The development of the spinal canal and its contents is highly complex and involves multiple programmed anatomic and functional developmental and maturational processes. Correct and detailed knowledge about spinal malformations is essential to understand and recognize these lesions early (preferably prenatally) to counsel parents during pregnancy, to plan possible intrauterine treatments, and to make decisions about the mode of delivery and the immediate postnatal treatment. This article discusses the imaging findings of the most frequently encountered neonatal spinal malformations and correlates these findings with the relevant embryologic processes. The presented classification is based on a correlation of clinical, neuroradiologic, and embryologic data.
The development of the spinal canal and its contents is highly complex and involves multiple programmed anatomic and functional developmental and maturational processes. These processes are tightly linked to each other and interact with each other at multiple anatomic levels simultaneously. The association of an open (non–skin covered) lumbar myelomeningocele and a Chiari II malformation is a well-known example of this multilevel interaction. In addition, malformations of the spinal canal and cord may be an isolated process involving only the neuroaxis or may be part of a complex syndrome or malformation (eg, cloacal malformation). Finally, the malformed spinal canal and cord may be secondarily injured because of prenatal, perinatal, and postnatal complications (eg, long-standing exposure of the neural tissue to the amniotic fluid, mechanical injury during delivery, or postnatal infection).
Correct and detailed knowledge about spinal malformations is essential to understand and recognize these lesions early (preferably prenatally) to counsel the parents during pregnancy, to plan possible intrauterine treatments, and to make decisions about the mode of delivery and the immediate postnatal treatment. The impact on quality of life varies significantly depending on the kind and extent of malformation. Correct classification of the identified malformation is a sine qua non. Frequently, spinal malformations are summarized as spinal dysraphism. Dysraphism is defined as an incomplete closure of a raphe or a defective fusion. Many of the malformations indeed belong to this category; however, a variety of spinal malformations may be observed that do not directly result from an incomplete closure of the neural tube during development. As two examples, early splitting of the notochord is linked to diastematomyelia (DMM), and persistence of the notochordal process may result in neurenteric fistula. Consequently, next to a detailed knowledge about the various malformations, a basic knowledge about embryology is essential.
This article discusses the imaging findings of the most frequently encountered neonatal spinal malformations and correlates these findings with the relevant embryologic processes. The presented classification is based on a correlation of clinical, neuroradiologic, and embryologic data.
Embryology
