Diagnostic cardiac catheterization procedures in children have been largely replaced by magnetic resonance (MR) imaging studies. However, when invasive catheterization is required, MR imaging has a significant role to play, when combined with invasive pressure measurements. Beyond the established reduction to the radiation dose involved, solely MR image-guided or MR image-assisted catheterization procedures can accurately address clinical questions, such as estimation of pulmonary vascular resistance and cardiac output response to stress, without needing to perform laborious measurements that are prone to errors. This article describes MR image-guided or MR image-assisted cardiac catheterization procedures for diagnosis and intervention in children.
Congenital heart disease occurs in 0.8% to 1% of children. Some of these patients will require interventional treatment in the form of cardiac catheterization or surgery, whereas others will require only medical therapy. Echocardiography is the investigation of choice for diagnosis and follow-up of these patients, whereas MR imaging is now increasingly used in most centers to assess complex congenital cases and to answer specific questions, which are not possible to address with echocardiography. The ability to obtain anatomic along with quantitative physiologic information, such as cardiac function and flows, in one examination has led to more detailed assessment and analysis of congenital heart defects. This continues to improve our understanding about congenital heart disease and its treatment options.
Cardiac catheterization procedures, routinely performed up to a few years ago to aid diagnosis, have now been mostly replaced by cardiac MR imaging scans. In parallel, a new form of hybrid catheterization has emerged that combines MR imaging with simultaneous pressure measurement in different cardiac chambers and vascular structures. The combination of radiographic (ie, x-ray) and MR imaging (XMR)-guided catheterizations can accurately address clinical questions, such as estimation of pulmonary vascular resistance (PVR) and cardiac output response to stress, without the limitation of hemodynamic assumptions during calculations. Also, for more accurate physiologic information, XMR catheterization can also offer detailed anatomic information of structures not well seen on echocardiography or MR imaging alone, such as small aortopulmonary collateral arteries, so that fluoroscopy and MR imaging can be combined in one procedure to avoid two general-anesthetic sessions in the same child and for the same purpose.
Beyond diagnosis, MR imaging currently expands its potential by guiding interventional procedures that may be performed in the MR imaging scanner in a similar fashion and with similar equipment to the ones used in the traditional catheterization suite. This article discusses in detail how MR imaging can be used for interventional diagnosis and treatment in young children with congenital heart disease.
MR imaging-guided diagnostic cardiac catheterizations
MR Imaging-Guided Diagnostic Catheterizations Combined with Radiographic Imaging
In the current era, diagnostic cardiac catheterizations have been greatly replaced either by MR imaging scans or by XMR catheterizations. The latter have been shown to entail lower radiation exposure doses when compared with controls, which is of particular importance in children with congenital heart disease who are usually subjected to various and repetitive procedures involving radiation. According to the UK National Radiation Protection Board, the mean risk of solid tumor development as a result of a single cardiac catheterization procedure is approximately 1 in 2500 in adults. This risk increases to 1 in 1000 in children if exposure occurs by the age of 5 years. Also, the proportion of the body that is irradiated increases as the size of the patient decreases, which, coupled with the increased radiosensitivity of the young and immature tissues, explains recent evidence showing that these children are at higher risk of developing cancer in later life.
A further benefit of XMR-guided catheterizations is the improved soft tissue characterization and visualization of cardiac structures and vessels, in contrast to radiographic-guided catheterizations, which rely on identification of landmarks and contrast angiography. XMR catheterizations also provide physiologic data, that are not possible to obtain during radiographic catheterization and that are essential for the assessment of complex patients. Such data involve cardiac output assessment, estimation of the pulmonary to systemic flow ratio (Qp/Qs), flows and maximum velocities across valves and in large vessels, pressure-volume loop relationships, and ventricular function. In addition, PVR and compliance can be accurately assessed using invasive pressure measurements and magnetic resonance (MR) flow data.
Equipment
XMR catheterizations take place in specifically designed catheterization laboratories with combined radiographic and MR imaging facilities ( Fig. 1 ). In the authors’ laboratory, a 1.5T MR imaging scanner (Achieva, Philips, Best, Netherlands) and a Philips BV Pulsera cardiac radiographic unit are used. There are in-room monitor and controls, which can swing across the bottom end and the top end of the magnet, and display MR images and hemodynamic pressure traces ( Fig. 2 ). The fluoroscopic area and MR field of an XMR laboratory can be separated either by doors or by a clearly defined line, demarcating the 5-gauss line of the magnet, beyond which it is possible for electronic devices, such as echocardiography machines and computer equipment, to be brought into the room ( Fig. 3 ). The tabletop design allows patients to be moved from one modality to the other in a very short time. Furthermore, the table position is stored within the system allowing image fusion between the MR imaging and radiographic system (ie, XMR) or even fusion with other imaging modalities (eg, echocardiography).
