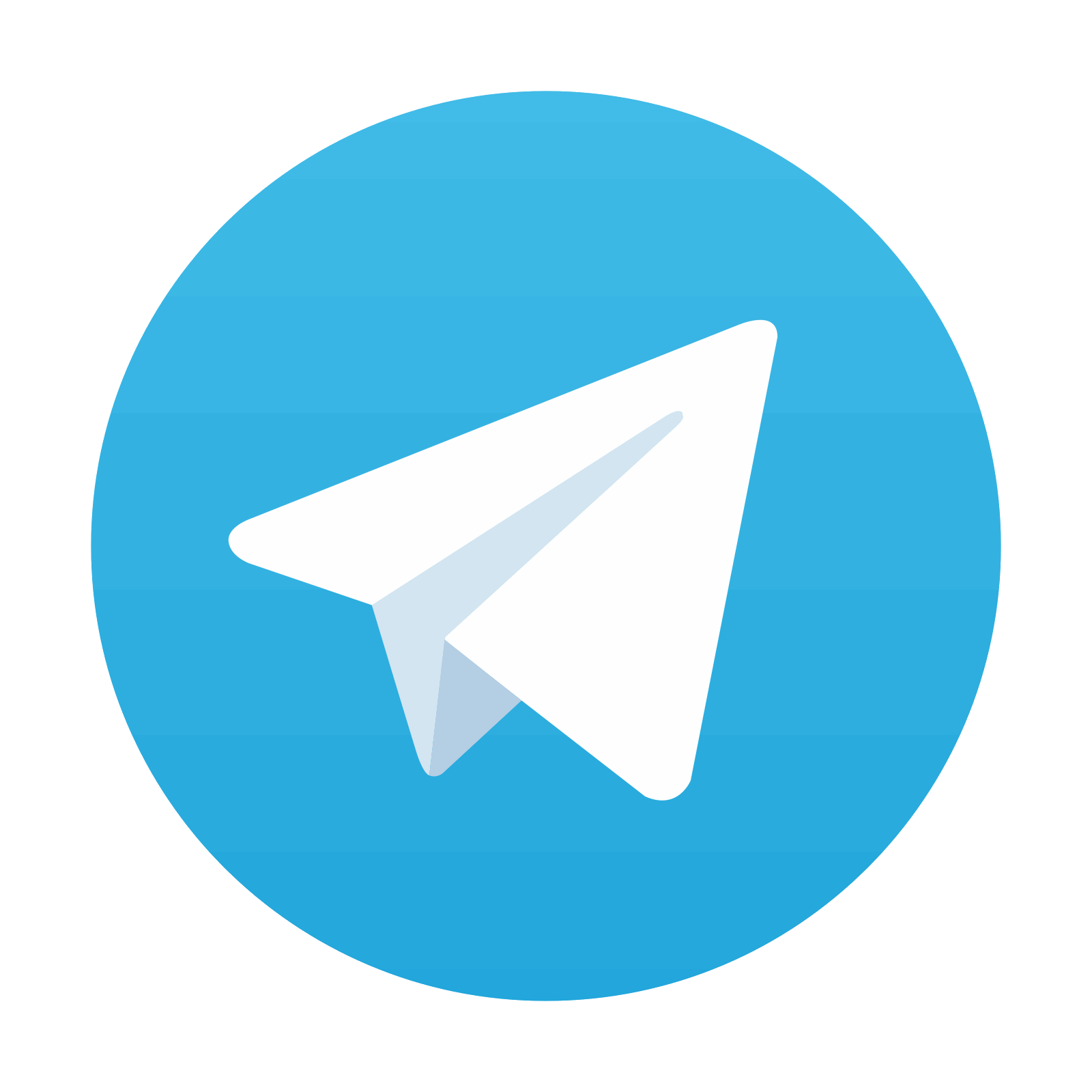
2 % aqueous osmium tetroxide.