The normal development of the spinal canal and its contents relies on four principal processes: (1) gastrulation with development of the notochord; (2) primary neurulation with ganglion development; (3) segmentation with appearance of the somites; and (4) secondary neurulation (caudal cell mass). These processes occur in a sequential order with partial overlap. Based on these processes, malformations are classified as (1) disorders of primary neurulation, (2) disorders of secondary neurulation, or (3) anomalies of notochordal development.
During the first 10 to 14 days the fetus consists of two distinct cell layers: the ectoderm and endoderm ( Fig. 1 ). Around the 14th day of life the process of gastrulation (Weeks 2–3) starts. Ectodermal cells glide between the endoderm and ectoderm along the primitive streak, which is localized along the craniocaudal axis of the dorsum of the embryonic disk ( Fig. 2 ). During this phase the embryo transforms into a trilayered organism. Most of the cells migrate laterally between the endoderm and ectoderm to form the mesoderm. While the primitive streak is developing, it thickens at its cephalic end to form a structure called the Henson node (see Fig. 2 ). A portion of the invaginating cells remains in the midline and migrates along the craniocaudal axis of the primitive streak to form the notochordal process (see Fig. 2 ). After resorption of the floor of the notochordal process the resulting prochordal plate transforms into the definitive notochord (at 20 days) ( Fig. 3 ). The definitive notochord defines the primitive axis and skeleton of the embryo and is eventually replaced by the vertebral column. It extends throughout the entire embryo and reaches as far as the level of the future midbrain, where it ends in the region of the future dorsum sella. Most importantly, the notochord induces the transformation of the overlying ectoderm into neuroectoderm with formation of the neural plate (see Fig. 3 ). The notochord secretes a protein called “sonic hedgehog,” which plays a critical role in signaling the development of motoneurons. This induction of the neural plate signals the beginning of the primary neurulation (Weeks 3–4). Between Days 18 and 20 the neural plate transforms into a neural groove, which starts to close into a neural tube at Day 21 of gestation ( Fig. 4 ). While the neural tube is closing, the neuroectoderm progressively detaches from the adjacent surface ectoderm and “dives” into the space between ectoderm and endoderm ( Figs. 5 and 6 ). The adjacent surface ectoderm closes dorsally to the neural tube (see Fig. 6 ). At the cephalic and caudal end of the neural tube the anterior and posterior neuropore close at Day 25 of gestation. Simultaneously, cells at the border of the neuroectoderm and ectoderm detach to form the neural crests (see Fig. 6 ). The neural crests subsequently fragments and give rise to the primordial of the ganglia, which again give rise to the sensory innervations. The corresponding level of the neural tube and later spinal cord furnishes the motor innervations. A somite plate develops on each side of the neural tube, which also becomes segmentated (somite) ( Fig. 7 ). At the end of the 5th week of gestation, 42 pairs of somites are noted (see Fig. 7 ). The somites develop a central cavity; the internal side gives rise to the sclerotome, which again migrates toward the notochord and becomes the vertebral primordial. Cells include fibroblasts, chondroblasts, and