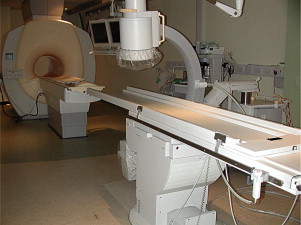
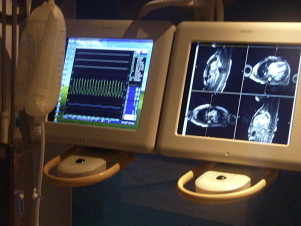
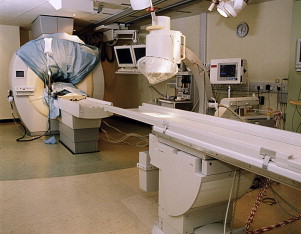
After the patient is placed on the table in the nonmagnetic area of the XMR room, the MR imaging coil and ECG leads are placed. MR-compatible patient monitoring and anesthetic equipment is used. For pressure monitoring, we use the commercially available hemodynamic monitoring system EP Tracer 102 (CardioTek B.V, Maastricht, the Netherlands). All of the anesthetic and monitoring tubing and lines are designed with extra length and are secured to the movable tabletop to ensure smooth patient transfer. The ECG and invasive pressure data are sent from the MR-compatible monitoring equipment via an optical network to a computer in the control room where the cardiac technician is stationed. The appropriate measurement and recording of the data is made in the usual way. The technician has access to monitors that show the appropriate radiographs or MR images of the procedure. Blood samples taken during the procedure are labeled in the room and passed to the technician in the control room. Monitoring of the cardiac rhythm during XMR catheterization is achieved with a vector electrocardiogram (VCG). The QRS detection algorithm automatically adjusts to the actual electrical axis of the patient’s heart and the specific multidimensional QRS waveform. This greatly improves the reliability of R wave detection to nearly 100%. A reliable R wave, with the P and T waves that are also always clearly seen with VCG, allows detection of nearly all arrhythmias. Unfortunately, there are no ECG systems that can reliably provide ST segment or T wave morphologic information. In the future, using signal-processing techniques may make it possible to improve ECG monitoring during MR imaging scanning.
Significant consideration has been given in the design, construction, and operation of the authors’ XMR facility with regard to safety, and a comprehensive safety protocol has been drawn up in our laboratory to minimize possible hazards ( Box 1 ).
Compulsory safety training of all MR imaging interventional staff
Specially designed clothes without pockets
Safety officer restricting entry to the main room during XMR intervention
Clear demarcation of ferromagnetic safe and unsafe areas within the room
MR-compatible anesthetic and monitoring equipment
Noise-proof headphone systems for all staff within the room
Scrub room shielded from x-rays and radiofrequencies
Positive-pressure air handling and filtration system
Tethering of all ferromagnetic equipment to the wall or floor
Safety checks whenever a patient is transferred between x-ray and MR areas to ensure that metallic instruments used for catheterization are not taken across to the MR end of the room
Written log of all safety infringements and regular review of safety procedures
XMR catheterization procedure
The children are prepared in a similar fashion to those undergoing routine cardiac catheterization. In the authors’ department, the patients are anesthetized and recovered in a dedicated area adjacent to the MR imaging scanning area. They are wheeled into the MR imaging room on MR-compatible trolleys and the procedure starts with cardiac catheterization in the nonmagnetic field area of the XMR laboratory. The facilities and equipment of the x-ray area are similar to the traditional catheterization laboratory. A non–MR-compatible angiographic pump is brought into the laboratory and used for detailed angiography, when needed. Standard ECG leads are placed along with a three-lead ECG attached to the anesthetic machine and the VCG, to use for cardiac monitoring during MR imaging scanning. The VCG electrodes are placed on the subcostal margin, outside the radiographic field of view, and the VCG is used for triggering MR imaging scans. An MR-compatible pulse oximeter and noninvasive blood pressure monitoring equipment are also attached. The exhaled anesthetic gases are monitored for end-tidal carbon dioxide and for the concentration of the volatile anesthetic agents. Flexible phased array radiofrequency (RF) coils are used. These coils are relatively x-ray lucent and do not need to be removed between radiographic and MR imaging.