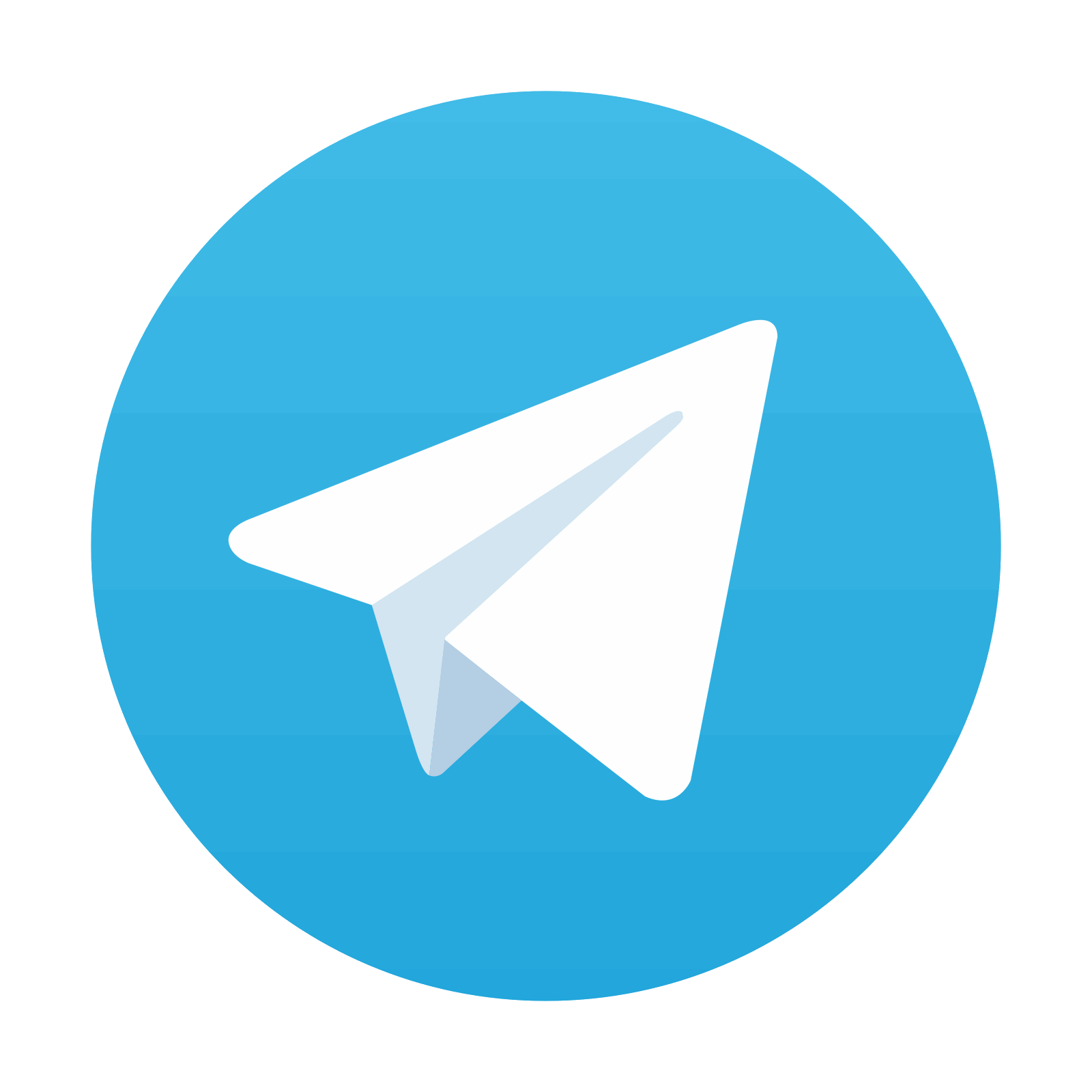
Stay updated, free articles. Join our Telegram channel

Full access? Get Clinical Tree
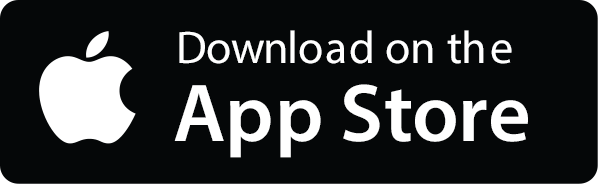
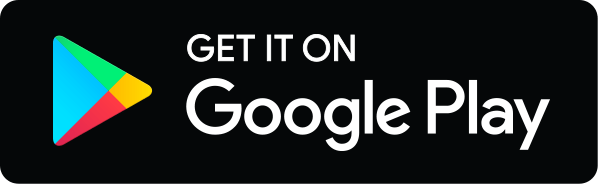