osteoblasts. The part of the somite that remains in place becomes the dermomyotomes. The dermomyotomes are subsequently divided into dermatomes and myotomes. The myotomes give rise to the vertebral muscles. The caudal half of each sclerotome joins the cephalic part of the subjacent sclerotome resulting in the intersegmental origin of the vertebral bodies ( Fig. 8 ). The lowest part of the cranial half of each sclerotome forms the intervertebral disk. The notochord regresses at the level of the vertebral bodies but persists at the level of the intervertebral disks and becomes the nucleus pulposus (see Fig. 8 ). The paraxial musculature derived from the somites remains segmental, allowing the musculature to bridge from one vertebral body to the next. This gives maximal flexibility and mobility of the vertebral column. The spinal nerves remain segmental and consequently leave the spinal canal between the intervertebral foramina.
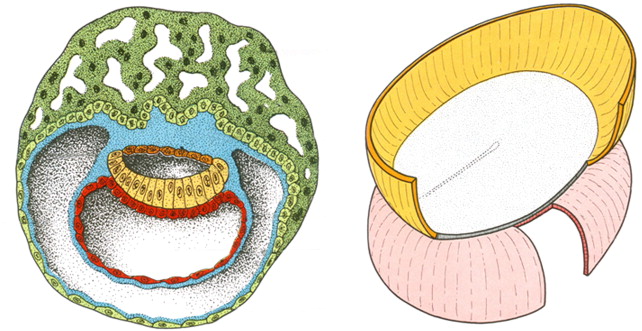
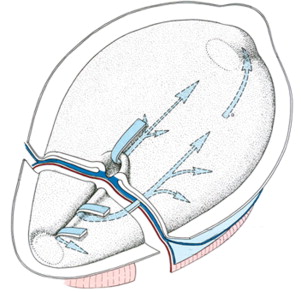
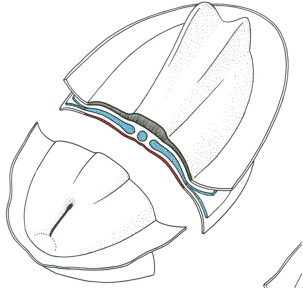
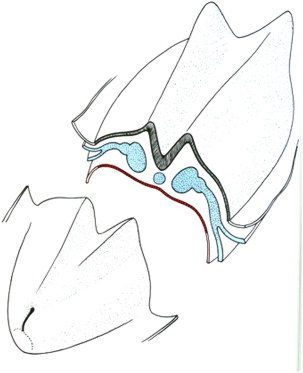
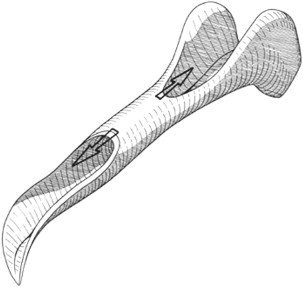
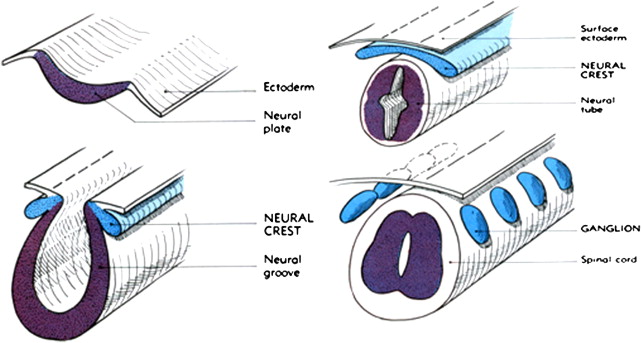
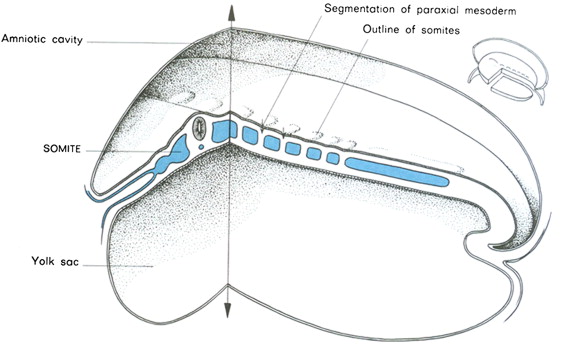
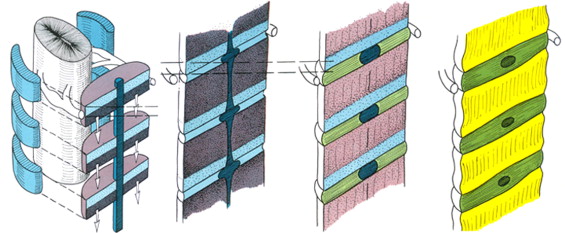
Next to the primary neurulation, which is responsible for the development of the brain and upper 90% of the spinal cord, the process of secondary neurulation (Weeks 5–6) forms the lower 10% of the spinal cord and filum terminale. Secondary neurulation starts after completion of the primary neurulation and proceeds until Day 48 of gestation. Inferior to the posterior neuropore a mass of cells derived from the caudal end of the primitive streak groups together and forms the distal spinal cord by a process of canalization, retrogressive differentiation, and finally fusion with the primary neural tube.
Disorders of primary neurulation
From a clinical and embryologic perspective the disorders of primary neurulation can be divided into (1) open spinal dysraphia, (2) dorsal dermal sinus, and (3) closed spinal dysraphia. In the open spinal dysraphia neural tissue is exposed to the skin with leakage of cerebrospinal fluid (CSF), whereas in the closed spinal dysraphia the malformed neural tube is covered by mesodermal (subcutaneous fat) and ectodermal (skin) elements. In these malformations no neural tissue is freely exposed and no leakage of CSF is present. In the case of a dorsal dermal sinus, a narrow canal persists between the neural tube and the overlying skin. Frequently, a small dimple is noted with intermittent leakage of drops of CSF, especially while the neonate is crying. The term “spina bifida occulta” is still en vogue but should be limited to the presence of a defective or incomplete fusion of the posterior osseous elements of the vertebral bodies. In these cases the neurulation is completed, the spinal cord is unremarkable, and the skin is covered. No associated central nervous system malformations are present. These “schisis” of the posterior vertebral arch are a frequent nonsignificant finding, predominantly involving the lumbar spine. All malformations of primary neurulation are characterized by cutaneous stigmata (birthmarks) with various degrees of skin discoloration or a hairy tuft.