After femoral venous and arterial access is obtained, a heparin bolus of 50 IU/kg is given with activated clotting time (ACT) monitoring. After right and/or left heart catheterization is completed under x-ray guidance, the patient is moved across to the MR imaging scanner on the sliding table after the standard ECG leads are removed and safety checks have been performed. These include an operating theater-style check of all metallic objects used under x-ray. Once the patient is inside the scanner, a second drape is placed and then lifted up and taped to the top of the magnet, which, in effect, provides sterile draping of the bore and sides of the magnet (see Fig. 3 ). MR-compatible catheters (Wedge Catheter, Arrow, Reading, PA, USA) placed during fluoroscopic-guided cardiac catheterization are left in situ, usually in the right ventricle or main pulmonary artery (MPA) for continuous hemodynamic pressure monitoring. Invasive arterial pressure is monitored through a femoral arterial cannula or sheath. Simultaneous MR imaging phase contrast or MR cine scans and pressure recording are performed. These give the physician a “snapshot” of the patient’s physiology at that particular time and reflect the conditions that they may have been subjected to (ie, nitric oxide administration during PVR assessment or dobutamine stress testing). In addition, a free-breathing, ECG-triggered, three-dimensional (3D) steady state free precession (SSFP) scan of the heart and great vessels and a 3D contrast-enhanced MR angiography are also performed to elucidate intracardiac and vascular anatomy.
The authors have performed more than 160 cardiac catheterizations for congenital heart disease in their XMR laboratory. Some of these have involved combined x-ray and MR-guided procedures, whereas others have been performed solely under MR guidance. Most of the catheterization procedures were performed for PVR measurement, although several were performed to define anatomy, perform XMR-guided interventions ( Fig. 4 ), or assess the cardiac stress response to dobutamine.
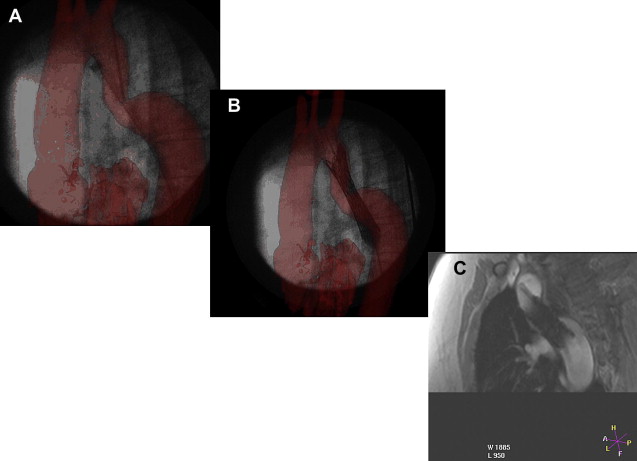
Assessment of pulmonary vascular resistance
PVR studies have been performed either with fluoroscopic or MR guidance. There is no patient age limit for the performance of these studies, but higher spatial resolution is required for phase contrast flow imaging in infants to ensure there are enough pixels covering the vessel of interest for accurate flow measurements. With the technique described above, the patient undergoes an MR imaging scan and phase contrast flow images are obtained in the MPA, right pulmonary artery (RPA), left pulmonary artery (LPA), and aorta with simultaneous pressure monitoring. For patients with univentricular heart palliation, flows will be assessed in the branch pulmonary arteries for PVR estimation, and in the two caval veins, the neoaorta (and native aorta, when of good size) for detailed assessment of the physiology and the flow distribution.
When the PVR at baseline is raised, 100% fraction of inspired oxygen and nitric oxide 20 ppm are given and the PVR is recalculated. It is important to obtain oxygen saturation and a blood gas at the beginning of the procedure and during different stages of the protocol, as dictated by the patient’s clinical condition and end-tidal carbon dioxide. This is to ensure a steady anesthetic state and normocarbia, which is vital for accurate PVR measurement because the latter can swing and be inadvertently elevated if the carbon dioxide levels are allowed to rise. The authors have found moderate to good agreement between the Fick method and the MR method of deriving PVR at baseline conditions. However, in the presence of nitric oxide, which is used to assess pulmonary vasoreactivity, there was less agreement between the two methods. There was not only worsening in agreement but also a large bias when PVR was measured in the presence of 100% oxygen and nitric oxide. We believe that this is the result of errors in the Fick method rather than the XMR method, which has important implications for patient management, particularly of young children. To this end, we suggest that PVR measurement in children is performed with the MR method wherever possible.