Open (Non–Skin Covered) Spinal Dysraphia
These malformations have the most significant impact on the quality of life for the affected child. They are classified as myelocele (MC) and myelomeningocele (MMC). Both malformations result from a disturbance of the primary neurulation. An incomplete or segmental defective closure of the neural tube results in a neural placode that failed to detach from the adjacent surface ectoderm. Consequently, a flattened midline neural placode is either in level with the cutaneous surface (myelocele) or is pushed above or dorsal to the adjacent skin by the CSF anteriorly to the neural placode (myelomeningocele) ( Fig. 9 ). Adjacent bone, muscle, and skin are also deficient in various degrees of severity. These malformations most frequently occur at the lumbar level; however, the thoracic or cervical spinal cord may also be involved. MMCs are more frequently encountered than MCs. Because the neural placode is directly exposed to the air direct surgical repair is indicated to prevent further damage or secondary inflammation of the malformed spinal cord. The neural placode itself is believed to be less functional because of multiple complex primary and secondary processes including the failure of closure itself with resulting deranged neuroarchitecture but also because of chronic injury resulting from the long-lasting exposure of the neural tissue to the amniotic fluid. In addition, all children (100%) with an open spinal dysraphia have an associated Chiari II malformation. It is believed that the chronic leakage of CSF at the level of the neural placode during the intrauterine development of the fetal brain, in particular the rhombencephalic vesicle, results in an incomplete or defective expansion of the rhombencephalic vesicle, which in turn prevents normal growth of the skull base and posterior fossa. The postnatally observed anatomic features of a Chiari II malformations can at least partially be explained by a too small posterior fossa with resultant upward and downward herniation of cerebellar and brainstem structures. In several centers around the world, based on this hypothesis open spinal dyraphias are closed as early as possible during intrauterine life with the goal to limit the severity of Chiari II malformation, in particular the degree of hydrocephalus. Identification of a MC or MMC is rather straightforward on prenatal and postnatal MR imaging (see Fig. 9 ). A midline neural placode is seen at the top of the MC or MMC either in level with the adjacent surface ectoderm or protruding above the level of the surface ectoderm. Nerve roots appear along the anterior surface of the neural placode and depending on the degree of neural placode protrusion appear “stretched” in their course toward the neural foramina. In MMC malformations various degrees of meningeal structures are encountered herniating next to the neural placode. The spinal canal is usually widened with hypoplastic lateral and dorsal musculoskeletal derivates. Infrequently, the neural placode may protrude asymmetrically outside of the malformed spinal canal. In addition, various degrees of hydromyelia may be encountered in the intact spinal cord superior to the MC or MMC. Within the cranial vault the typical stigmata of a Chiari II malformation are encountered. All these findings should nowadays be detected by prenatal ultrasound examinations and if necessary confirmed by prenatal fetal MR imaging.
Closed (Skin Covered) Spinal Dysraphism
Skin covered or closed spinal dysraphisms include lipomyeloceles (LMC) and lipomyelomeningoceles (LMMC). These malformations have a significantly better clinical and neurologic prognosis because the neural tissue is covered and protected by skin and subcutaneous tissue. They are believed to result from a premature disjunction of the neural tube from the adjacent surface ectoderm before the neural tube is completely closed. Consequently, mesenchymal elements of the adjacent mesoderm have access to the inner surface of the not yet completely closed neural tube. For reasons not completely understood, the interaction of mesenchymal elements with the inner lining of the neural tube induces an excess production of fat. Depending on the amount and extension of the fat, various size lipomas occur that may be located exclusively intradural or may extend into the subcutaneous region. Because the overlying skin is closed and no CSF leakage occurs, no associated Chiari II malformation is encountered and the neural placode is less injured because of chronic exposure to the amniotic fluid during intrauterine life. Consequently, the neurologic function is preserved at a higher functional level compared with the open, non–skin covered spinal dysraphisms. Most children can walk but may suffer from bladder and bowel dysfunction.
Similar to the open spinal dysraphisms, the closed malformations are classified depending on the amount of tissue that is protruding outside of the spinal canal. If the neural tissue is seen within the level of the spinal canal the malformation is classified as LMC; if the neural tissue is pushed outside of the level of the spinal canal it is classified as LMMC ( Figs. 10 and 11 ). The size of the lipoma can vary significantly and may pose a large cosmetic issue for the child, especially if the child grows older. Surgical reduction of the lipoma is frequently performed later during life.
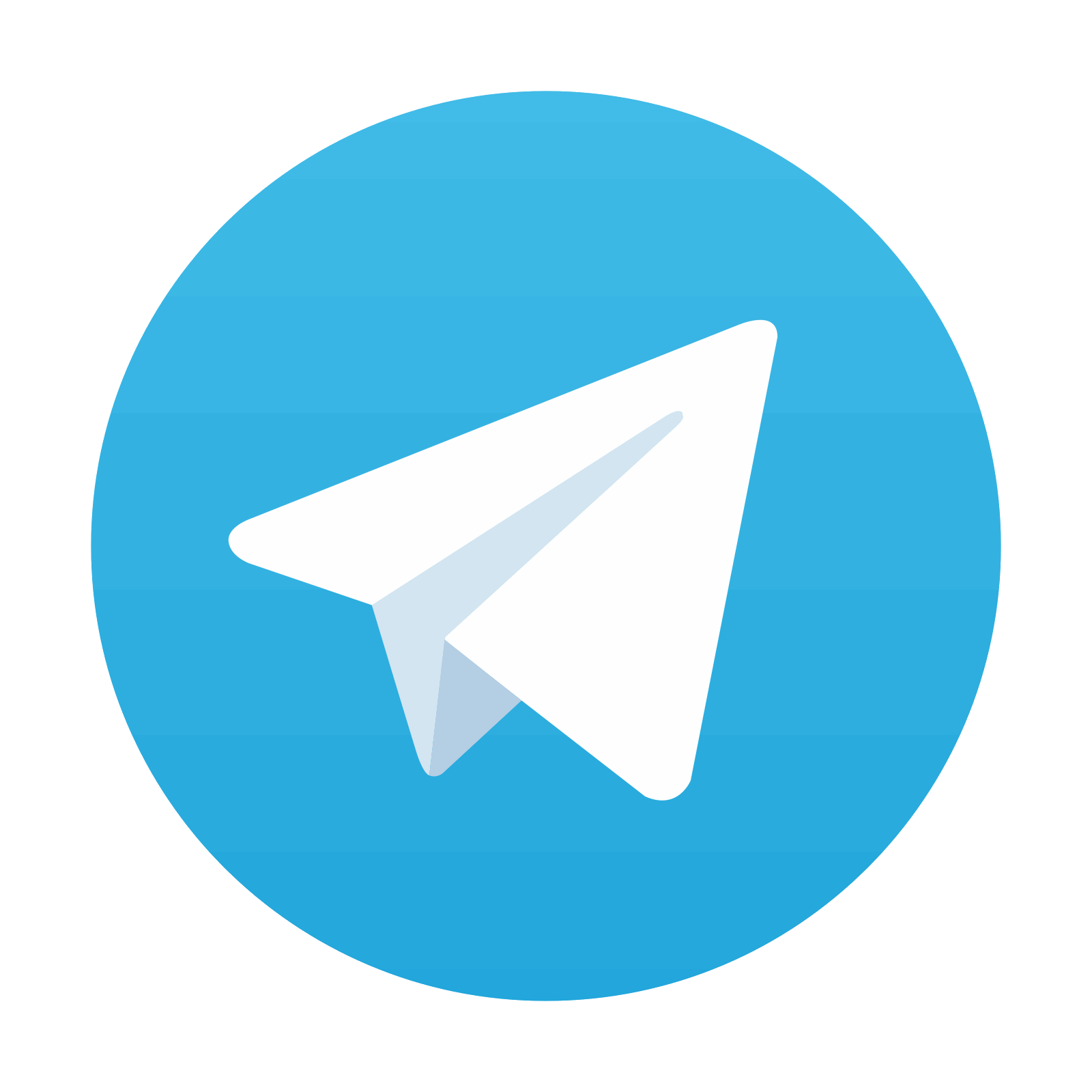
Stay updated, free articles. Join our Telegram channel

Full access? Get Clinical Tree
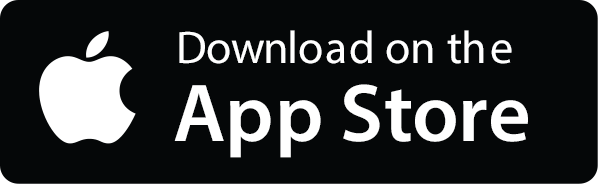
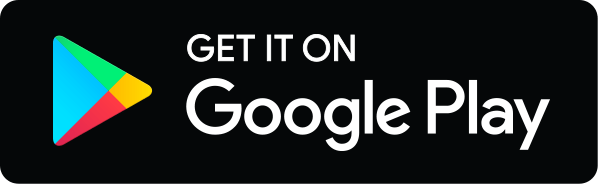