Dobutamine stress study for assessment of cardiac output response
Dobutamine stress study for assessment of the cardiac output response is regularly required in young infants and children, such as children with Alagille syndrome and pulmonary artery stenosis or, more rarely, in children with univentricular heart circulation. MR imaging is the ideal tool for the evaluation of cardiac function and cardiac output. When combined with dobutamine stress it allows the measurement of right and left ventricular function, cardiac output, and cardiac output in response to stress and systemic vasodilation.
Adding to a full anatomic assessment, MR imaging cardiac catheterization combined with dobutamine stress enables evaluation of the right ventricle (RV) pressure compared with systemic, the RV systolic and diastolic function in response to stress, the gradient across the pulmonary valve or branch pulmonary arteries and the pulmonary artery pressure, and PVR. If the PVR is elevated, pulmonary vasodilators can be administered and the patient’s PVR and hemodynamics are reassessed. Selective angiography can also be performed to address clinical questions, such as pulmonary arteriovenous malformations or abnormal collateral vessels that are not clearly visualized on MR imaging.
The authors’ MR imaging with dobutamine stress scan protocol is described as follows: We always ensure that the patient is not intravascularly dry because this may affect the cardiac output response to stress. After femoral venous and arterial access is obtained, a heparin bolus of 30 to 50 IU/kg is given with ACT monitoring. Right heart catheterization is performed either with fluoroscopic or MR imaging guidance. Pressure measurements are obtained in the femoral artery, inferior vena cava, right atrium, right ventricle, MPA, LPA (proximal and distal), RPA (proximal and distal), RPA and LPA wedge, or left atrium if an atrial communication is present. An MR imaging compatible catheter (wedge catheter) is left in the RV and an MR imaging scan is performed. This includes a four-chamber view, RV and LV outflow tract views in two planes, Gad MRA, 3D-volume SSFP scan, short axis stack (for LV and RV functional assessment), phase contrast flow images in the aorta and pulmonary artery (for assessment of cardiac output and shunt), and differential branch pulmonary artery flows. The PVR is obtained from a pulmonary artery flow measurement combined with direct pressure measurement, as described above. After baseline data are obtained, dobutamine is infused at 10 μg/kg/min and repeat short axis stack and phase contrast flows in the aorta and pulmonary artery are obtained. The infusion is then increased to 20 μg/kg/min with repeat short axis stack and phase contrast flows in the aorta and pulmonary artery. We aim for a heart rate increase of more than 50% from baseline. After all physiologic measurements have been obtained, the dobutamine infusion is discontinued and the patient is woken up and extubated. Children are observed on the ward for a few hours after anesthetic and are usually discharged home the same day.
Value of dobutamine stress studies in patients before liver transplantation
The value of dobutamine stress studies in patients with Alagille syndrome and branch pulmonary artery stenosis has been demonstrated. It was concluded that patients with a maximal increase in cardiac output of less than 40% and/or a right ventricular to aortic pressure ratio of equal or greater than 0.5 should be considered at higher risk and were associated with less favorable outcome following liver transplantation. This study was not performed in an XMR setting, but in the cardiac catheterization laboratory, using a flow-directed thermodilution catheter, and cardiac output calculations were performed on a dedicated computer (Vigalence, Baxter, Newbury, Berkshire, UK). In the current era, MR imaging would easily obtain the above calculations, with assessment of the ventricular function and volumes, as well as flows across the great vessels.
Solely MR Imaging-Guided Diagnostic Cardiac Catheterizations
MR imaging-guided catheterizations have been diagnostic in nature (in humans) and interventional (in animals). Visualization has been aided by passive and active visualization techniques and catheter tracking. Passive tracking technique is commonly based on visualization of susceptibility artifacts or signal voids caused by the interventional device under MR imaging, whereas active catheter tracking and visualization uses an electrical connection to the MR imaging scanner, and localization or tracking of the device requires the device itself, along with any additional hardware or software that comes with it. Typically, the device is equipped with a coil or an antenna that functions in either receive-only mode or transmit–receive mode.
Active guidewires that have been used for MR imaging-guide interventions in animals are tracked or visualized by employing miniature RF coils or loopless antenna each connected to the scanner using a long metallic wire. This long wire can heat up during certain MR imaging sequences due to resonating RF waves. Despite modifications to reduce the risk of heating with active devices, under certain conditions heating at the tip can occur up to 70°C with obvious associated risks. New strategies for RF-safe active devices have been proposed, including optical transmission and use of transformers to shorten the length of the conducting wire ( Figs. 5 and 6 ). However, no clinically safe active guidewires have been developed so far. Semiactive catheters use tuned fiducial markers that produce increased MR signal locally without wires connecting to the scanner. There are, however, issues with miniaturization and the ability of firmly securing these markers to catheters.
